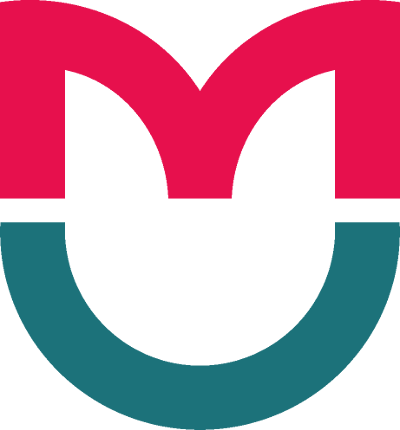
Статья размещена в открытом доступе и распространяется на условиях лицензии Creative Commons Attribution (CC BY).
ОБЗОР
Альтернативы антибиотикам: литические ферменты бактериофагов и фаговая терапия
Научно-исследовательский институт физико-химической биологии им. А. Н. Белозерского,Московский государственный университет им. М. В. Ломоносова, Москва, Россия
Для корреспонденции: Назаров Павел Александрович
ул. Наримановская, д. 22, к. 3, кв 294, г. Москва, 117997; moc.liamg@apvorazan
Финансирование: работа выполнена при поддержке Российского научного фонда (грант 14-50-00029).
Благодарности: автор благодарит сотрудников лаборатории биофизики мембран, отдела биоэнергетики НИИ ФХБ им. А. Н. Белозекрского МГУ, лаборатории молекулярной биоинженерии ИБХ им. М. М. Шемякина и Ю. А. Овчинникова РАН и лаборатории генетики бактериофагов НИИ вакцин и сывороток им. И. И. Мечникова за обсуждения некоторых аспектов использования бактериофагов, фаголизинов и антибактериальной фотодинамической терапии.
- Назаров П. А. Человечество может выиграть войну против бактерий. Коммерсант Наука. 2017; (5): 20–2.
- World Health Organization. Global action plan on antimicrobial resistance. Geneva, Switzerland: WHO Document Production Services; 2015. 21 p. Available from: http://www.who.int/antimicrobial-resistance/publications/global-action-plan/en/.
- Liu YY, Wang Y, Walsh TR, Yi LX, Zhang R, Spencer J et al. Emergence of plasmid-mediated colistin resistance mechanism MCR-1 in animals and human beings in China: a microbiological and molecular biological study. Lancet Infect Dis. 2016 Feb; 16 (2): 161–8. DOI: 10.1016/S1473-3099(15)00424-7.
- Pader V, Hakim S, Painter KL, Wigneshweraraj S, Clarke TB, Edwards AM. Staphylococcus aureus inactivates daptomycin by releasing membrane phospholipids. Nat Microbiol. 2016 Oct 24; 2: 16194. DOI: 10.1038/nmicrobiol.2016.194.
- Chen L, Todd R, Kiehlbauch J, Walters M, Kallen A. Notes from the Field: Pan-Resistant New Delhi Metallo-Beta-Lactamase-Producing Klebsiella pneumoniae — Washoe County, Nevada, 2016. MMWR Morb Mortal Wkly Rep. 2017 Jan 13; 66 (1): 33. DOI: 10.15585/mmwr.mm6601a7.
- World Health Organization. Antibacterial agents in clinical development. Geneva, Switzerland: WHO Document Production Services; 2017. 48 p. Availble from: http://apps.who.int/iris/bitstream/10665/258965/1/WHO-EMP-IAU-2017.11-eng.pdf?ua=1.
- Kohanski MA, Dwyer DJ, Collins JJ. How antibiotics kill bacteria: from targets to networks. Nat Rev Microbiol. 2010 Jun; 8 (6): 423–35. DOI: 10.1038/nrmicro2333.
- Baltz RH. Antimicrobials from Actinomycetes: Back to the Future. Microbe. 2007; 2 (3): 125–31.
- Lewis K. Platforms for antibiotic discovery. Nat Rev Drug Discov. 2013 May; 12 (5): 371–87. DOI: 10.1038/nrd3975.
