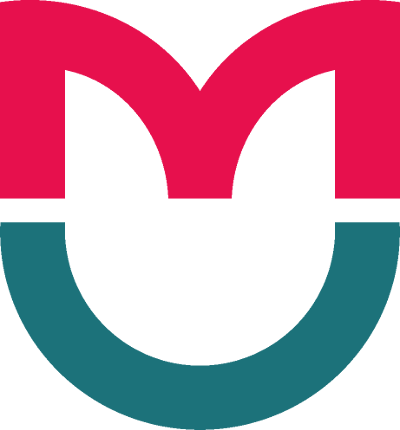
ORIGINAL RESEARCH
Targeted sequencing in patients with clinically diagnosed hereditary lipid metabolism disorder and acute coronary syndrome
1 Central State Medical Academy of the Department of Presidential Affairs of the Russian Federation, Moscow, Russia
2 City Clinical Hospital № 51, Moscow, Russia
3 Kharkevich Institute for Information Transmission Problems, RAS, Moscow
4 ReadSense OOO, Troitsk Center for Nanotechnologies of Rusnano Foundation for Nanotechnology Infrastructure and Educational Projects, Moscow
5 Kazan State Medical University, Kazan
6 National Medical Research Center for Cardiology, Moscow, Russia
7 Stavropol State Medical University, Stavropol
Correspondence should be addressed: Anastasia О. Averkova
Marshala Timoshenko 19, b. 1A, Moscow, 121359; ur.liam@keva
One of the known causes of premature coronary artery disease (CAD) is familial hypercholesterolemia (FH), a hereditary lipid metabolism disorder that increases the risk of developing cardiovascular disease twentyfold [1]. It is the most common monogenic autosomal-dominant lipid metabolism disorder characterized by elevated low-density lipoprotein (LDL) cholesterol. The majority (60–80%) of patients with clinically established heterozygous FH carry mutations in the gene coding for the LDL receptor (LDLR); the prevalence of each implicated mutation varies geographically. These mutant variants result in fewer LDL receptor molecules on the cell surface or their decreased activity. The LDLR gene is located on the short arm of chromosome 19 and consists of 18 exons transcribed and translated into 5 LDLR-forming domains [2]. About 5–10% patients with heterozygous FH have mutations in the APOB gene that are phenotypically expressed as a less pronounced elevation of cholesterol levels; these mutant variants are more common in Central Europe than in other regions [3]. The defect in the ApoB100 protein, a component of LDL particles, prevents it from binding to LDLRs. APOB has been mapped to chromosome 2p and comprises 29 exons [2]. Gain-of-function (GOF) mutations of the proprotein convertase subtilisin/kexin type 9 gene (PCSK9) are another cause of autosomal-dominant FH accounting for < 1% of all FH cases in the majority of the studied populations. GOF variants lead to rapid LDLR internalization and downregulate the number of LDLR molecules. The PCSK9 gene sits on the short arm of chromosome 1p32 and is constituted by 12 exons and 11 introns [4, 5]. Mutations in other genes involved in lipid metabolism rarely cause FH [3]. Some patients carry two mutations in the genes associated with FH. Unfortunately, in 20 to 40% of patients with clinically confirmed FH the causative mutation cannot be identified.
The aim of this work was to determine the spectrum of pathogenic variants involved in lipid metabolism in patients with acute coronary syndrome (ACS) and a clinical diagnosis of FH by targeted sequencing.
METHODS
For this study we used the samples obtained from 2,081 patients with ACS who had participated in two multicenter observational studies carried out in 4 centers in Moscow, Kazan and Stavropol. The patients had been recruited in 2004 through 2007 and in 2014 through 2016. The study protocol was described in [6]. The study was approved by the Ethics Committee of the Medical Educational Research Center of the Department of Presidential Affairs of the Russian Federation (Protocol 14/14 dated October 20, 2014).
We selected 326 patients from the 1st and 374 patients from the 2nd recruitment periods. Over the first recruitment period the inclusion criteria were as follows: premature ACS (at ≤ 55 years of age in men and at ≤ 60 years in women); ACS with an onset at least 10 days before index admission to hospital; FH diagnosis based on the Dutch Lipid Clinic Network score and Simon Broome criteria [7, 8]; written informed consent to participate. During the second recruitment period the inclusion criteria were the same but additionally those patients were included who had indications for percutaneous coronary intervention (PCI), regardless of whether the latter was eventually performed or not.
