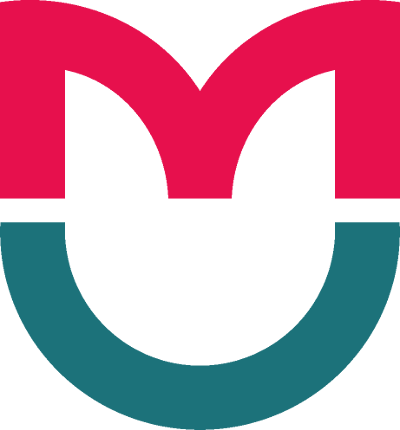
This article is an open access article distributed under the terms and conditions of the Creative Commons Attribution license (CC BY).
REVIEW
Diagnostic potential of cell-free DNA as a liquid biopsy marker
1 Laboratory of Pharmacogenomics,Institute of Chemical Biology and Fundamental Medicine of the Siberian Branch of the Russian Academy of Sciences, Novosibirsk, Russia
2 Novosibirsk State University, Novosibirsk, Russia
Correspondence should be addressed: Maxim L. Filipenko
Prospekt Akademika Lavrentieva, d. 8, Novosibirsk, Russia, 630090; ur.csn.hcobin@xam
Funding: this review was supported by the state-funded project for basic research (2017-2020) Development of methods of personalized medicine (VI.62.2.2, 0309-2016-0007).
In a broad sense, liquid biopsies are methods that have long been used in clinical routine to test for the presence of tumor markers in the blood plasma, measure activity of liver enzymes or concentrations of thyroid hormones, etc. However, this term has acquired a new meaning reflecting the hypothesis that nucleic acids of specialized body cells, including tumor and fetal cells, can find their way into biological fluids, from which they can be isolated in order to obtain clinically important information about their sources of origin. Considering the rapid development of methods for nucleic acid analysis and the accumulated knowledge about human genome and epigenome in health and pathology, analysis of DNA released into the systemic circulation (cell-free DNA, cfDNA) appears to be a very promising diagnostic tool. It is this type of cfDNA assays that is currently referred to as liquid biopsy.
Although the following review focuses on cfDNA, we would like to emphasize that various types of RNA can also provide diagnostically relevant information and be analyzed along with DNA.
Study of cfDNA: milestones
Cell-free DNA was first discovered in the peripheral blood plasma of humans by Mandel and Métais in 1948 [1]. Unfortunately, their work went unnoticed by the research community. Until the 1970s the majority of studies of cfDNA were carried out in patients with systemic lupus erythematosus and rheumatoid arthritis [2, 3, 4], focusing on the presence of free DNA in the blood serum. Those studies entailed significant advances in the methods of cfDNA quantification that were improved through the use of natural autoantibodies to various DNA types obtained from patients with systemic lupus erythematosus.
In 1977 Stroun et al. went on to give what has become a classical definition of cell-free and circulating DNA [5]. In the same year Leon et al. proposed a radioimmunoassay for cfDNA quantification based on the use of labeled DNA as antigens and the serum of patients with systemic lupus erythematosus as a source of antibodies [6]. In 93 % of healthy participants, cfDNA concentrations in the blood serum ranged from 0 to 50 ng/ml. Those values were taken as reference for healthy individuals, which was perfectly legitimate considering the evidence obtained later using state-of-the-art techniques. In Leon’s study concentrations of cfDNA in the blood serum of half of cancer patients (the main group) were considerably higher, ranging from 50 to 5000 ng/ml. After radiation therapy cfDNA concentrations went down in 66 to 90 % of patients with lymphoma, lung, ovarian, uterine and cervical cancers and in 16 to 33 % of patients with glioma, breast tumors and colorectal cancer. Patients with increased or unchanged post- radiation cfDNA levels showed no response to treatment. Later, detection of tumor-specific mutations, microsatellite instability and methylation of DNA circulating in the bloodstream confirmed its tumor-derived origin [7, 8, 9].
The end of the 20th century saw the invention of quantitative real-time polymerase chain reaction, a universal and well- reproducible technique for cfDNA quantification [10, 11], which also made it possible to determine ratios of differently sized cfDNA fragments in the sample [12]. Another milestone was marked in the history of cfDNA exploration by the employment of high-throughput sequencing for whole-genome analysis of specificity and quantification of cfDNA [13, 14].
