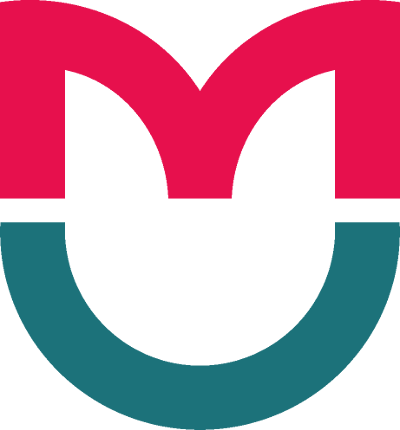
ORIGINAL RESEARCH
EEG coherence in children with cerebral palsy against the background of rehabilitation employing a brain-computer-hand exoskeleton neurointerface
1 V.I. Vernadsky Crimean Federal University, Simferopol, Russia
2 Research Institute of Children's Balneology, Physiotherapy and Medical Rehabilitation, Yevpatoria, Russia
Correspondence should be addressed: Vladimir B. Pavlenko
pr. Vernadskogo, 4, Simferopol, 295007, Russia; moc.liamg@55vapv
Funding: The study was supported by the Russian Science Foundation and the Republic of Crimea grant No. 22-15-20035, https://rscf.ru/en/project/22-15-20035/
Author contribution: Pavlenko VB, Chuyan EN — study planning, data analysis and interpretation, manuscript drafting; Vlasenko SV — study planning, data collection, data analysis and interpretation, manuscript drafting; Pavlenko DV — data collection, data analysis and interpretation, manuscript drafting; Birukova EA, Orekhova LS — data collection, data analysis, manuscript drafting.
Compliance with ethical standards: the study was approved by the V.I. Vernandsky Crimean Federal University ethics committee (Minutes #1 of January 25, 2022). Parents submitted signed informed consent forms allowing their children to participate in the experiment.
Cerebral palsy is a group of persistent disorders of movement and posture [1, 2]. However, brain plasticity potentiates treatment of this disease. Actively advanced methods of neurorehabilitation revolve around plasticity, when patients are tasked with multiple repetitions of certain movements or imagining making them. The latter, motor imagery, was noted to even more taxing cognitively than movements per se, thus activating the regenerative processes of the nervous tissue [3]. One of the approaches to neurorehabilitation relies on noninvasive brain-computer interfaces (NIBCI) combined with a hand exoskeleton [4]. In children with cerebral palsy, this approach helped improve motor functions of the upper limbs [5, 6] and motor realization of speech [7].
In the context of rehabilitation of cerebral palsy patients, functional state of the brain and dynamics of neuroplasticity are assessed by analyzing the amplitude of sensorimotor rhythms recorded on an electroencephalogram (EEG) [6, 8, 9]. Another promising approach involves study of the specifics of structural and functional connectivity of neural networks of the brain during treatment. Structural connectivity is defined as a set of neural pathways representing the established anatomical imagery [10]. In patients with cerebral palsy, diffusion tensor magnetic resonance imaging (DTI) revealed abnormalities in the corticospinal tract and the somatosensory thalamocortical projections. Additionally, structural integrity of the commissural and associative pathways of the large hemispheres were found altered [11]. Functional connectivity is defined as a set of interconnections between dynamic activities of neurons in different regions of the brain. The repertoire of functional configurations reflects the underlying anatomical connections. Functional MRI (fMRI) and electrophysiological methods, such as electro- and magnetoencephalography, enable studies in this area [10].
Analysis of the fMRI data allows assessing functional connectivity by calculating correlations between the indicators of activity of brain regions at rest. Children with cerebral palsy were found to be widely susceptible to functional connectivity disorders; moreover, depending on the form of the disease and the choice of the analyzed areas, both reduced and increased values of this indicator (compared to the control group) were registered [11]. Of particular interest is the revealed deterioration of the relationship between frontal and parietal regions, which can promote motor and cognitive disorders in cerebral palsy [12].
However, the results yielded by resting-state fMRI are not a reliable indicator of impaired motor abilities in cerebral palsy [2]. Moreover, fMRI does not offer sufficient temporal resolution, since it measures the metabolic (secondary) response to neuronal activation [13]. EEG recordings have high temporal resolution, thus, they are important for describing spatial and temporal dynamic interdependencies of neural activation and connectivity. The process of description includes an analysis of spectral coherence, which is reflects paired cross-correlation in the time domain [10]. Spectral coherence is usually calculated in the bands of EEG rhythms. Its coefficient varies from zero to one depending on the degree of synchronization of the activity of functionally related regions of the cortex [13, 14].