The diagnosis of FH was based on the Dutch Lipid Clinic Network (DLCN) score and Simon Broome criteria. Generally, the DLCN set of criteria accounts for the patient’s family history, early CAD onset in the patient, physical examination (tendinous xanthomas, corneal arcus), and LDL levels. Patients who score more than 8 points are concluded to have definite FH; 6–8 points, probable FH; 3–5 points, possible FH; less than 3 points, unlikely FH [7]. The Simon Broome criteria account for the total cholesterol and LDL levels, the age of the patient and their kinship to a relative who also has elevated LDL and cholesterol. In the clinical setting, only possible FH can be detected; the diagnosis of definite FH cannot be established without identifying its causative mutation [8].
Molecular genetic screening was performed on the samples of 38 patients selected using the following algorithm. Patients enrolled during the first recruitment period were eligible for the genetic screening if they scored ≥ 5 points on the DLCN scale or scored 4 points on the DLCN scale and also had FH according to the Simon Broome criteria (a total of 10 people). Patients recruited during the second period were eligible if they scored ≥ 5 points on the DLCN scale or met the Simon Broome criteria and had a family history of cardiovascular disorders (a total of 24 individuals). This second sample also included 4 patients who did not have premature ACS at the time of hospital admission but still had an episode of early CAD manifestation according to their medical history (≥ 6 points on the DLCN scale or 5 points on the DLCN scale + a family history of cardiovascular disorders).
Sequencing was carried out in the ReadSense laboratory (Moscow). DNA was isolated from blood samples using the K-Sorb DNA isolation kit (Syntol; Russia) and spin columns. Preparation of DNA libraries was aided by the NEB Next Ultra kit (NEB; USA). Capture-based enrichment of target protein-coding regions was done using the NimbleGen panel of biotinylated probes (Roche; USA). Quality of the enriched DNA libraries was assessed by capillary gel electrophoresis on BioAnalyzer 2100 (Agilent; USA) and Qubit fluorometer (Invitrogen; USA). The libraries were sequenced using the 300-cycle v2 MiSeq reagent kit on the Illumina MiSeq next-generation sequencer (Illumina; USA) set up according to the manufacturer’s recommendations.
First, 3 genes associated with FH were sequenced, including LDLR, APOB, and PCSK9. Then, if no relevant variants were detected, the panel was expanded to cover the entire range of genes involved in lipid metabolism: APOA1, APOA5, APOC2, APOE, APOC3, ABCA1, ABCG1, ABCG5, ABCG8, ANGPTL3, CEL, CH25H, CPT2, CYP2D6, CYP3A4, CYP3A5, GPD1, GPIHBP1, INSIG2, LCAT, LDLRAP1, LIPA, LMF1, LPA, LPL, MTTP, NPC1L1, PNPLA2, PPARA, and SAR1B.
A number of steps were taken to evaluate the quality of the enrichment panel. The reads obtained for each sample were mapped to the reference human genome (GRCh37.p13 hg19). To understand whether sequencing coverage was sufficient, we analyzed the nucleotides used for probe synthesis that were covered at a minimum of 20x. Once detected, the target mutations were annotated using HGMD, COSMIC, ClinVar, 1000 GenomesProject, dbSNP, and ExAC databases. Conservation of substitutions was estimated by a number of prediction tools (POLYPHEN, SIFT, MUTATION TASTER, FATHMM, CADD, DANN, and EIGEN). Mutant variants were annotated as recommended by ACMG [9].
RESULTS
All patients who carried variants of the studied genes were heterozygous. Variants of the three “classic” genes implicated in FH were detected in 11 patients.
Among them were 3 pathogenic and likely pathogenic variants of the LDLR gene and one variant with uncertain clinical significance. Of them, one deleterious mutation (p.Val273_Cys313del) was previously undescribed. It is an in-frame deletion in exon 6 resulting in a missing class A7 domain at the N-terminus of the LDLR [10]. This mutation belongs to class 3 of LDLR mutations leading to the production of a defective receptor that cannot bind to LDL. A similar mutation g.11218068_11218190del was described by Usifo et al. [11]. Another mutation detected in our sample yet previously undescribed (p.Ala776Ser) is located in exon 16 and has uncertain significance. Such rare missense mutations observed in the general population are normally classified as non-pathogenic. However, we cannot completely rule out their contribution to hypercholesterolemia [12]. The likely pathogenic mutation p.Gly20Arg in the LDLR gene occurred in two patients. The pathogenic mutation p.Glu208Lys was detected in one patient.
Pathogenic and likely pathogenic APOB variants were observed in two patients. Two more patients carried mutant APOB variants of uncertain significance. The population frequency of all APOB mutations identified in our study is less than 1%.
Two patients carried previously undescribed PCSK9 mutations of uncertain significance. One of them (p.Glu612Lys) is located in exon 11. PCSK9 mutations resulting in the modification of the functional cysteine- and histidine-rich C-terminal domain are known to be associated with FH. Specifically, these genetic variants add to the severity of hypercholesterolemic phenotype in patients who also have mutations in the LDLR gene (our patient also had a likely pathogenic mutation p.Gly20Arg in this gene) [13]. The second PCSK9 mutation c.*415G>A is located in exon 12. This region is untranslatable and contains elements responsible for PCSK9 expression. However, it is impossible to predict the functional consequences of this genetic variation.