- Ling LL, Schneider T, Peoples AJ, Spoering AL, Engels I, Conlon BP et al. A new antibiotic kills pathogens without detectable resistance. Nature. 2015 Jan 22; 517 (7535): 455–9. DOI: 10.1038/nature14098.
- Dibrov P, Dibrov E, Maddaford TG, Kenneth M, Nelson J, Resch C et al. Development of a novel rationally designed antibiotic to inhibit a nontraditional bacterial target. Can J Physiol Pharmacol. 2017 May; 95 (5): 595–603. DOI: 10.1193/cjpp-2016-0505.
- Hards K, Cook GM. Targeting bacterial energetics to produce new antimicrobials. Drug Resistance Updates. 2018; 36: 1–12.
- Jones D. Tuberculosis success. Nat Rev Drug Discov. 2013; 12 (3): 175–6.
- Khailova LS, Nazarov PA, Sumbatyan NV, Korshunova GA, Rokitskaya TI, Dedukhova VI et al. Uncoupling and Toxic Action of Alkyltriphenylphosphonium Cations on Mitochondria and the Bacterium Bacillus subtilis as a Function of Alkyl Chain Length. Biochemistry (Mosc). 2015 Dec; 80 (12): 1589–97. DOI: 10.1134/S000629791512007X.
- Nazarov PA, Osterman IA, Tokarchuk AV, Karakozova MV, Korshunova GA, Lyamzaev KG et al. Mitochondria-targeted antioxidants as highly effective antibiotics. Sci Rep. 2017 may 3; 7 (1): 1394. DOI: 10.1038/s41598-017-00802-8.
- Czaplewski L, Bax R, Clokie M, Dawson M, Fairhead H, Fischetti VA et al. Alternatives to antibiotics-a pipeline portfolio review. Lancet Infect Dis. 2016 Feb; 16 (2): 239–51. DOI: 10.1016/S1473-3099(15)00466-1.
- Ladhani SN, Campbell H, Parikh SR, Saliba V, Borrow R, Ramsay M. The introduction of the meningococcal B (MenB) vaccine (Bexsero®) into the national infant immunisation programme--New challenges for public health. J Infect. 2015 Dec; 71 (6): 611–4. DOI: 10.1016/j.jinf.2015.09.035.
- DiGiandomenico A, Sellman BR. Antibacterial monoclonal antibodies: the next generation? Curr Opin Microbiol. 2015 Oct; 27: 78–85. DOI: 10.1016/j.mib.2015.07.014.
- Babcock GJ, Broering TJ, Hernandez HJ, Mandell RB, Donahue K, Boatright N et al. Human monoclonal antibodies directed against toxins A and B prevent Clostridium difficile-induced mortality in hamsters. Infect Immun. 2006 Nov; 74 (11): 6339–47. DOI: 10.1128/IAI.00982-06.
- Foletti D, Strop P, Shaughnessy L, Hasa-Moreno A, Casas MG, Russell M et al. Mechanism of action and in vivo efficacy of a human-derived antibody against Staphylococcus aureus alpha-hemolysin. J Mol Biol 2013 May 27; 425 (10): 1641–54. DOI: 10.1016/j.jmb.2013.02.008.
- Oganesyan V, Peng L, Damschroder MM, Cheng L, Sadowska A, Tkaczyk C et al. Mechanisms of neutralization of a human anti-alpha toxin antibody. J Biol Chem. 2014 Oct 24; 289 (43): 29874–80. DOI: 10.10174/jbc.M114.601328.
- Lowy I, Molrine DC, Leav BA, Blair BM, Baxter R, Gerding DN et al. Treatment with monoclonal antibodies against Clostridium difficile toxins. N Engl J Med. 2010 Jan 21; 362 (3): 197–205. DOI: 10.1056/NEJMoa0907635.
- Szijártó V, Lukasiewicz J, Gozdziewicz TK, Magyarics Z, Nagy E, Nagy G. Diagnostic potential of monoclonal antibodies specific to the unique O-antigen of multidrug-resistant epidemic Escherichia coli clone ST131-O25b:H4. Clin Vaccine Immunol. 2014 Jul; 21 (7): 930–9. DOI: 10.1128/CVI.00685-13.