To date, there is only a few works that analyze EEG coherence in children with cerebral palsy. Initially, the coherence of EEG rhythms in such patients was measured only at rest. In children with diplegia (bilateral brain lesions), interhemispheric coherence in the occipital region had a lower α rhythm value than registered in the control group. In the bands of δ, θ, and β rhythms, coherence was elevated for inter- and intrahemispheric lead pairs, which was interpreted as a reflection of the compensatory processes [15]. In children with hemiparesis, interhemispheric coherence values were high in the bands of δ and θ rhythms, and low — in the bands of α and β rhythms [16]. The values of coherence in the affected hemisphere were lower than in the relatively intact hemisphere, which researchers attributed to the consequences of local disruptions in the neocortex and subcortical white matter.
A later study involved patients with unilateral cerebral palsy and investigated interhemispheric coherence (as registered with EEG) above the central region of the cerebral cortex at rest and when extending wrist [17]. In the α rhythm band, the coherence at rest was reduced, and the higher the degree of motor function disorders, the lower the level thereof. Another recent study, which involved children with unilateral cerebral palsy, looked into the intrahemispheric coherence between central and frontal regions of the neocortex in the µ rhythm band when standing and walking calmly [18]. As opposed to the results of the aforementioned studies [14, 15], in a standing position, compared to the control group, patients had higher coherence in the damaged hemisphere and lower in the intact one.
EEG-based coherence was assessed as indicator of neuroplasticity in the context of rehabilitation of stroke patients. The researchers have underscored the importance of its dynamics as a reflection of the process of recovery of neocortical functions [19]. However, to our knowledge, there was only one study that considered EEG-based coherence data in patients with cerebral palsy during treatment. That work involved children with impaired mobility of the lower extremities, and sought to establish functional connectivity of cortical regions in a series of motor imagery sessions [20]. When patients imagined that they moved a limb up, the classifier program detected changes in the EEG pattern and displayed images on the screen that served as visual feedback. The rehabilitation course pushed up the EEG clustering coefficient in the 8–15 Hz band, which was interpreted as an improvement of the neocortex's network capacities. However, data on the results of motor rehabilitation are not presented in the work.
In this connection, this study aimed to analyze the changes of coherence in the θ-, α- and β rhythm bands as recorded in the EEG in the context of balneotherapy combined with a course of neurorehabilitation (enabled by the NIBCI — hand exoskeleton complex) prescribed to children with cerebral palsy, and to investigate the relationship of these changes with the indicators of motor activity.
METHODS
Sample characteristics
The study was conducted in 2022–2023 at the Health and Rehabilitation Technologies Center of V.I. Vernandsky Crimean Federal University, and at Gelilovich Chaika Health Center for Children and Children with Parents. The participants were 30 children aged 6-15 years, undergoing treatment at the health center combined with a course of neurorehabilitation employing NIBCI. The inclusion criteria were: diagnosed cerebral palsy (as per ICD 10); spastic diplegia with motor functions development at levels I-III as per the Gross Motor Function Classification System for Cerebral Palsy (GMFCS). The exclusion criteria were: motor functions development level above III as per GMFCS; aphasic disorders; drug-induced uncorrectable epilepsy; visual impairments disallowing distinguishing instructions on the screen; moderate, severe and profound mental retardation (F71–73 as per ICD 10).
Ultimately, we excluded the children whose EEG contained many artifacts due to excessive motor activity, and had the sample of 23 children (7 girls, 16 boys) aged 7–15 years, with 15 of them suffering mostly right hand movement disorders, 6 — movement disorders of the left hand, and two with such disorders equally pronounced for both hands.
Assessment of indicators of motor functions
To assess the motor functions of the patients, we used the Barthel index, which allows measuring performance in activities of daily living based in a questionnaire [21]. The higher the performance indicators, the higher the index values (range from 0 to 100 points). The Barthel index is applicable to both adult and infant cerebral palsy patients [22]. Children that participated in the study took the Barthel index test on the second day after admission to the health center. They have filled the questionnaire uncovering their motor functions performance the second time once the treatment course was over, on the 14th or 15th day of their stay in the health center.