Patients with clinically established FH had mutations in other genes involved in lipid metabolism, such as APOE, which turned to be mutant in two participants. One of those patients had a previously undescribed and likely pathogenic mutation (p.Arg160His) located in exon 4. This genomic region is involved in the binding of the APOE lipoprotein to LDLR. Its mutant variants have been shown to be associated with autosomal-dominant familial dysbetalipoproteinemia [14]. Four participants carried the ε4 allele of the APOE gene resulting in the atherogenic isoform of apolipoprotein E (one of those patients also had the p.Ala4002Val variant of uncertain significance in the APOB gene).
Three patients had ABCA1 mutations; of them one was pathogenic and two likely pathogenic implicated in low HDL. Two patients were found to have ABCG8 mutations thought to be associated with sitosterolemia: one was pathogenic and another one of uncertain significance [15]. One patient had a likely pathogenic mutation in the ABCG5 gene; one patient carried a possibly pathogenic mutation in the LPL gene; one bore a pathogenic mutation in ANGPTL3; another, a mutation of uncertain significance in the MTTP gene.
Thus, of 38 patients with clinically established FH 24 (63.2%) carried variants that could be phenotypically expressed as FH or early CAD. Five of the identified variants were previously undescribed.
The results of our study are presented in the table below and in figure.
DISCUSSION
The prevalence of FH in the general population is 0.2–0.5%, but in patients with ACS it can be as high as 8%. Therefore, its accurate diagnosis in this particular subpopulation is very important as it enables adequate lipid-lowering therapy and initiate cascade screening [16, 17]. In Russia the first steps are being taken in investigating the prevalence of mutations implicated in FH [18]. However, we already know about the extreme genetic heterogeneity and the absence of the founder effect demonstrated by a research team from Saint Petersburg [19]. They screened a group of patients with FH who did not have ACS and found no carriers of mutant APOB. Another research work conducted in Northwest part of Russia also showed the absence of APOB mutations in the studied subpopulation [20]. In our sample of patients with premature ACS, LDLR and APOB mutant variants were equally represented (4 mutant variants of LDLR of which 3 were pathogenic or likely pathogenic and one was present in two patients; 4 mutant variants of APOB of which two were pathogenic or likely pathogenic). Interestingly, there were 2 patients in our sample with the FH phenotype who carried mutations of uncertain significance in the PCSK9 gene. So far, only a few cases of PCSK9 variants have been described in the Russian population [21]. All known deleterious mutations of PCSK9 are very rare and have an allelic frequency of < 0.1% in the general population [22]. Distribution of SNPs in the general population only mildly affecting cholesterol metabolism demonstrates a balance between the alleles that up- and downregulate the levels of LDL. In the individuals at the extreme ends of this spectrum, alleles that increase LDL levels are hereditarily prevalent; together these alleles can cause elevated LDL characteristic of heterozygous FH. One of the most typical and well-studied examples of such single nucleotide polymorphisms is the ε2/ε3/ε4 polymorphism of the APOE gene. The risk of developing CAD increases in the carriers of the ε4 allele manifold [23, 24]. The ε4 allele of p.Cys130Arg has been shown to be associated with FH in the Russian population [25], which is also confirmed by the present study that has identified this polymorphism in 4 patients. Importantly, the actual frequency of rare variants in other genes involved in lipid metabolism, including APOE, ABCA1, ABCG5, ABCG8, LPL, ANGPTL3, and MTTP, may be different from what we believe in the subpopulation of Russian patients with ACS and FH. Patient 1 (see the Table) carried a previously undescribed but definitely pathogenic mutation p.Val273_Cys313del in the LDLR gene [11]. The proband’s daughter also had elevated total cholesterol and underwent a cascade screening procedure, which found no presence of the pathogenic variant in her genome. To sum up, of 38 patients 14 (36.8%) had pathogenic or likely pathogenic variants and 10 (26.3%) carried variants of uncertain significance. The literature does not provide us with the data on the frequency of FH-associated variants in patients with premature ACS. However, the authors who conducted target sequencing in 104 patients with a clinical diagnosis of FH revealed the presence of pathogenic variants both in the “classic” genes, including LDLR, APOB, and PCSK9, and rare genes involved in lipid metabolism in 67% of the participants with definite FH [26].
CONCLUSIONS
The use of clinical diagnostic tests followed by targeted sequencing helps to identify pathogenic variants not only in the “classic” genes implicated in FH but also in the “rare” genes that might be associated with the FH phenotype in patients with ACS and FH.