How do nucleic acids get into the systemic circulation?
In their pioneer study Leon et al. concluded that tumors are not the only source of cfDNA circulating in the blood, as only half of their cancer patients had elevated plasma levels of cfDNA while the other half did not. Recently there has been evidence that concentrations of free DNA in the cells increase within the first few hours or days following cytotoxic chemotherapy administration; the DNA then disappears within a week. In pathology associated with increased cell death or tissue damage (hepatitis, sepsis or trauma), blood plasma concentrations of cfDNA also go up. These facts support the assumption that necrosis, apoptosis and perhaps a few other types of cell death largely determine concentrations of nucleic acids in the blood plasma, especially in cancer patients. Mechanisms of nucleic acid release from the cells might vary in different cancer types. In 1989 Stroun et al. hypothesized that in cancer patients cfDNA is released into the bloodstream mainly by tumor cells [15]; this hypothesis was corroborated by the discovery of oncogenic mutations in cfDNA isolated from the blood plasma of patients with leukemia [16] and pancreatic cancer [7].
Studies of fetal cfDNA demonstrated that its half-life is 16.3 min [17]. These findings were extrapolated onto all types of cfDNA and confirmed by further experiments conducted in animals who received DNA injections [18]. Analysis by gel electrophoresis revealed that cfDNA is fragmented and has characteristics similar to those of DNA isolated from apoptotic cells; it was also found that a small proportion of cfDNA is represented by high-molecular-weight fragments [19]. Jahr et al. believed that the presence of such fragments should be associated with necrosis of tumor cells. It should be noted though that this conclusion has been seriously questioned recently: while analyzing cfDNA obtained from cancer patients, Diehl et al. discovered that its high-molecular-weight fragments do not contain tumor-specific mutations often found in short (less than 200 b. p.) cfDNA fragments [20]. The researchers hypothesized that high-molecular-weight cfDNA could originate from phagocytosed necrotic cells.
According to the commonly shared belief, necrosis triggers release of high-molecular-weight cfDNA into the bloodstream. However, this type of cell death also induces production of large amounts of DNA packaged into typically sized nucleosomes [21, 22]. Normally nucleosomes are released from the cells 24 to 48 hours after apoptosis induction or 12 hours after its early signs begin to show [23]. Although the majority of nucleosomes released into the circulatory system are effectively eliminated by the liver, some of them can still be found in the blood or other bodily fluids. Elevated levels of nucleosomes in the blood can result from increased cell death induced by degenerative, autoimmune, inflammatory, ischemic, or toxin-mediated conditions and traumas, or from the presence of malignant tumors, when elimination mechanismsareoverloadedorcompromised.Thus,circulating nucleosomes can originate from the cells dying from apoptosis or necrosis or a combination of various forms of cell death, depending on the type and intensity of the stimuli and the energy state of the cell.
Another source of cfDNA in the systemic circulation is high- molecular-weight DNA of neutrophils. Neutrophil extracellular traps (NETs) are generated by activated neutrophils during NETosis [24], when nuclear constituents, including DNA molecules, citrullinated histones and granule enzymes, such as elastase, are released into the extracellular environment. Once released, they form web-like structures capable of capturing bacteria, ensuring high concentrations of antibacterial substances. It has become clear in the recent years that NETs can be “built” under sterile proinflammatory conditions, such as thrombosis, cancer, systemic lupus erythematosus, atherosclerosis, and diabetes. Formation of neutrophil traps can be stimulated by chemokines, such as interleukin 8 (CXCL8) [25, 26], and growth factors, such as granulocyte colony-stimulating factor (G-CSF) [27] and transforming growth factor-β. Depending on the microenvironment, expression of the aforementioned factors in the tumor can be stimulated above normal levels, i. e., NETosis may indicate the presence of a malignant tumor. In turn, NETs can stimulate tumor growth and angiogenesis, promote metastases and tumor-induced thromboembolism [28].
It is possible that cfDNA is excreted by exosomes. This mechanism is described in detail in the works by Peters and Reclusa [29, 30].
