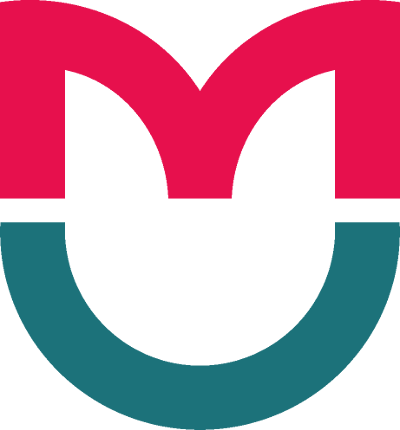
REVIEW
Immunological memory as a basis for a wise vaccination strategy. A rationale for introducing a comprehensive seroepidemiological surveillance system in Russia
1 Laboratory of Translational Biomedicine,N. F. Gamaleya Federal Research Center for Epidemiology and Microbiology, Moscow, Russia
2 Department of Virology, Faculty of Biology,Lomonosov Moscow State University, Moscow
3 Institute of Molecular Medicine,I. M. Sechenov First Moscow State Medical University, Moscow, Russia
4 Department of Epidemiology,N. F. Gamaleya Federal Research Center for Epidemiology and Microbiology, Moscow, Russia
5 N. F. Gamaleya Federal Research Center for Epidemiology and Microbiology, Moscow
Correspondence should be addressed: Vladimir A. Gushchin
ul. Gamalei, d. 18, Moscow, Russia, 123098; moc.liamg@adainawow
Funding: this paper was prepared with support of the Russian Ministry of Education and Science, project no. RFMEFI60117X0018.
All authors' contribution to this work is equal: selection and analysis of literature, planning of the manuscript's structure, data interpretation, drafting of the manuscript, editing.
Vaccination is a powerful and cost-effective tool for fighting infection. It confers direct protection against pathogens and establishes a long-lasting immunological memory [1]. In spite of significant achievements in preventive vaccination, development of novel or improved vaccines is hampered by the lack of fundamental immunological knowledge, excessive bureaucratic regulations, financial and sociopolitical barriers, which also contribute to the irrational use of older preparations. For example, about 20 million children currently do not have access to routine vaccination (see the Figure), which entails deaths of several million people each year [1]. Even more infected people die because there are no vaccines against the pathogen (HIV, malaria) [2] or because the vaccine is only partially protective (tuberculosis) [3].
Until the 1970s, the list of recommended vaccines against life-threatening infections was quite short, and preventive vaccination basically aimed to cover the entire population regardless of age. Among the greatest achievements of that time was complete eradication of smallpox and partial elimination of measles, polio, diphtheria, tetanus and tuberculosis (reported in a few developed regions in Europe and North America). Current immunization schedules for children, teenagers and adults are increasingly intricate and offer vaccinations against multiple pathogens with dozens of serotypes.
Immunization schedules ensure the best use of available vaccines and also serve to accurately estimate the need for vaccination among different population groups. The list of recommended vaccines is constantly expanding, further complicating the immunization schedule. Still, the latter cannot be revised unless we start to monitor the efficiency of current vaccination programs and obtain a deeper understanding of herd immunity structure [5].
It is known that immunity of those who have developed immunological memory against a particular pathogen (through vaccination or natural infection) protects susceptible members of the population from this infection. This phenomenon is commonly referred to as herd immunity. Threshold proportions of immunes necessary for establishing herd immunity against a particular infection vary depending on the number of individual contacts in the population and the probability of infection transmission which, in turn, depends on the pathogen. For example, the spread of diphtheria, polio and rubella can be controlled if the proportion of immune individuals evenly distributed in the population is 82 % to 87 %; with mumps, the threshold value is 85 % to 90 %, and with measles and pertussis, this proportion is 92 % to 95 % [6].
It should be noted that a hundred percent immunity against infection is nonexistent: not every healthy individual develops a long-lasting post-vaccination immunological memory [7]. So far, factors determining this selectivity, including patient’s genotype, remain understudied [8]. Also, particularly vulnerable to infection are patients with compromised immunity, HIV, or cancer [9]; infants in whom vaccination can be ineffective or unsafe [10], and elderly people with waning immunity [11]. Besides, even trustworthy vaccines sometimes differ in their effectiveness depending on the lot or season [10, 12]. Therefore, maintaining a high proportion of truly immunized individuals is crucial for the population in general and its vulnerable members in particular.
Importantly, herd immunity can be established only if immunized individuals are distributed evenly in the population [5]. It is known that geographical and social clustering of vaccine-hesitant groups, e. g. religious communities, seriously reduces herd immunity in the regions of their residence. This phenomenon has been demonstrated in the Mennonites (USA), indigenous peoples of the US and Canada, and religious communities in the Netherlands [6, 13]. Increasing vaccine hesitancy both in Russia and worldwide has become a high-profile issue [14, 15] and sparked serious discussions among the scientists [16, 17, 18, 19, 20]. Understanding the landscape of population immunity is critical for better identification of susceptible individuals and requires targeted serological surveillance.
Serological surveillance is an important tool used to monitor infection, identify at-risk populations and predict new threats. It provides information that is often missing from epidemiological and vaccination reports [20]. Modern serological surveillance relies on quantitative and qualitative measurements of specific antibodies in the blood serum that indicate post-vaccination or naturally acquired immunity and protect an individual from infection [21]. Antibodies generated in response to disease point to clinical or even subclinical infections that otherwise could be overlooked. For example, 20 % of infants with no medical history of pertussis and no previous vaccinations against this infection were found to have antibodies to the pertussis toxin [22]. Centralized serological surveillance of population immunity should be a part of any large-scale vaccination campaign.