- Kelly-Quintos C, Cavacini LA, Posner MR, Goldmann D, Pier GB. Characterization of the opsonic and protective activity against Staphylococcus aureus of fully human monoclonal antibodies specific for the bacterial surface polysaccharide poly-Nacetylglucosamine. Infect Immun. 2006 May; 74 (5): 2742–50. DOI: 10.1128/IAI.74.5.2742-2750.2006.
- Hazenbos WL, Kajihara KK, Vandlen R, Morisaki JH, Lehar SM, Kwakkenbos MJ et al. Novel staphylococcal glycosyltransferases SdgA and SdgB mediate immunogenicity and protection of virulence-associated cell wall proteins. PLoS Pathog. 2013; 9 (10): e1003653. DOI: 10.1371/journal.ppat.1003653.
- Palliyil S, Downham C, Broadbent I, Charlton K, Porter AJ. High-sensitivity monoclonal antibodies specific for homoserine lactones protect mice from lethal Pseudomonas aeruginosa infections. Appl Environ Microbiol. 2014 Jan; 80 (2): 462–9. DOI: 10.1128/AEM.02912-13.
- Palliyil S. Generation of High-Sensitivity Monoclonal Antibodies Specific for Homoserine Lactones. Methods Mol Biol. 2018; 1673: 325–52. DOI: 10.1007/978-1-4939-7309-5_25.
- Varshney AK, Wang X, MacIntyre J, Zollner RS, Kelleher K, Kovalenko OV et al: Humanized staphylococcal enterotoxin B (SEB)-specific monoclonal antibodies protect from SEB intoxication and Staphylococcus aureus infections alone or as adjunctive therapy with vancomycin. J Infect Dis. 2014 Sep 15; 210 (6): 973–81. DOI: 10.1093/infdis/jiu198.
- Hilliard JJ, Datta V, Tkaczyk C, Hamilton M, Sadowska A, Jones-Nelson O et al. Anti- alpha toxin monoclonal antibody and antibiotic combination therapyimproves disease outcome and accelerates healing in a Staphylococcus aureus dermonecrosis model. Antimicrob Agents Chemother. 2015 Jan; 59 (1): 299–309. DOI: 10.1128/AAC.03918-14.
- Zou L, Liu M, Wang Y, Lu J, Pang Y. Determination of in vitro synergy between linezolid and other antimicrobial agents against Mycobacterium tuberculosis isolates. Tuberculosis (Edinb). 2015 Dec; 95 (6): 839–42. DOI: 10.1016/j.tube.2015.07.003.
- Berditsch M, Jäger T, Strempel N, Schwartz T, Overhage J, Ulrich AS. Synergistic effect of membrane-active peptides polymyxin B and gramicidin S on multidrug-resistant strains and biofilms of Pseudomonas aeruginosa. Antimicrob Agents Chemother. 2015 Sep; 59 (9): 5288–96. DOI: 10.1128/AAC.00682-15.
- Krishnamoorthy G, Leus IV, Weeks JW, Wolloscheck D, Rybenkov VV, Zgurskaya HI. Synergy between Active Efflux and Outer Membrane Diffusion Defines Rules of Antibiotic Permeation into Gram-Negative Bacteria. MBio. 2017; 8 (5): e01172-17. DOI: 10.1128/mBio.01172-17.
- Omarova EO, Nazarov PA, Firsov AM, Strakhovskaya MG, Arkhipova AY, Moisenovich MM et al. Carboranyl-Chlorin e6 as a Potent Antimicrobial Photosensitizer. PLoS One. 2015; 10 (11): e0141990. DOI: 10.1371/journal.pone.0141990.
- Maliszewska I, Kałas W, Wysokińska E, Tylus W, Pietrzyk N, Popko K et al. Enhancement of photo-bactericidal effect of tetrasulfonated hydroxyaluminum phthalocyanine on Pseudomonas aeruginosa. Lasers Med Sci. 2018 Jan; 33 (1): 79–88. DOI: 10.1007/s10103-017-2337-0.