Finally, cfDNA can be a product of active metabolic secretion [31, 32, 33], coming from a complex it forms with glycolipoproteins and RNA. In their in vitro cell culture experiment, Aucamp et al. demonstrated a statistically significant correlation between the rates of glycolysis and cfDNA release. Fragments of released cfDNA were about 2,000 b. p. long, which ruled out the possibility of their apoptotic or necrotic origin. So far mechanisms and contribution of active secretion into the general pool of cfDNA remain understudied.
Cell-free DNA as a diagnostic tool
Prenatal screening
Following the discovery of fetal cfDNA in the maternal blood plasma [34], it was proposed to use this nucleic acid for noninvasive prenatal testing (NIPT) for fetal aneuploidies. Fetal cfDNA appears in the maternal blood in weeks 5–7 of pregnancy [35]. In the first trimester about 10 % of the total fetal cfDNA originate from apoptotic trophoblast cells. It was shown that data yielded by high-throughput sequencing are accurate enough to establish a clinical diagnosis [36]. A number of companies (Natera, Verinata, Sequenom, etc.) started to offer NIPT on a commercial basis. Analysis of fetal cfDNA in the maternal blood plasma is also actively used for early diagnosis of monogenic diseases [37, 38]. Fig. 1 exemplifies the use of fetal DNA in prenatal screening.
Free DNA is sometimes found in the follicular fluid and therefore used as a prognostic marker of embryo quality and success of in vitro fertilization (IVF) [39]. Having analyzed a total of 55 samples, Shamonki et al. discovered that embryonic DNA is present in spent culture media in concentrations ranging from 2 to 642 ng/μL [40]. Their findings were consistent with the results of preimplantation genetic screening of trophectoderm biopsy samples. If validated further, this method can make prenatal screening easier and improve IVF effectiveness.
Organ transplantation
Cell-free DNA can be used as a biomarker of organ transplant rejection. This clinically important application of cfDNA is based on the assumption that graft cells will die in case of rejection and their DNA will be released into the bloodstream of the recipient.
Survival rates for lung transplant recipients are poor compared to other types of transplants: diagnostic tests often fail to differentiate between infection and rejection. In their study de Vlaminck et al. observed a correlation between the levels of donor cfDNA in the recipient’s blood and the results of invasive rejection tests (AUC = 0.9). Moreover, the researchers demonstrated that cfDNA analysis can detect the presence of such pathogens as cytomegalovirus, herpesvirus HHV6 and HHV7 and adenovirus which are often underdiagnosed but frequently present in patients with lung transplants [41].
Bloom et al. observed a correlation between concentrations of donor cfDNA in the blood plasma of kidney transplant recipients measured by targeted high-throughput sequencing and allograft rejection status established histologically [42]. The researchers proposed the following reference values for donor cfDNA concentrations: levels < 1 % of total cfDNA indicate the absence of active rejection, levels > 1 % indicate a probability of active rejection. CareDx Clinical Laboratory has already started marketing the AlloSure dd-cfDNA assay for clinical diagnosis of acute rejection of kidney transplant.
Prediction of sepsis
Sepsis is a systemic inflammatory response to infection caused by pathogen dissemination through the bloodstream from the primary focus of infection to other organs and tissues. It is a common cause of death. Predicting sepsis outcome is difficult in patients with trauma or after surgery; new clinical biomarkers are needed to reduce mortality.
One of the forms of innate immunity is response of the organism to compromised blood sterility manifested as formation of neutrophil extracellular traps. The abovementioned NETs are constituted of high-molecular-weight cfDNA associated with histones, proteases and a few other proteins. A prospective pilot study enrolling 45 patients with multiple trauma (8 patients were excluded from the study after it had started) revealed an association between the initially high blood plasma levels of cfDNA/NETs (> 800 ng/ml) that increased again on days 5–9 of hospital stay and subsequent sepsis, multiple organ failure and death [43]. In contrast, dynamics of C-reactive protein levels did not differ significantly between patients who developed sepsis and those who did not. Further clinical validation is required to decide on the prognostic value of cfDNA/NETs.