Basic principles of serological surveillance
Any state-supported system for serological surveillance [23, 24] aims to obtain reliable data to estimate herd immunity, assess efficiency of current immunization programs and the need to revise immunization schedules and vaccine procurement volumes. Therefore, such a system should:
1. determine the actual proportion of individuals with protective titers against pathogens circulating in endemic areas, including infections that can be controlled by specific prevention measures, using appropriate stratification if required;
2. assess efficiency of current immunization programs from regional, socio-economic and age perspectives by comparing vaccination coverage statistics with the actual figures on the truly immune population;
3. update the statistics on herd immunity in population groups or areas (herd immunity refers to the percent of immune individuals in a studied population, sufficient to prevent an epidemic).
The main criteria a comprehensive serosurveillance system should meet are quality and quantity of the obtained data ensured by adherence to the following principles [25, 26]:
– adequate coverage: surveillance should be performed over the targeted population or environmental objects critical for accurate estimation of prevalence of infection markers (depending on the pathogen type, its endemicity, modes of transmission and risk factors); methods of statistical analysis should be applied;
–relevance: samples should be representative of the studied group or object; studies should be conducted in accordance with the principles of modern epidemiology;
– continuity: continuity of surveillance, i.e. a possibility to reproduce a study over the course of a few years;
– data homogeneity implies that data are homogenous enough to be pooled for further analysis within a given epidemiological and time frames.
A serosurveillance system would be impossible without authorized experts whose primary task is to collect, process and archive the samples using quality lab equipment and their own data processing centers. The need for a serosurveillance system was plainly indicated by the Russian Federation Government [23] and stems from the current state of the field.
Current methods for estimating herd immunity in Russia
At present, no large-scale studies are being conducted in Russia to estimate the prevalence of serological markers of vaccine-preventable infections whose results could be reliably extrapolated onto the entire population. Effectiveness of immunization programs is normally indirectly assessed by
- analyzing documented vaccination coverage rates;
- analyzing infection incidence rates,
- local seroepidemiological studies.
We will enlarge on these methods below.
Vaccination coverage
Every authorized vaccination provider is expected to fill out two statistical report forms [27, 28]: Form 5 that reports the absolute number of administered vaccine doses, and Form 6 that lists population groups eligible for vaccination before reaching the certain (decreed) age stated in the National immunization schedule [29, 30]. Based on these forms, the following parameters are calculated: vaccination coverage among children, i. e. the proportion of children who have reached a recommended age for vaccination and thus received at least one vaccine from a full vaccination course; total vaccination coverage, i. e. the proportion of individuals of all ages who have received a full vaccination course; timeliness, i. e. the proportion of children who received their vaccines on time, i. e. before turning a certain age (see [31] for details). These reports are then collected by Rospotrebnadzor (the Russian Federal Service for Surveillance on Consumer Rights Protection and Human Wellbeing) [32, 33], and immunization coverage data get included into the Annual State Report [34].
This approach accounts for every country’s resident who has received a vaccine, but does not account for those who have not received it. First, to calculate the proportion of vaccinated individuals, one should know the exact size of a population subgroup, but healthcare facilities (HCFs) that administer vaccines to their patients only have information about those who are registered with the facility (the so-called census population) [33]. Migration and failure to register with a local practice render these data unrepresentative of the actual size of different subpopulations in the society. Besides, in the absence of a single unified database of electronic medical records, information about vaccines administered to an individual is lost once they move to a different area. Second, even the most accurate estimates of patients vaccinated during the year are not representative of the size of the population vaccinated at any time point in their life. For example, the work by Tsvirkun [35] reports the rates of measles vaccination coverage in children from 1968 to 2013. In the late 1960s immunization coverage was 30–50 %, by the end of the 1980s it had reached 75 %, and by 2013 — 97 % to 98 %. In spite of seemingly inspiring immunization coverage, mathematical modeling predicted [35] that by the year 2014 the proportion of susceptible individuals would be as high as 25.6 %. Of 74.4 % of immune individuals, 50 % were previously vaccinated, while other 24.4 % acquired immunity after the disease. These figures differ drastically from thresholds necessary for measles eradication [36, 37, 38, 39].
Another example of vaccine preventable infection is hepatitis B. The federal hepatitis B immunization program for newborns was launched in Russia in 2001 [40, 41]. Unfortunately, details on the immunization coverage in infants before the year 2010 have never been published, but between years 2010 and 2016 the rates were about 97 % [42]. By comparing these figures to birth rates in that period (about 2 million births a year [43]), one can assume that at least 14 million children have been vaccinated against hepatitis B so far, accounting for 10 % of the country’s population. But such high immunization coverage does not provide any information about the total number of Russians who have received the vaccine by now. First, we do not know how many people had become immune by 2001 (either through vaccination or after the disease) and how many newborns were vaccinated between 2001 and 2009. Second, there is only scarce data available on vaccinations between 2006 and 2008 [44, 45], arguing that 18.5 to 25 million adults, teenagers and infants were vaccinated outside the immunization schedule. These data are not sufficient to infer the number of Russian residents who have already received a vaccine against hepatitis B and/or have immunity to this disease.
Finally, vaccination does not always mean immunization [7, 10, 12]. Therefore, documented immunization coverage cannot be regarded as a criterion to estimate herd immunity.
Analysis of infection incidence rates
Incidence is defined as a proportion of new cases of infection within a period of time with respect to the total population [46]. Every case gets listed in the expedited report No. 058/U [47, 48] and in the statistical report Form 2 [27]. These forms are sent over to Rospotrebnadzor [32, 33] for summarizing and publishing [34]. Incidence rates are an objective criterion for public health, which is determined, among other things, by immunization coverage and effectiveness. However, incidence rates cannot be used to measure herd immunity for a number of reasons.
First, only new cases of infection are registered, specifically in patients who decided to seek advice of a doctor. Therefore, the actual incidence remains unknown. Moreover, a cohort of patients who regularly visit a doctor may be absolutely unrepresentative of the entire population [25].