- Fekrazad R, Zare H, Vand SM. Photodynamic therapy effect on cell growth inhibition induced by Radachlorin and toluidine blue O on Staphylococcus aureus and Escherichia coli: An in vitro study. Photodiagnosis Photodyn Ther. 2016 Sep;15: 213–7. DOI: 10.1016/j.pdpdt.2016.07.001.
- Sperandio FF, Huang YY, Hamblin MR. Antimicrobial photodynamic therapy to kill Gram-negative bacteria. Recent Pat Antiinfect Drug Discov. 2013 Aug; 8 (2): 108–20.
- Мирошников К. А., Чертков О. В., Назаров П. А., Месянжинов В. В. Пептидо-гликанлизирующие ферменты бактериофагов — перспективные противобактериальные агенты. Успехи биологической химии. 2006; 46: 65–98.
- Suttle CA. Marine viruses--major players in the global ecosystem. Nat Rev Microbiol. 2007 Oct; 5 (10): 801–12. DOI: 10.1038/nrmicro1750.
- Wittebole X, De Roock S, Opal SM. A historical overview of bacteriophage therapy as an alternative to antibiotics for the treatment of bacterial pathogens. Virulence. 2014 Jan 1; 5 (1): 226–35. DOI: 10.4161/viru.25991.
- Twort FW. An investigation on the nature of ultramicroscopic viruses. Lancet. 1915 Dec 4; (4814): 1241–3.
- D'Herelle F. Sur un microbe invisible antagoniste des bacilles dysentériques. C R Acad Sci (Paris). 1917; 165: 373–5. French.
- Bruynoghe R., Maisin J. Essais de thérapeutique au moyen du bacteriophage. C R Soc Biol. 1921: 85: 1120–1. French.
- Barrow PA, Soothill JS. Bacteriophage therapy and prophylaxis: rediscovery and renewed assessment of potential. Trends Microbiol. 1997 Jul; 5 (7): 268–71. DOI: 10.1016/S0966-842X(97)01054-8.
- Sulakvelidze A, Alavidze Z, Morris JG Jr. Bacteriophage Therapy. Antimicrob Agents Chemother. 2001 Mar; 45 (3): 649–59. DOI: 10.1128/AAC.45.3.649-659.2001.
- Skurnik M, Strauch E. Phage therapy: facts and fiction. Int J Med Microbiol. 2006 Feb; 296 (1): 5–14. DOI: 10.1016/j.ijmm.2005.09.002.
- Lin DM, Koskella B, Lin HC Phage therapy: An alternative to antibiotics in the age of multi-drug resistance. World J Gastrointest Pharmacol Ther. 2017 Aug 6; 8 (3): 162–173. DOI: 10.4292/wjgpt.v8.i3.162.
- Levin B, Bull JJ. Phage therapy revisited: the population biology of a bacterial infection and its treatment with bacteriophage and antibiotics. Am Naturalist. 1996; 147: 881–98.
- Rea K, Dinan TG, Cryan JF The microbiome: A key regulator of stress and neuroinflammation. Neurobiol Stress. 2016 Oct; 4: 23–33. DOI: 10.1016/j.ynstr.2016.03.001.
- Mai V, Ukhanova M, Reinhard MK, Li M, Sulakvelidze A. Bacteriophage administration significantly reduces Shigella colonization and shedding by Shigella-challenged mice without deleterious side effects and distortions in the gut microbiota. Bacteriophage. 2015 Aug; 5 (4): e1088124. DOI: 10.10180/21597081.2015.1088124.
- Galtier M, De Sordi L, Maura D, Arachchi H, Volant S, Dillies MA et al. Bacteriophages to reduce gut carriage of antibiotic resistant uropathogens with low impact on microbiota composition. Environ Microbiol. 2016 Jul; 18 (7): 2237–45. DOI: 10.1111/1462-2920.13284.