Rehabilitation procedures
In the health center, the treatment included daily aero- and heliotherapy, hydrokinesiotherapy in thermal mineral water (duration — 10–15 minutes), physical therapy, massage of paretic muscles, electrical stimulation of muscles antagonistic to paretic. Every other day the patients underwent peloid treatment. Botulinum therapy was not used in the health center. Neurorehabilitation employed the brain – computer – hand exoskeleton complex, with the letter being Exokist-2 (Exoplast, Russia; Reg. Cert. RZN 2018/7681). The non-invasive braincomputer interface enabled identification of EEG-registered patterns emerging as part of imagined extension of the hand. A classifier software was used for the purpose; it generates visual feedback and hand exoskeleton movement commands.
The EEG recordings were monopolar, taken with the help of the BMM-52 electroencephalograph (NVX-52 amplifier, Moscow, Zelenograd, MKS) with 32 leads. The electrodes were arranged under the incomplete 10-10 system. An averaged electrode was used as a reference. During recording, the cutoff frequencies for the high and low pass filters were 4 and 30 Hz, respectively. The digitization frequency of EEG signals was 500 Hz.
During the neurorehabilitation, patients sat in a chair in front of a monitor that showed them visual instructions. On their hands, they were wearing mittens of the exoskeleton. In the center of the screen there was a rounded white mark, upon which the patients could fix their gaze; around the mark, there were three arrows, which gave instructions by changing color. The commands were as follows: relax, imagine extension of the left or right hand. To create the specific kinesthetic image, children were given the following instructions: "Imagine that you have a small ball in your hand. You open the hand and drop it. Feel this movement." When the patient successfully executed the command and the classifier program detected certain EEG patterns, the gaze-fixing mark turned green, and the exoskeleton performed the respective movement, i.e., the patient's hand extended passively. Thus was the combined visual and kinesthetic feedback signal generated. The neurorehabilitation course began on the third day of stay at the health center. The patients underwent 10 sessions (daily, except for Sunday) as follows: three takes per session, each take 8 minutes long, at least 5 minutes of rest between the takes. Within a session, the movement imagery task for each hand was repeated 24 times. During the first session, the share of correct responses that triggered the exoskeleton (i.e., were interpreted by the classifier software as correct) ranged from
0.18–0.66, and by the end of the course these figures were 0.30–0.84. Other details of the rehabilitation technique were described earlier [7].
To assess the rearrangement of the cortical regions' interconnections following neurorehabilitation, we studied the EEG to analyze coherence in θ, α, β1 and β2 rhythms in the bands 4–8, 8–13, 14–20 and 20-30 Hz, respectively. Sections of EEG recordings with the amplitude exceeding 250 µV, and those with a large number of artifacts, were excluded. We analyzed at least 10 artifact-free epochs, with the minimum overall duration of EEG being 50 seconds. Since movements proper and imagery thereof rely in the interactions within the frontoparietal network [3], the intrahemispheric coherence of biopotentials was calculated for ten pairs of leads of the frontal, central and parietal regions of the left (F3–C3, FS3–C3, C3– CP3, C3–P3, F3–P3) and right (F4–C4, FS4–C4, C4–CP4, C4–P4, F4–P4) hemispheres; as for the interhemispheric coherence, it was calculated for for five pairs (F3–F4, FS3– FS4, C3–C4, CP3–CP4, P3–P4). For the purpose, we used the Neurosoft software (Russia), which is part of the NeuronSpektr-5 electroencephalograph kit.
The values of the coherence coefficients were transformed using the natural logarithm function. Numerical values beyond 3 σ were discarded. As a result, distribution of the coherence values was close to normal, which allowed analyzing them using the techniques of parametric statistics.
Statistical data processing
We used STATISTICA v.12 software (StatSoft Inc.; USA) to perform statistical analysis of the data. For each of the rhythms, the coherence values derived from the EEG were subjected to ANOVA with repeated measurements, TRAINING (first and tenth sessions) and PAIRS (10 or 5 pairs of leads) factors. The linear contrast method was used to assess changes in coherence in each of the lead pairs. Since baseline values of the correlation coefficients, which were not subjected to logarithmization, may also be of interest, and their distribution, as shown by the Shapiro-Wilk test, was abnormal, the specific indicators are given in the text as median and interquartile range Me [Q1; Q3]. The distribution of the Barthel index was also abnormal, therefore, we applied a similar approach to the presentation of the respective statistical data. To assess the differences in the Barthel index values before and after complex treatment, we used the Wilcoxon test; for correlations, the test of choice was Spearman's. The differences and correlation coefficients were considered significant at p < 0.05.