Second, a number of infections require laboratory confirmation for diagnosis. This diagnostic step is very likely to be skipped if a local primary care facility is poorly equipped [49]. Good examples here are flu and acute viral respiratory infection. According to the official statistics, in 2016 flu afflicted only 88.5 thousand Russians (0.06 % of the population), while AVRI of unknown etiology — 31.7 million people (about 20 % of the population) [34]. Similar incidence rates were reported earlier [42, 50]. In contrast, as many as 22–29 million US residents (7 % to 9 % of the population) were diagnosed with flu during the non-epidemic season of 2015-2016 [51], while in Japan the annual incidence of flu was 10–16 % in 2010–2014 [52, 53, 54]. A clear disparity between flu and AVRI incidences typical for Russia is explained by the fact that AVRI can be diagnosed based on the symptoms, while flu diagnosis requires laboratory confirmation [55]. Flu is a vaccine-preventable infection, while AVRIs are not; therefore, underreported flu incidence prevents us from assessing the success of immunization programs.
Third, for some infections the number of new cases only loosely correlates with their prevalence. For example, hepatitis B is manifested either as a chronic or acute condition [56]. According to the official statistics, the incidence of acute hepatitis B decreased from 141 cases per 100,000 people in 1999 to 22.7 cases in 2016, which was largely due to the immunization program for newborns and the successful war on drugs [57, 58]. The total number of Russians infected with hepatitis B was determined by screening various population groups for the presence of HBsAg in the blood serum and accounted for 2 % to 4 % of the population (3–6 million virus carriers). This percentage seems to be stable over the years [59, 60, 61, 62] due to the epidemiological specifics of virus dissemination [63]. The changing number of new registered hepatitis B cases does not correlate with the actual incidence of the infection in the population and is a poor marker of herd immunity against this disease.
An important metric related to incidence is mortality; however, in Russia the method used for calculating mortality may distort the resulting data. WHO estimates that infections account for 25 % of total deaths worldwide and for 10 % of deaths in the developed countries [2]. But according to the official statistics [43], between 2010 and 2014 only 1.7 % to 3.1 % of deaths were caused by infections in Russia [64]. The reason is simple: many fatal tumors (especially gynecologic cancers), gastrointestinal and cardio-vascular diseases are caused by infections, but it is impossible to reliably identify the cause of the disease in a person who dies of its complications or co-morbidities [65]. This means that data on infection-related deaths are inaccurate and cannot be used to estimate herd immunity.
Seroepidemiological studies
In Russia, there is a state-supported system for serological surveillance of vaccine-preventable infections in the target populations [31, 33, 36], operating via regional centers for hygiene and epidemiology of Rospotrebnadzor and core research facilities [35, 55]. This system, however, is not intended for studying herd immunity, but rather for investigating resistance to a particular infection [67] in vaccinated individuals representing differently aged sentinel populations [68]. This information is essential when assessing vaccination effectiveness, but such type of surveillance does not allow studying herd immunity of the entire country’s population.
A wealth of information could be provided by population studies of prevalence of serological markers (including antibodies to pathogens) conducted by research institutions on their own initiative. Unfortunately, such studies are rare, carried out in small populations, published in different journals, and do not build a holistic picture of herd immunity in Russia.
Below are a few examples of such research works. The study [69] demonstrated that only 60 % to 78 % of 300 Leningrad oblast residents (the proportion varied depending on the age group) had antibodies to rubella. The samples used in that work were collected back in 1995, before the state- funded rubella vaccination program was launched in 2000 and revaccination was made compulsory in 2002 [41]. Another study of 779 samples obtained from St. Petersburg residents in 2013 [70] demonstrated that only 82.1 % of individuals were immune to rubella, indicating a weak effect of vaccination.
Data on the true prevalence of protective antibodies to mumps directly correlate with immunization coverage rates. Thus, according to State Research Center of Virology and Biotechnology VECTOR (Novosibirsk, Russia [71]), by the year 2006 a total of 90–95 % of the population had been vaccinated against mumps. The authors of [72] report that 97 % of 60 children examined in Novosibirsk at that time had antibodies to mumps. The study [73] carried out in Moscow in 904 individuals of different age revealed the presence of protective antibodies in 93 % of the participants.
In another study of post-vaccination immunity against hepatitis B conducted in 2013 in 970 residents of St. Petersburg, 55 % of the population were shown to have antibodies to HBsAg [74]. Interestingly, similar studies that used samples obtained before national hepatitis B immunization programs were launched (2001 [41]) yielded lower estimates of herd immunity. Thus, [75, 76] demonstrate that only 19–52 % of indigenous peoples of Siberia (sample sizes ranged from 194 to 657 individuals, samples were collected between 1993 and 2002) have antibodies to HBsAg. Similar data were obtained in another study [77] conducted in 302 Moscow residents in 2011–2013: antibodies to HBsAg were found in 20.9 % of the participants. Considering that mean age of the participants was 45.5 ± 8.6 years, one could assume that unvaccinated individuals prevailed in the sample. Based on the comparison of these three works, an inference could be made that the national hepatitis B immunization program is effective, if the data were not coming from such diverse populations.
The aforementioned methods of studying herd immunity in Russia cannot yield reliable results, even if they are used in combination. There is a need for a new national system of serological surveillance. But before elaborating on the issue, let us have a look at how serological surveillance operates abroad.
International programs for seroepidemiological surveillance and their results
To estimate immunization coverage, information systems are being developed or already operating in the USA and Europe, recording all administrations of vaccines to individuals. According to the criteria set by the Centers for Disease Control and Prevention [78], such systems:
– operate at the population level and are defined as computerized confidential databases that keep track of all vaccines and their doses administered by authorized providers to the residents of the region;
– promptly provide consolidated records of patient’s immunization history;
– aggregate vaccination data at the population level for further use in epidemiological surveillance and public health control, or for estimating immunization coverage and incidence of vaccine-preventable infections.