- Servick K. Drug development. Beleaguered phage therapy trial presses on. Science. 2016 Jun 24; 352 (6293): 1506. DOI: 10.1126/science.352.6293.1506.
- Bourdin G, Navarro A, Sarker SA, Pittet AC, Qadri F, Sultana S et al. Coverage of diarrhoea-associated Escherichia coli isolates from different origins with two types of phage cocktails. Microb Biotechnol. 2014 Mar; 7 (2): 165–76. DOI: 10.1111/1751-7915.12113.
- Sarker SA, Sultana S, Reuteler G, Moine D, Descombes P, Charton F et al. Oral Phage Therapy of Acute Bacterial Diarrhea with Two Coliphage Preparations: A Randomized Trial in Children from Bangladesh. EBioMedicine. 2016 Jan 5; 4: 124–37. DOI: 10.1016/j.ebiom.2015.12.023.
- Latz S, Wahida A, Arif A, Häfner H, Hoß M, Ritter K et al. Preliminary survey of local bacteriophages with lytic activity against multi-drug resistant bacteria. J Basic Microbiol. 2016 Oct; 56 (10): 1117–23. DOI: 10.1002/jibm.201600108.
- Abedon ST. Ecology of Anti-Biofilm Agents I: Antibiotics versus Bacteriophages. Pharmaceuticals (Basel). 2015 Sep 9; 8 (3): 525–58. DOI: 10.3390/ph8030525.
- Gabisoniya TG, Loladze MZ, Nadiradze MM, Chakhunashvili NK, Alibegashvili MG, Tamarashvili NG et al. Effects of bacteriophages on biofilm formation by strains of Pseudomonas aeruginosa. Appl Biochem Microbiol. 2016; 52: 293–7.
- Motlagh AM, Bhattacharjee AS, Goel R. Biofilm control with natural and genetically-modified phages. World J Microbiol Biotechnol. 2016 Apr; 32 (4): 67. DOI: 10.1007/s11274-016-2009-4.
- Brüssow H, Canchaya C, Hardt WD. Phages and the evolution of bacterial pathogens: from genomic rearrangements to lysogenic conversion. Microbiol Mol Biol Rev. 2004 Sep; 68 (3): 560–602. DOI: 10.1128/MMBR.68.3.560-602.2004.
- Penadés JR, Chen J, Quiles-Puchalt N, Carpena N, Novick RP. Bacteriophage-mediated spread of bacterial virulence genes. Curr Opin Microbiol. 2015 Feb; 23: 171–8. DOI: 10.1016/j.mib.2014.11.019.
- Modi SR, Lee HH, Spina CS, Collins JJ. Antibiotic treatment expands the resistance reservoir and ecological network of the phage metagenome. Nature. 2013 Jul 11; 499 (7457): 219–22. DOI: 10.1038/nature12212.
- Quirós P, Colomer-Lluch M, Martínez-Castillo A, Miró E, Argente M, Jofre J et al. Antibiotic resistance genes in the bacteriophage DNA fraction of human fecal samples. Antimicrob Agents Chemother. 2014; 58 (1): 606–9. DOI: 10.1128/AAC.01684-13.
- Colomer-Lluch M, Jofre J, Muniesa M. Antibiotic resistance genes in the bacteriophage DNA fraction of environmental samples. PLoS One. 2011 Mar 3; 6 (3): e17549. DOI: 10.1371/journal.pone.0017549.
- Davis BM, Waldor MK. Filamentous phages linked to virulence of Vibrio cholerae. Curr Opin Microbiol. 2003 Feb; 6 (1): 35–42.
- Broudy TB, Fischetti VA. In Vivo Lysogenic Conversion of Tox− Streptococcus pyogenes to Tox+ with Lysogenic Streptococci or Free Phage. Infect Immun. 2003 Jul; 71 (7): 3782–6. DOI: 10.1128/IAI.71.7.3782-3786.2003.