A recent study by Hamaguchi et al. conducted in mice demonstrated that cfDNA concentrations are elevated in early stages of sepsis, rendering cfDNA an early biomarker of this condition [44]. In Hamaguchi’s experiment cfDNA was hypothesized to originate from necrotic cells and not neutrophils. If proved right by further research, this hypothesis may change the understanding of mechanisms of cfDNA production in sepsis.
Cancer
Development and progression of cancer are associated with accumulation of somatic mutations. Analysis of such mutations is increasingly used for diagnostic, prognostic and therapeutical purposes. At present, genetic profiles of solid tumors are determined by studying the DNA obtained from surgical resections or biopsies. Obviously, these invasive interventions cannot be frequently performed on the same patient. Besides, results of such DNA analysis may not reflect the heterogeneity and evolution of the whole tumor, since only a small piece of tissue incised at a particular time point is obtained for analysis. In contrast, tumor cfDNA isolated from the blood plasma or urine can provide sufficient data on the genetic features of the primary tumor or its metastases and help to track its genomic evolution. The past three years have seen over a thousand of published works about aspects of cfDNA study in patients with various types of cancer. The diagnostic potential of cfDNA as a biomarker of cancer is described in [45, 46, 47].
There are 3 approaches to the use of cfDNA for cancer diagnosis (fig. 2).
The first relies on measuring tumor cfDNA in the blood of a patient to establish accurate diagnosis or monitor tumor load. Although a lot of research works have convincingly demonstrated that blood cfDNA concentrations are elevated in cancer patients, cfDNA quantification assays are still not used routinely. Perhaps it is because of the absence of a standard protocol for cfDNA isolation and quantification and the low specificity the method has in cancer patients, compared to patients suffering from other diseases. Yet, as early as 1977 Leon et al. demonstrated that a short half-life makes cfDNA a very promising biomarker of tumor load [6]. Many protein tumor markers routinely used to monitor patient’s response
to treatment (PSA, CA125, CEA, αFP) stay in the systemic circulation for up to several days [48], while the half-life of cfDNA is only a few hours [49, 50]. Detection of somatic mutations and epigenetic modifications during quantification of tumor cfDNA can improve the diagnostic value of the method [51, 52, 53]. Targeted high-throughput sequencing for quantification of tumor-derived cfDNA following surgical resection of colorectal tumors can be successfully used to accurately predict cancer recurrences (HR = 18; 95 % CI 7.9–40.0; p < 0.001) [54].
Notably, the work by Tie et al. [54] demonstrates that detection of driver somatic mutations in cfDNA can facilitate the choice of treatment and that cfDNA can be used in screening tests for cancer. A recent study by Bettegowda et al. showed that tumor cfDNA is found in 73, 57, 48 and 50 % of patients with localized colorectal, gastroesophageal, and pancreatic cancer and breast adenocarcinoma, respectively [55]. It was shown that tumor cfDNA is found in more than 75 % of patients with metastatic tumors of the pancreas, ovaries, colon, bladder, breast, skin and liver. Further improvement of the method can increase its accuracy, while reasonable cost can make it attractive for the use in clinical practice.
The third approach pertains to cfDNA analysis of tumor- specific epigenetic modifications. In 2016 Margolin et al. [56] discovered that ZNF154 of cfDNA has a highly conserved hypermethylation profile typical for various cancer types. If validated, this pan-cancer biomarker will be an effective diagnostic tool. In 2016 another pioneer work by Lehmann- Werman et al. demonstrated that total methylation of cfDNA circulating in the human blood plasma can be “mapped” onto DNA fractions corresponding to specialized human cells [57]. It means that using high-throughput sequencing, an organ or tissue (or a cell type) can be identified that is affected by pathology and produces high amounts of specific cfDNA, given that there are age-related or other reference intervals for total cfDNA concentrations in the blood plasma. This method has a potential to become an effective non-invasive screening tool facilitating early diagnosis of cancer and may prove be useful in the development of targeted panels for high-throughput sequencing, as it can help to reduce screening costs.