In 2016 such systems were operating at the national level in 10 European countries (Germany, Sweden, Netherlands, Norway, and others); 5 countries had more than one subnational surveillance system (UK, Austria, etc.); 6 countries were launching pilot serosurveillance projects (France, Bulgaria, etc.) [79]. The global immunization plan for 2015–2020 describe these systems as “the integral part of a well- functioning health system” [80]. But although they ensure an accurate assessment of vaccination effectiveness, they fail to estimate the actual herd immunity, because administration of a vaccine does not necessarily lead to a successful immunization. Discrepancy between documented and actual vaccinations, imperfect tools for vaccine count and a few other factors promote the need for subnational and national projects aimed to study vaccine-induced immunity at the population level by collecting information (demographics, life histories, vaccination histories, etc.) and biosamples (blood). The samples are stored in biobanks [81] and used for laboratory-based seroepidemiological studies. The examples of national programs for serosurveillance are given below.
One of the vastest nation-wide studies of population immunity was conducted in the Netherlands and included two stages: the first referred to as PIENTER 1 took place in 1995–1996, and the second (PIENTER 2) — in 2006–2007; the project PIENTER 3 is ongoing. In the first stage 9,948 samples of blood serum were collected and tested for the presence of antibodies against 7 infections included in the national immunization schedule at that time, namely diphtheria, tetanus, measles, mumps, rubella and haemophilus influenzae type B [82, 83]. Samples were also tested for serological markers of hepatitis A, B and C (to detect chronic cases) and toxoplasmosis [84, 85, 86]. Data obtained in the course of PIENTER 1 were used to revise the national immunization plan; in the course of PIENTER 2 the list of surveyed infections was expanded to include meningococcal infection, human papillomavirus and varicella. In the second stage 6,386 samples were tested for the presence of 19 marker antibodies (for some pathogens more than one marker was proposed) [5, 87]. Both PIENTER 1 and PIENTER 2 were vigorously discussed in academic journals and national media, contributing to country’s authority in the field of epidemiology.
In 2006 another study was conducted in Belgium aimed to estimate the prevalence of markers of 5 vaccine-preventable infections (measles, mumps, rubella, diphtheria and tetanus) in 3,974 samples. The study identified a few age groups with low immunity; additional immunization was recommended for these groups [88]. The data were confirmed by and supplemented with findings of Belgian researchers who also studied pertussis markers in 1,500 samples in 2012 [89, 20].
Between 2003 and 2006, another research study was carried out in Germany: 13,900 samples of blood were collected and tested for the presence of antibodies to measles, mumps and rubella. Initially, the samples were intended to be used in other public health programs [90]. So far, similar projects have been launched in China, Japan, USA, Italy, and other countries, signaling the significance of serosurveys. The summary of national surveillance programs is given in Table 1.
Importantly, serological studies provide a possibility to update herd immunity statistics in various social groups and regions, revise immunization schedules, estimate the effectiveness of immunization programs and the amount of vaccines and diagnostic assays required for their implementation.
Routine vaccination ensures sufficient resistance to specific pathogens but does not guarantee complete control over infection outbreaks, calling for additional programs that would cover particular age groups or regions at risk. Incident cases of infection in a susceptible population may indicate the need for vaccination, but it might be too late for the latter once an epidemic breaks out. It is easy to lose control over the situation if the infection has long been “forgotten”, which does not mean that stable herd immunity has been fully established. Epidemiological modeling based on serological surveillance is a more reliable analytical tool [105]. For example, in response to a diphtheria outbreak in Eastern Europe, a serological survey was conducted revealing the lack of protective titers against the anatoxin, and an additional course of vaccination was proposed for the elderly population [106].
Serological surveillance has been helping to keep measles at bay in many countries, as it can identify the optimal vaccination age for susceptible groups of population. In the UK in the mid-1990s serological surveillance and mathematic modeling used in combination predicted a measles epidemic [107, 108]. Subsequently, a vaccination campaign was launched covering over 92 % of children aged 5 to 16 years. Further surveillance revealed that although the campaign was, on the whole, successful, it did not reduce susceptibility to measles in preschool children. This evidence was sufficient to prove the necessity of second dose administration of the MMR-vaccine (Measles/Mumps/Rubella) as part of immunization routine. A similar situation was observed in Australia [110, 111]. Those examples prove the importance of serological surveillance for maintaining herd immunity using vaccines with well-known properties.
Examples of regular and timely revisions of national immunization schedules inspired by seroepidemiological studies in the Netherlands indicate that serosurveillance has become an irreplaceable analytical tool [5]. Based on PIENTER 1 results, routine vaccination was introduced against pneumococcal infection, the hepatitis B immunization schedule was revised, the Infanrix-IPV + Hib vaccine was substituted with Pedicacel, and a recombinant vaccine was proposed instead of previously used separate vaccines (DT-IPV and aP at the age of 4) [112]. All changes to immunization schedules were assessed in the course of PIENTER 2. During PIENTER 2, vaccines against meningococcal infection were introduced to be administered at 14 months and 19 years (in parallel with MMR), and a new vaccine against human papillomavirus was proposed for girls at 12 years [5]. Effectiveness of new vaccines and duration of vaccine-induced immunity can be estimated by further serosurveillance. Studies of genotype composition of circulating strains and their serotypes can also stimulate development of new effective vaccines [113, 114, 115].
Laboratory techniques for studying herd immunity
Screening tests are an essential component of serological surveillance, contributing to our understanding of herd immunity. According to [68], the following diagnostic techniques are employed by serological studies: the indirect hemagglutination test (IHAT) for the detection of antibodies to measles virus, diphtheria and tetanus anatoxins; the agglutination test (AT) for the detection of pertussis agglutinins; the enzyme-linked immunosorbent assay (ELISA) for the detection of antibodies to measles, rubella, mumps, hepatitis B and pertussis; the tissue-culture virus-neutralization test for the detection of antibodies to polio. ELISA is a very common method for quantitative and qualitative estimation of IgG antibodies in human blood serum. However, it may not be the best option when a sample needs to be tested for several different infections or when the number of samples is too big, because each marker is analyzed in a separate run. Therefore, large-scale serological studies of vaccine effectiveness employ multiplex immunoassays (MIA) capable of determining immunoglobulin antibodies to several antigens in parallel. Advantageously, MIA can be performed on very small serum samples (5 μL) [116], making this assay type convenient for underage patients. Multiplex assays are also very flexible as they can be expanded to incorporate additional antigens [117].