RESULTS
Motor functions indicators before and after the rehabilitation
Before the treatment combined with neurorehabilitation, the mean Barthel index value was was 70 [60; 85] points, after ― 77.5 [74.0; 95.0] points (the differences are significant at p < 0.001). Thus, the children's capacity for independent movement and self-service have increased significantly.
Coherence dynamics following the rehabilitation
Applied to the changes of intrahemispheric coherence associated with the left hand movement imagining, ANOVA revealed an effect of the TRAINING factor in the α rhythm band: F 1, 14 = 7.21; p = 0.02. Compared to the first session, the level of coherence registered during the last session has increased, especially in the right hemisphere, which was contralateral to the imagined movement. The linear contrast method revealed significant differences in the C4–CP4 pair, where the value has grown significantly at p = 0.004 (figureA; red line). The value of the coherence coefficient in this pair increased from 0.38 [0.36; 0.45] to 0.43 [0.39; 0.47].
As detected in the θ rhythm's band, imagining movements of the right hand was affected by the interaction of the TRAINING and PAIR factors: F 9, 90 = 2.37; p = 0.02 in the range of the EEG rhythm. In this band, the level of coherence decreased in the pairs of the right, ipsilateral hemisphere. The linear contrast method confirmed the differences in the C4–FC4 pair, where the decrease in coherence was significant at p = 0.01 (figureB; green line). In this pair, the values of the coherence coefficient decreased from 0.41 [0.36; 0.42] to 0.36 [0.33; 0.38]. Also, TRAINING factor was revealed to affect imagining of the right hand movements in the β1 rhythm's band: F 1, 7 = 24,091 at p = 0.002. In this band, the level of coherence was also decreasing. The linear contrast method confirmed significant differences in the contralateral hemisphere's pair C3–CP3 at p = 0.03, and F4–P4 of the ipsilateral hemisphere at p = 0.01 (figureB; blue lines). In these pairs, the values of the coherence coefficients decreased from 0.39 [0.37; 0.42] to 0.37 [0.34; 0.41] and from 0.40 [0.37; 0.43] to 0.38 [0.36; 0.40], respectively.
In the β2 rhythm band, the analysis of dynamics of the intrahemispheric coherence revealed no significant effects of the TRAINING factor nor its interaction with the PAIR factor.
The analysis of the dynamics of interhemispheric coherence in the bands of the θ, α, β1 and β2 EEG rhythms as associated with imagining movements of the left and right hands, as well as analysis of the intra- and interhemispheric coherence in a relaxed state, revealed no effect of the TRAINING factor and its interaction with the PAIR factor.
The relationship between coherence and motor functions indicators
To assess the relationship between coherence and motor functions, we took the values registered with the help of EEG during the final session of the neurorehabilitation course, and incorporated the Barthel index values that reflect the motor functions of children at the end of the course. Only those pairs of leads that yielded significant changes were selected for the analysis (shown above). We have established a relationship between coherence of the α rhythm in the C4–CP4 pair and the value of the Barthel index (r = 0.52; p = 0.04): the higher the said coherence in the given pair of right hemisphere leads that is associated with imagining movements of the left hand, the better the patient's capacity to move and self-care. There were no significant correlations identified for other EEG-recorded bands.