- Wang X, Wood TK. Cryptic prophages as targets for drug development. Drug Resist Updat. 2016 Jul; 27: 30–8. DOI: 10.1016/j.drup.2016.06.001.
- Weinbauer MG. Ecology of prokaryotic viruses. FEMS Microbiol Rev. 2004 May; 28 (2): 127–81. DOI: 10.1016/j.femsre.2003.08.001.
- Krylov VN, Pleteneva EL, Bourkaltseva M, Shaburova O, Volckaert G, Sykilinda N et al. Myoviridae bacteriophages of Pseudomonas aeruginosa: a long and complex evolutionary pathway. Res Microbiol. 2003 May; 154 (4); 269–75. DOI: 10.1016/S0923-2508(03)00070-6.
- Mesyanzhinov VV, Robben J, Grymonprez B, Kostyuchenko VA, Burkaltseva MV, Sykilinda NN et al. The genome of bacteriophage fKZ of Pseudomonas aeruginosa. J Mol Biol. 2002 Mar 15; 317 (1): 1–19. DOI: 10.1006/jmbi.2001.5396.
- Hertveldt K, Lavigne R, Pleteneva E, Sernova N, Kurochkina L, Korchevskii R et al. Genome comparison of Pseudomonas aeruginosa large phages. J Mol Biol. 2005 Dec 2; 354 (3): 536–45. DOI: 10.1016/j.jmb.2005.08.075.
- Navarre WW, Schneewind O. Surface proteins of gram-positive bacteria and mechanisms of their targeting to the cell wall envelope. Microbiol Mol Biol Rev. 1999 Mar; 63 (1): 174–229.
- Beveridge TJ. Structures of gram-negative cell walls and their derived membrane vesicles. J Bacteriol. 1999 Aug; 181 (16): 4725–33.
- Delbrück M. The Growth of Bacteriophage and Lysis of the Host. J Gen Physiol. 1940; 23 (5): 643–60.
- Leiman PG, Chipman PR, Kostyuchenko VA, Mesyanzhinov VV, Rossmann MG. Three-dimensional rearrangement of proteins in the tail of bacteriophage T4 on infection of its host. Cell. 2004 Aug 20;118 (4): 419–29. DOI: 10.1016/j.cell.2004.07.022.
- Kanamaru S, Leiman PG, Kostyuchenko VA, Chipman PR, Mesyanzhinov VV, Arisaka F et al. Structure of the cell-puncturing device of bacteriophage T4. Nature. 2002 Jan 31; 415 (6871): 553–7. DOI: 10.1038/415553a.
- Roach DR, Donovan DM. Antimicrobial bacteriophage-derived proteins and therapeutic applications. Bacteriophage. 2015 Jul-Sep; 5 (3): e1062590. DOI: 10.1080/21597081.2015.1062590.
- Young R. Phage lysis: do we have the hole story yet? Curr Opin Microbiol. 2013 Dec; 16 (6): 790–7. DOI: 10.1016/j.mib.2013.08.008.
- Young R. Phage lysis: three steps, three choices, one outcome. J Microbiol. 2014 Mar; 52 (3): 243–58. DOI: 10.1007/s12275-014-4087-z.
- Henrissat B. A classification of glycosyl hydrolases based on amino acid sequence similarities. Biochem J. 1991 Dec 1; 280 (Pt 2): 309–16.
- Henrissat B, Bairoch A. Updating the sequence-based classification of glycosyl hydrolases. Biochem J. 1996 Jun 1; 316 (Pt 2): 695–6.
- Blackburn NT, Clarke AJ. Identification of four families of peptidoglycan lytic transglycosylases. J Mol Evol. 2001 Jan; 52 (1): 78–84.
- Höltje JV. Lytic transglycosylases. EXS. 1996; 75: 425–9.
- Fastrez J. Phage lysozymes. EXS. 1996; 75: 35–64.
- Weaver LH, Grütter MG, Remington SJ, Gray TM, Isaacs NW, Matthews BW. Comparison of goose-type, chicken-type, and phage-type lysozymes illustrates the changes that occur in both amino acid sequence and three-dimensional structure during evolution. J Mol Evol. 1984–1985; 21 (2): 97–111.