There are a few commercial platforms for multiplexing. Although the techniques and reagents for sample preparation and marker detection employed by these systems are different, all of them utilize polystyrene microsphere beads as solid phase. One of the most popular diagnostic platforms is xMAP (Multiple Analyte Profiling). Its key component is polystyrene beads internally labeled with different ratios of 2 to 3 spectrally distinct fluorophores thus generating 50 to 500 unique bead regions and subsequently combining the beads into a multiplex assay [118].
Importantly, the majority of multiplex assays for serosurveillance are in-house manufactured and optimized for specific tasks. Designed to quantify blood serum IgG antibodies to at least one pathogen, they have a capacity to simultaneously work with up to 23 different antigens, such as pneumococcal capsular polysaccharides [119]. Some researchers propose their own multiplex platforms in order to compare their performance with standard immunoassays, evaluate their sensitivity, reproducibility and specificity. Others seek to design a good diagnostic tool for serological surveillance that would be capable of simultaneous detection of several infections. For example, the Belgian team has proposed an assay for measuring antibodies to diphtheria, tetanus and pertussis to estimate herd immunity of Belgium residents to these infections, compare the obtained data with previous serological findings and decide whether this novel platform can be used in large-scale research studies [20]. This assay incorporates 5 antigens: diphtheria, tetanus and pertussis toxins, filamentous hemagglutinin and pertactin, linked to the surface of magnetic polystyrene beads [118]. Its performance was tested using serum samples of Belgians aged 20–29.9 years; the assay was then validated using a different panel of 37 serum samples and compared with ELISA results. It was discovered that 26.4 % of the participants had low levels of antibodies to diphtheria toxin (below the protective threshold, < 0.1 IU/ml). Susceptibility to tetanus was observed in 8.6 % of cases. A good correlation was observed between the multiplex and monoplex assays [20]. The multiplex assay results also correlated well commercial ELISAs (Table 2). According to FDA (Food and Drug Administration, USA) and EMA (European Medicines Agencies, ЕС) guidelines, a validated assay for antibody quantification is expected to demonstrate precision of ≤ 20 % coefficient of variance [120]. Therefore, we conclude that results of an xMAP-based MIA are highly precise. Another important characteristic of a diagnostic serological test is the limit of detection (LOD) unique for each antigen in the multiplex. LOD established for the described pentaplex assay was sufficient for screening purposes (Table 2).
Another assay was proposed by Dutch researchers for the detection of antibodies to the same 5 antigens [121]. Using a small serum sample, the team attempted to assess how reliable their assay was for serological diagnosis. It proved to have a lower LLOQ (lower limit of quantitation) than ELISA: 0.00078 EU/ml vs. 2 AU/ml for the pertussis toxin, respectively, and 0.00006 IU/ml vs. 0.01 IU/ml for the diphtheria toxin, respectively [121]. But the correlation coefficient was quite high (Table 3).
Specificity of each monoplex component of a multiplex assay is often tested by conducting homologous and heterologous inhibition experiments [116, 119, 120, 121]. The serum sample containing high levels of IgG antibodies to all studied infections is divided into aliquots whose number corresponds to the number of antigens; then each aliquot is incubated with one of the antigens. After preincubation, a suspension of antigen-bead complexes containing all studied antigens is added to the serum. Specificity of each monoplex is inferred from the comparison with the unpreincubated control. It is believed that the acceptable limit of detection (observed during homologous inhibition test) is 80–120 % [120]. Specificity of a multiplex assay created by Dutch researchers [121] proved to be very high (Table 2). Its diagnostic characteristics were quite good, and throughput capacity was remarkable; detection was rapid, and the amount of samples and antigens required for the analysis was minor.
Another innovative triplex assay was created by American researchers for the detection of Corynebacterium diphtheriae, Clostridium tetani and Haemophilus influenzae type b [122]. The assay incorporates three antigens: tetanus and diphtheria toxoids and the capsular polysaccharide of H. influenzae conjugated to human serum albumin (HbO-HA). It was designed to measure immunogenicity of combined vaccines and was used to reveal that 92.6 % of the studied serum samples had protective levels of IgG antibodies to the tetanus toxoid (≥ 0.1 IU/ML), 80.2 % — to the diphtheria toxoid (≥ 0.1 IU/ml) and 39 % — to the capsular polysaccharide of H. influenzae. Reference measurements were performed with ELISA. The correlation between the two methods was strong (Table 2) both for pre- and postvaccination samples [123].
Of particular interest are multiplex assays for the detection of antibodies to Streptococcus pneumoniae (Table 2). As a rule, such assays incorporate a large variety of antigens ranging from 9 [117] to 23 serotypes of the capsular polysaccharide [119]. Reliable detection of pneumococcal polysaccharides is more complicated resulting in a few assay modifications. First, poly-L-lysin coating is used to ensure better coupling of polysaccharide molecules to the polystyrene beads surface. Second, there is a need to exclude antibodies specific to soluble pneumococcal cell wall polysaccharides and polysaccharide C typical for non-capsular strains of S. pneumoniae, because they do not confer immunity against this pathogen. Therefore, solution used to dilute the serum should contain absorbents — soluble polysaccharides of the cell wall, including polysaccharide C and serotype 22F polysaccharide [117, 122]. The same modifications are introduced to reference ELISAs, improving specificity of the assay and revealing its actual sensitivity.