DISCUSSION
After the course of neurorehabilitation, we identified significant changes in the intrahemispheric coherence of the θ, α and β1 rhythms in individual links of the frontoparietal circuits, which were accompanied by improvements in the patients' motor functions. During the final session, imagining movements of the left hand was associated with a level of the α rhythm coherence significantly higher than registered at the beginning of the rehabilitation. What can reinforcement of interconnections in the band of this rhythm signify? Some researchers suggest that synchronized EEG oscillations enable rapid and selective interaction between regions of the brain [23-25]. In their opinion, it is the α rhythm that supports largescale synchronization, since α waves are ubiquitous in the cerebral cortex, and they reflect the alternation of periods of inhibition and excitation. Synchronization of such periods in different regions of the brain enhances their interactions, which, inter alia, is a precondition for functioning of the frontoparietal network. It is important to note that the activity in the α rhythm band that we recorded in the central and adjacent leads corresponds to the low-frequency component of the µ rhythm generated in these areas of the neocortex. The dynamics of the µ rhythm reflects the processes of realization or imagining of movements; it is a recommended electrophysiological marker of plasticity of the cortical sensorimotor system [8]. In our study, contrasting revealed significance of growth of the α rhythm coherence in the C4–CP4 pair (figureA) located above the postcentral sensorimotor cortex and the inferior parietal lobule [26]. In healthy subjects, these regions were activated during movement imagery tasks and were loci of neural networks the coherence of which correlated with the results of such tasks [27].
It is necessary to add that kinesthetic imagery of movements requires actualization of information in the child's memory. Previous studies have reported that phase interactions in the α rhythm band detected in the central and parietal cortex underlie the downward modulation of local oscillation amplitudes in sensory regions, supporting the functions of attention and memory [23]. Thus, the growth of EEG coherence in the α rhythm band improves interaction of brain regions that actualize movement images in memory and keep focus on them. As a result, kinesthetic imagery becomes more successful, as shown by the increased share of correct responses registered by the classifier software. Imagining movements, people activate same neural structures that participate in the actual realization thereof [28, 29], thus, successful kinesthetic imagery enhances the processes of neuroplasticity of cortical and subcortical structures. Consequently, coordination of real limb movements improves, which significantly boosts the Barthel index value.
We have also revealed a decrease in the level of coherence of the θ and β1 rhythms associated with the right hand movement imagining (final session compared to the first one). Previously, children with cerebral palsy have shown elevated coherence of the θ rhythm [15, 16], which was interpreted as an action by the compensatory mechanism triggered by a disruption of functional connectivity in the α rhythm band. Thus, a decrease in the coherence of the θ rhythm can be regarded as a manifestation of certain positive changes in the functioning of the neocortex.
The decrease in the coherence of the β1 rhythm may be caused by the specifics of the task. The band of this rhythm corresponds to the high-frequency component of the µ rhythm. Its dynamics reflects the activity of the primary motor cortex in the process of actual realization of movements [8]. At our sessions, children only needed to imagine, but not realize a movement, which involved mainly the sensorimotor areas of the neocortex. It should be added that the coherence of the β rhythm increases when the movements performed require a higher level of control [30]. Obviously, the task that involved kinesthetic imagery did not call for this sort of control. It can be assumed that during the first session, it was harder for the participants to imagine movements, which necessitated additional activation of the primary motor area. Further on, imagining required less effort, and the activation of the cortex was more local.
In conclusion, we believe it is necessary to note the following. As previously shown, dysfunction of the frontoparietal network in children with cerebral palsy promotes motor and cognitive impairments [12]. The changes in the coherence of EEGregistered rhythms in the links of this network that we have identified can be regarded as indicators of positive changes in the functional state of the neocortex of patients.
The robustness of this study was limited by the lack of a control group that would have included children with cerebral palsy; this group could have had the EEG taken under similar conditions (kinesthetic imagery), but would not have had the neurorehabilitation course. The time of stay at the health center (21 days) disallowed designing this effort as a crossover study, which would be the most appropriate and ethical approach in this case.
CONCLUSIONS
Combination of balneotherapy and a course enabled by the brain-computer-hand exoskeleton interface yielded a significant increase of the Barthel index in children with cerebral palsy (p < 0.001), indicating an improvement in mobility and self-service capabilities. We have identified a statistically significant (p < 0.05) change in the coherence of intrahemispheric connections in the bands of θ, α and β1 rhythms. The basis of such changes may be reinforcement of the plasticity processes in the neural networks of the neocortex, which control planning, imagining, and execution of complex movements. The data from this study can be used to assess the functional state of brain of the patients in the context of rehabilitation and in development of the new methods for correcting motor function disruptions in children with cerebral palsy. It seems feasible to further analyze EEG of patients in a crossover study in the context of a course of neurorehabilitation at the Health and Rehabilitation Technologies Center of V.I. Vernandsky Crimean Federal University.