Positions of nucleosomes are another epigenetic modification relevant for cfDNA analysis. They influence the structure of cfDNA fragments released during apoptosis. Patterns of cfDNA fragmentation were described in a number of works, including a 2016 work by Snyder et al. who reported that deep cfDNA sequencing allows identifying positions of nucleosomes and transcription factors, specific for certain cell types [58]. The researchers showed that cfDNA of healthy donors resembles most that of lymphoid and myeloid cells. At the same time, sequencing of cfDNA obtained from the blood plasma of patients with various cancer types matched the studied nucleic acids to 76 corresponding cell lines. It was concluded that the method could be used for detection of acute or chronic pathology of human organs and tissues.
Although the importance of non-invasive screening for cancer can hardly be overestimated, only one such assay has been registered with Food and Drug Administration so far. It is the cobas EGFR Mutation Test v2 (Roche Molecular Systems, USA, registered in 2016) for detecting somatic activating mutations of EGFR in the blood plasma.
Owing to the use of cfDNA analysis, a few interesting clinical cases were made known. For example, in their work Smith et al. obtained discordant results between cfDNA analysis and diagnostic fetal karyotyping [59]. Later, it became clear that the patient had colorectal adenocarcinoma. The researchers emphasized the need for a very accurate interpretation of anomalies discovered during cfDNA analysis and advocated the use of a multidisciplinary approach.
Other applications
Increased concentrations of cfDNA in the blood plasma and serum of patients with autoimmune diseases, especially patients with systemic lupus erythematosus, have been demonstrated in many research studies [4, 60, 61]. However, there are still no unambiguous recommendations as to how to use cfDNA quantification assays in clinical routine.
Ershova et al. [62] studied concentrations of cfDNA and 8-Oxo-2'-deoxyguanosine (8-oxodG) in cfDNA of patients with acute psychotic disorders. Elevated levels of 8-oxodG were found in cfDNA and lymphocytes (FL1-8-oxodG). Considering that the ratio of cfDNA to FL1-8-oxodG reflects the level of apoptosis in the damaged cells, the scientists concluded that an increase in the number of cells with damaged DNA in body tissues may have an impact on the etiology of acute psychosis.
Breitbach et al. proposed to monitor cfDNA concentrations in athletes [63]. Long endurance exercises may lead to chronic inflammation that in turn stimulates continuous slow release of DNA from apoptotic or necrotic cells. Thus, cfDNA may be used as a biomarker of overtraining. To validate this hypothesis, prospective studies are necessary that would be conducted in heterogeneous groups of athletes performing controlled physical exercise.
Chronic obstruction of the lungs characterized by unproductive neutrophilic inflammation in the respiratory tract accompanies progression of cystic fibrosis and leads to death. Upon contact with a pathogen or following their long activation, neutrophils release the aforementioned traps containing large amounts of DNA. NETs were long considered a defensive tool because of their antibacterial and antifungal properties. However, their excessive formation is associated with autoimmune diseases. Marcos et al. conducted analysis of cfDNA (putatively NETs-derived DNA) and showed that cfDNA concentrations correlate positively with pulmonary obstruction, colonization of the lungs by microorganisms, and chemokines levels in patients with cystic fibrosis and in model mice [64]. Thus, neutrophilic inflammation in the lungs in cystic fibrosis may be associated with considerably increased cfDNA concentrations typically observed in NETosis and may cause lung dysfunction. If this assumption is valid, it renders possible the use of DNAse, antiprotease and other NETosis inhibitors as therapeutical agents against cystic fibrosis.
Finally, differential methylation of the promoter of PPARγ found in cfDNA circulating in the human blood plasma may correlate with different degrees of severity of liver fibrosis in patients with non-alcoholic fatty liver disease [65].
The above list is by no means exhaustive. It is constantly expanding as methods for quantification and structural analysis of cfDNA in various biological fluids are being improved.
CONCLUSION
A globally growing interest in cell-free DNA reflects a great potential of non-invasive liquid biopsy as a diagnostic and monitoring tool that can be used in patients with cancer, stroke, myocardial infarction, autoimmune disorders, traumas, and pregnancy complications. So far, there is no clear understanding as to whether cfDNA has specific functions in the cell or the organism and what its molecular mechanisms are in health and pathology. These questions can be elucidated by biologists, bioinformatician, specialists who study evolution of humans, and clinicians. Besides, inclusion of liquid biopsy into clinical routine requires optimization of technologies at all stages of the diagnostic process.