British researchers designed a multiplex assay for quantification of IgG antibodies to polysaccharides of 9 S. pneumoniae serotypes. The assay was validated using the standard reference serum 89-SF [117]. Then, the assay was run on the serum samples of individuals who had and had not been previously vaccinated against S. pneumoniae. ELISA was used as a reference method. The original multiplex showed the lack of interference between its monoplex components, was sufficiently specific and sensitive, as required by FDA and EMA: the coefficient of variance did not exceed 20 % (Table 2). Besides, the multiplex proved to be stable after being stored for 12 months at +4 °C, showing only a slight decrease in fluorescence intensity by 10–19 %. The assay showed good correlation with ELISA, therefore it might be a better option for monitoring herd immunity against S. pneumoniae since it is time-saving and capable of detecting antibodies to all antigens contained in the vaccine.
An assay for detecting IgG antibodies to polysaccharides of 13 pneumococcal serotypes was developed by a research team from the Netherlands [124]. The assay was developed to measure humoral immune response to a 7-valent conjugate vaccine (PCV-7). Blood serum samples were collected from PCV-7 prevaccinated infants at 2, 3 and 4 months of age and at 11 months of age after booster vaccination. A few nonvaccine polysaccharide serotypes were also incorporated into the assay, revealing elevated levels of IgG antibodies to nonvaccine serotype 6A, resulting from its cross-reactivity with serotype 6B. Geometric mean concentrations of antibodies measured by the original multiplex immunoassay were higher than those measured by standard ELISA, but the coefficient of correlation between the two assays was high (Table 2). Differences between ELISA and MIA results suggest that the established protective threshold of 0.35 μg/ml should be reconsidered for the use in MIA and probably analyzed for each serotype separately [124]. A similar study was conducted a few years earlier in the USA (Table 2) [122]. An immunoassay was designed to simultaneously detect antibodies to 14 pneumococcal polysaccharide serotypes. The assay was run on the serum samples obtained before and after vaccination. Specificity of the assay was tested by conducting homologous and heterologous inhibition tests and turned out to be high for all polysaccharide serotypes except for two closely related serotypes 9N and 9V. However, with some serotypes heterologous inhibition was as high as 50 %. Cross reactivity could not be avoided by preincubation of serum samples with pneumococcal polysaccharide C, but preadsorbtion with polysaccharide serotype 22F considerably reduced the level of heterologous inhibition. The results obtained with ELISA and MIA in this study were highly concordant.
The uniqueness of another assay designed to quantify IgG antibodies to polysaccharides of S. pneumoniae lies in its capacity to simultaneously detect immunoglobulins to 23 polysaccharide serotypes (Table 2) [119]. Apart from polysaccharide serotypes coupled to microsphere beads, the assay incorporated an internal control — microspheres coupled with cell wall polysaccharides of S. pneumoniae and the second control — microspheres coupled with nonvaccine serotype PnPS25 polysaccharide used to evaluate reproducibility of the results, which is critical for pre- and post-vaccination studies. The assay was validated using antipneumococcal standard reference serum 89S-2 (FDA) containing IgG to pneumococcal antigens extracted from the blood serum of individuals vaccinated with a 23-valent vaccine against S. pneumoniae. The ELISA and MIA results were highly correlated, while homologous inhibition was observed for all serotypes except for polysaccharide serotypes 2, 3 ,4, and 5 (no heterologous inhibitions was observed for these serotypes either) [119].
Routine immunization against viral infections such as measles, mumps, rubella and varicella is carried out in the majority of WHO member states. Multiplex assays are also available for measuring immune response to these pathogens. In the study [116] native purified antigens coupled with magnetic microspheres were used (Table 2). The assay was run on 70 samples of human blood serum of vaccinated and unvaccinated individuals. The researchers concluded that their xMAP-based assay was highly specific and sensitive. Besides, LLOQ was calculated for each analyte (Table 3) demonstrating that the x-MAP-based assay was more sensitive to the studied infections than ELISA.
Given their excellent diagnostic characteristics and cost- effectiveness, multiplex assays should be used in large-scale serological studies.
Promising methods for evaluation of immunological memory
Although immunological techniques are becoming increasingly common and provide a wealth of information for serological surveillance, there is growing evidence indicating the need for novel approaches [125]. Current techniques are unable to evaluate the cell-mediated immunity and cannot be tailored to identify infection markers in a particular cell population of interest. They can also simultaneously detect only a few markers. New approaches will have to account for the fundamental properties of immunological memory and molecular features of pathogens. Novel methods can lead to discovery of unknown properties of the immune system or new interpretations of the already known facts. Detection of specific antibodies is the primary method of assessing immunological memory described in the previous sections of the article. In this section we will speak about the limitations of this approach and briefly analyze the new methods employed by epidemiological studies.
Immunological memory is a dynamic parameter of the adaptive immunity. It changes throughout a person’s life, influenced by infections, vaccinations, microbiota composition and lifestyle [125, 126, 127, 128]. First, the ratio of native to immune memory cells decreases with age, resulting in deteriorating immunological memory characteristics [129, 130, 131]. The diversity of adaptive immunity receptors in infancy is similar across the population, but at older age differences become more visible mirroring the events that have occurred in person’s immune system throughout life [127, 128]. Compositions of cell populations, as well as cytokine and immunoglobulin profiles, are different between healthy people [125], shaped by genetic factors, age, sex, gut microbiota, vaccination, lifestyle, environment, season, and circadian rhythms. But little is known about the impact of these factors on the qualitative characteristics of immunity. There is an ongoing active search for markers that can link qualitative and quantitative characteristics of the immune system with the existing pathology or the risk of its development [125].
The article [21] calls for the establishment of a world serology bank and proposes to divide all pathogens into 4 groups. The first group includes acute immunizing antigenically stable pathogens (measles, rubella and smallpox). The second group contains acute immunizing antigenically variable pathogens (flu, invasive bacterial infections, dengue). The third group comprises infections for which antibodies are not protective; among those are tuberculosis, that induce different immune response depending on the infection stage; malaria that triggers production of several antibody types by infected red blood cells; and HIV. The fourth group is the largest and includes infections that do not induce sustained immune response or trigger production of specific antibodies that, however, do not confer immunity against this infection in the future. This group includes intestinal infections and human papillomavirus.