- Monzingo AF, Marcotte EM, Hart PJ, Robertus JD. Chitinases, chitosanases, and lysozymes can be divided into procaryotic and eucaryotic families sharing a conserved core. Nat Struct Biol. 1996 Feb; 3 (2): 133–40.
- Jaeger T, Arsic M, Mayer C. Scission of the lactyl ether bond of N-acetylmuramic acid by Escherichia coli "etherase". J Biol Chem. 2005 Aug 26; 280 (34): 30100–6. DOI: 10.1074/jbc.M502208200.
- Díaz E, López R, García JL. Chimeric phage-bacterial enzymes: a clue to the modular evolution of genes. Proc Natl Acad Sci U S A. 1990 Oct; 87 (20): 8125–9.
- Desiere F, Lucchini S, Brüssow H. Evolution of Streptococcus thermophilus bacteriophage genomes by modular exchanges followed by point mutations and small deletions and insertions. Virology. 1998 Feb 15; 241 (2): 345–56.
- Sheehan MM, Stanley E, Fitzgerald GF, van Sinderen D. Identification and Characterization of a Lysis Module Present in a Large Proportion of Bacteriophages Infecting Streptococcus thermophilus. Appl Environ Microbiol. 1999 Feb; 65 (2): 569–77.
- Hermoso JA, Monterroso B, Albert A, Galán B, Ahrazem O, García P, et al. Structural basis for selective recognition of pneumococcal cell wall by modular endolysin from phage Cp-1. Structure. 2003 Oct; 11 (10): 1239–49.
- López R, García E, García P, García JL. The pneumococcal cell wall degrading enzymes: a modular design to create new lysins? Microb Drug Resist. 1997 Summer; 3 (2): 199–211.
- Gilmer DB, Schmitz JE, Euler CW, Fischetti VA. Novel bacteriophage lysin with broad lytic activity protects against mixed infection by Streptococcus pyogenes and methicillin-resistant Staphylococcus aureus. Antimicrob Agents Chemother. 2013 Jun; 57 (6): 2743–50. DOI: 10.1128/AAC.02526-12.
- Yang H, Wang DB, Dong Q, Zhang Z, Cui Z, Deng J et al. Existence of separate domains in lysin PlyG for recognizing Bacillus anthracis spores and vegetative cells. Antimicrob Agents Chemother. 2012 Oct; 56 (10): 5031–9. DOI: 10.1128/AAC.00891-12.
- Loeffler JM, Fischetti VA. Synergistic lethal effect of a combination of phage lytic enzymes with different activities on penicillin-sensitive and -resistant Streptococcus pneumoniae strains. Antimicrob Agents Chemother. 2003 Jan; 47 (1): 375–7. DOI: 10.1128/AAC.47.1.375-377.2003.
- Fischetti VA Bacteriophage lytic enzymes: novel anti-infectives. Trends Microbiol. 2005 Oct; 13 (10): 491–6. DOI: 10.1016/j.tim.2005.08.007.
- Yang H, Zhang Y, Yu J, Huang Y, Zhang XE, Wei H. Novel chimeric lysin with high-level antimicrobial activity against methicillin-resistant Staphylococcus aureus in vitro and in vivo. Antimicrob Agents Chemother. 2014; 58 (1): 536–42. DOI: 10.1128/AAC.01793-13.
- Djurkovic S, Loeffler JM, Fischetti VA. Synergistic killing of Streptococcus pneumoniae with the bacteriophage lytic enzyme Cpl-1 and penicillin or gentamicin depends on the level of penicillin resistance. Antimicrob Agents Chemother. 2005 Mar; 49 (3): 1225–8. DOI: 10.1128/AAC.49.3.1225-1228.2005.
- Schmelcher M, Donovan DM, Loessner MJ. Bacteriophage endolysins as novel antimicrobials. Future Microbiol. 2012 Oct; 7 (10): 1147–71. DOI: 10.2217/fmb.12.97.