The first group induces sustained humoral response leading to production of protective antibodies. Detected in the sample, these antibodies reliably indicate a past infection or vaccination. With the second group, the presence of antibodies may confer protection against future infection; the antibodies can also be used in vaccine development and pandemic modeling. The existing serological assays can measure the level of resistance to both groups of infections. But they fail to identify whether immunity was induced by vaccination or naturally by a disease [21, 132]. For example, the presence of anti-HBsAg and anti- HBcore antibodies may indicate hepatitis B in the past, while the presence of only anti-HBsAg antibodies indicates that the person has been vaccinated [133]. Some infections may be detected using special immunological markers added to a vaccine [134]. The recombinant vaccine against tuberculosis GamTBvac developed by the authors of this article makes use of the response to the dextran-binding domain participating in deposition onto the dextrane adjuvant [135].
Antibodies produced in response to infections included in the third and fourth groups are not protective against future invasions of the same pathogens. However, they indicate a current or past infection. Therefore, in the absence of reliable markers of established immunity against these groups of pathogens, serological findings can be used to assess vaccination coverage in the population, given that vaccination induces a long-lasting humoral response [21]. Discovery of trustworthy markers of induced immunity would be beneficial for epidemiological surveillance and could inspire development of novel effective vaccines. Vaccines against pulmonary TB, malaria and HIV are still nonexistent partly because no reliable markers of immune protection have been discovered so far. However, there are known cases of immunologically mediated resistance to infections belonging to groups 3 and 4. Here the absence of protective humoral response is explained by the crucial role of cell-mediated immunity [21, 136].
Cell-mediated immunity can be measured using the Interferon Gamma Release Assay (IGRA). This method is based on measuring the level of interferon-gamma (IFN-g) production by lymphocytes of whole blood or the PBMC-fraction (subpopulation of peripheral blood mononuclear cells) exposed to antigens [137]. IFNg levels are determined by ELISA. This approach is widely used to confirm TB diagnosis and detect other infections caused by intracellular pathogens (tularemia, leishmaniasis, cytomegalovirus infection) [138]. Unfortunately, the assay only indicates the presence of infection but does not detect any markers of immunity against it.
A search for the markers of immunity against infection can be aided by flow cytometry and its modification including cell sorting. This method allows analyzing single cells using fluorescently labeled antibodies and cell compartment markers by measuring up to 30 parameters simultaneously in hundreds of thousands and millions of cells [139]. This analysis yields a wealth of reliable information about differentiation and properties of cell subpopulations. The method can be used in routine epidemiological surveillance if the need should arise to search for immunity or sensitivity markers in particular cell populations. Developers should make the protocol for this method as simple as possible. There may be barriers hampering its introduction into routine practice, such as strict requirements for working with live cells. Using cell sorting by modified cytometers, targeted populations of cells can be separated and tested for the presence of immunity markers by different techniques. Sorted cells can be frozen and stored in the biobank until use.
Another promising method is based on estimating the diversity of antibody producing T-lymphocyte [128] and B-lymphocyte receptors by next-generation sequencing (NGS). Diversity of receptors in cell subpopulations provides a wealth of information about patient’s immunological memory, including his/her medical history and the risks of possible infections in the future. State-of-the-art techniques can show what types of antibodies are produced by a particular person and what linear epitopes his/her immunological memory recognizes. Unfortunately, there is no way to identify specificity of all receptors in total. Advances in bioinformatics, cybernetics, artificial intelligence, machine learning, and large-scale studies initiated to investigate correlations between a phenotype and the variability of adaptive immunity receptor loci may provide the answer. Among the disadvantages of NGS are labor-intensive DNA-library preparations, high costs of the analysis, expensive equipment, and the need for highly skilled professionals.
Information obtained with the abovementioned techniques can be of great importance for fundamental immunology. If discovered, novel immunity markers will be adapted for use in simple diagnostic procedures, including multiplex immunoassays [140], IGRA-tests, polymerase chain reaction assays, flow cytometry [139] or mass spectrometry [141].
A proposal for a system of serological surveillance in Russia, expenses and economic rationale
Based on the international expertise and the knowledge of methods for studying herd immunity, we hereby propose a system for serological surveillance in Russia according to the principles mentioned in the first section of this article.
Surveillance should be performed by the Ministry of Healthcare of the Russian Federation, Rospotrebnadzor or another authorized agency capable of providing administrative resources for the interaction with regional healthcare facilities in order to facilitate sample collection. The following hierarchy is proposed.
1. Sample collection. Regional healthcare ministries should be invited to participate in sample collection, since it is in their best interest to have access to reliable epidemiological data; local healthcare facilities should be engaged considering that samples should be obtained from healthy individuals. At this stage participants are recruited, questionnaires filled out, samples collected, processed (serum extraction) and transported to the biobank.
2. Registration, certification and storage of samples in a specialized national serum biobank [23]. The biobank is central [142] to the surveillance system, because it а) accumulates collected samples, aliquotes them and stores for a long period of time under standard conditions; b) automatically processes information about the samples, including the date and location of sample collection, donor personal records, and study results, which considerably reduces the probability of mistakes during testing or result processing, increases reliability of the obtained data, and allows automated receiving and processing of serological statistics.
3. Laboratory screening of the obtained samples for the presence of serological markers using multiplex ELISAs for simultaneous quantification of tens of different markers in a sample, which will reduce costs and time required for the procedure. Authorized laboratories involved are expected to have technical capacities to work with blood samples, which may contain group III and IV biological agents, and to perform culture-independent diagnostic tests for group II biological agents [143, 144].
4. Analytical center for data processing and epidemiological prediction that will also present exhaustive information to public healthcare or other agencies.
According to the international serological surveillance projects, revision of immunization programs should rely on the data obtained from 0.02–0.05 % of the country’s population [5, 20, 87, 88, 90], which is 30–75 thousands of seemingly healthy people in Russia. Study participants should give their informed consent and complete the questionnaire form providing information on their age, sex, socio-economic status, residence, previous vaccinations, chronic infectious diseases, and risk factors. Participants should be motivated by a modest payment and a detailed feedback on their immunity status.
All samples should be stratified into cohorts of at least 500 individuals based on specific epidemiological parameters. Cohorts over 500 individuals ensure accurate estimation (confidence interval) of infection marker carriers proportion with ± 5 % precision at the population level for the seroprevalence of 40–60 %, and with ± 2.5 % precision at the population level for the seroprevalence of 90 % to 100 % (p < 0.05) [145], which is sufficient for the analysis of herd immunity [88]. Stratification should be primarily based on age. Vaccination schedules and age-related changes of the adaptive immunity [146, 147], as well as data obtained in the course of similar projects abroad [148] suggest that stratification into groups should be based on vaccination ages recommended by the national immunization schedule [30]: children before 1, 2, 3, 4, 5, 6, 12, 15 and 18 months of age; before 2 years of age and older until the age of 11 years with 1-year interval; from 11 until 35 years of age with 3-year intervals; from 35 years of age with 5-year intervals (there should be no less than 35 age groups in total each including over 500 individuals). Because mass sample collection in infants below 12 months of age is hampered by technical and ethical difficulties, in the pilot stage of the project children can be stratified based on the established decreed ages specified in Form 6 [28]: before 12 months, before 2 years, with 1-year intervals until the age of 18. This stratification approach will help to estimate the actual number of immune members of the population and to study effectiveness of immunization programs in all age groups.
Of importance is territorial and socio-economic stratification. We believe that the system of healthcare in Russia provides equally effectives services (at least, as far as immunization programs go) for all Russian residents regardless of their area of residence or income. However, this supposition can only be confirmed or disproved by serological surveillance. Besides it should be remembered that endemicity of infection depends on the climate zone and the economic status of the region, thereby affecting local herd immunity. Stratification proposed by the Russian Longitudinal Monitoring Survey (RLMS) conducted between 1992 and 1998 [149] appears to be quite adequate for our purposes. The entire territory of Russia was divided into 10 geographical and 10 life quality strata. To ensure representativity of the sample (individuals registered with a local healthcare facility and households), the method proposed in the ESSE-RF study (2012–2014) [150] was used, similar to that of NHANES (National Health and Nutrition Examination Survey [151]). The authors of these works claim that their method is well-established and suitable for big and diverse populations.
The range of serological markers should be defined. We recommend including in the surveillance all vaccine-preventable infections listed in the national immunization schedule. This list contains 23 infections. Because of seasonal mutations, a separate method should be elaborated for flu strains [152, 153] and tuberculosis, because the presence of IgG antibodies in blood does not indicate protection against these infections [135]. We also recommend using ELISA as a diagnostic tool.
Ideally, cross-sectional studies [25] of herd immunity at a particular time point at the population level should be conducted on a regular basis, once every 6 or 7 years, because this interval is sufficient to establish a stable immunity to vaccine- preventable infections or infections in general [31, 146, 154] and indicates succession of “immune generations”.
According to our estimates, one round of serological surveillance in 10 pilot regions (30,000 samples, 30 antibodies) will cost 300 million rubles, including 62 million rubles for sample collection (payments to participants and medical personnel involved, expenses on consumables and paper work); 23 million rubles for modernization of regional centers for sample collection and preparation; 16 million rubles for transportation; 99 million rubles for multiplex immunoassays; 100 million rubles for expenses on analytical centers (biobank stewardship, equipment, paychecks, administrative costs). We believe that long-term results of serological surveillance will justify expenditures of the state.
Economic effect from serological surveillance can be expected to emerge after additional vaccination of susceptible population groups as the economic burden caused by consequences of vaccine-preventable diseases will decrease. A method to assess this type of burden was proposed by the experts of the Central Research Institute of Epidemiology [155, 156]. According to [34, 50], the state loses a total of 6 billion rubles as a result of infections that can be prevented by vaccination (pertussis, measles, mumps, tetanus, diphtheria, rubella, acute hepatitis B, hepatitis A, brucellosis, tularemia, typhoid fever, rabies, hemorrhagic fevers, including tick-borne encephalitis and yellow fever). Strong herd immunity against these infections aided by additional vaccination of susceptible population groups will be economically beneficial. In this light, 300 million rubles appear to be a reasonable cost, even if additional vaccinations will be necessary. The ratio of expenses to the long-term beneficial economic effect is estimated between 1 : 5.7 and 1 : 14.4 [157], rendering vaccination programs economically justifiable.
CONCLUSION
Increasingly intricate immunization schedules, migration, ageing, and vaccine hesitancy call for the need to introduce a system for population-wide serological surveillance capable of estimating herd immunity against infections and identify susceptible population groups. This system will help to update statistics on herd immunity in different social groups and regions, revise the national immunization schedule, calculate the amount of vaccines, diagnostic tools and efforts required for protecting the nation against infections, as described in [23].
Current methods applied in the studies of herd immunity are not comprehensive and cannot be used as reliable prognostic tools: data on vaccination coverage and infection incidence are often unrepresentative of the entire country’s population and the serological studies are too few, limited to a certain area and contradict the basic principles of serosurveillance (adequate coverage, continuity and homogeneity of data).
The effective system for serological surveillance should include collection of samples representative of the studied population, establishing biobanks of serum, DNA, RNA and cell samples, development of screening tests for the immunological memory qualitative assessment and epidemiological modeling based on the data on herd immunity. A surveillance system is crucial for developing a wise vaccination strategy and ensuring protection against biological threats guaranteed by the Constitution of the Russian Federation.