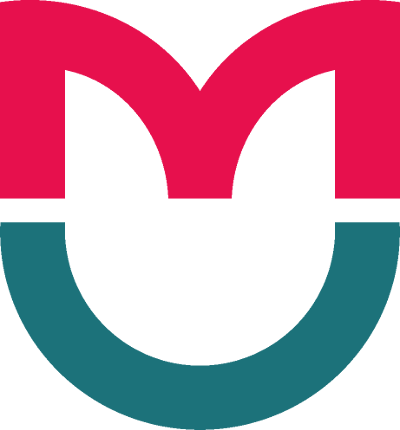
This article is an open access article distributed under the terms and conditions of the Creative Commons Attribution license (CC BY).
ORIGINAL RESEARCH
Association of local bioimpedance analysis of the abdominal region with morphological and biochemical traits
1 Lopukhin Federal Research and Clinical Center of Physical-Chemical Medicine of the Federal Medical Biological Agency, Moscow, Russia
2 Pirogov Russian National Research Medical University, Moscow, Russia
Correspondence should be addressed: Elvira A. Bondareva
Malaya Pirogovskaya, 1а, Moscow, 119453, Russia; moc.liamg@e.averadnob
Funding: the study was supported by the Russian Science Foundation (RSF grant No. 22-75-10122).
Author contribution: Bondareva EA — study design, statistical analysis, manuscript writing; Leonov GE — conducting BIA; Parfenteva OI, Gadzhiakhmedova AN — ultrasound scan, statistical analysis; Arutiunian AA, Kovaleva ON, Bevziuk NA — anthropometric measurements, BIA, ultrasound scan; Shemyakov SE — literature review, manuscript writing; Kulemin NA — manuscript writing.
Compliance with ethical standards: the study was approved by the Ethics Committee of the Lopukhin Federal Research and Clinical Center of PhysicalChemical Medicine of FMBA of Russia (protocol No. 2022/12/06 dated 06 December 2022). All the examined individuals submitted the informed consent to participation in the study.
It is well known that the pattern of fat depot distribution (body fat topography) is an independent factor associated with the severity of cardiovascular disorders, risk of metabolic diseases, and overall mortality [1]. Timely assessment of body fat topography will make it possible to determine the groups at risk of metabolic and cardiovascular disorders associated with abdominal (central) obesity, regardless of body mass index (BMI) and body fat percentage (PBF) [1]. In turn, abdominal fat is formed from the depot of subcutaneous and visceral fat. Simple anthropometric traits and indices, such as waist circumference (WC), waist-to-hip ratio (WHR), and waist-toheight ratio (WHtR), are used to estimate the abdominal fat quantity. However, these estimates showed low sensitivity at the individual level and turned out to be ineffective for separate quantitative assessment of subcutaneous and visceral fat [2]. Computed tomography (CT) and magnetic resonance imaging (MRI) are the reference methods for assessment of these components. However, these methods cannot be used for the large-scale screening of the population due to high cost, radiation exposure, and high demands on the personnel qualification. Moreover, the methods cannot be used outside the specialized medical institutions and/or research centers [3]. The method for quantitative assessment of abdominal fat, devoid of the shortcomings of CT and MRI and ensuring comparable accuracy and sensitivity, is in demand in medicine, in prediction of the risk associated with the specific features of fat accumulation in the abdominal region.
Bioimpedance analysis (BIA) of body composition is widely used in the world’s medical practice to assess body fat mass and lean body mass, as well as to assess body’s water compartments [4]. However, conventional (hand–leg) schemes allow one to obtain the integrated body composition estimates only and do not allow to judge on the fat depot distribution or to assess subcutaneous and visceral fat in the abdominal region separately. Furthermore, the contribution of the trunk to the impedance estimate does not exceed 5–10%, when the conventional electrode placement scheme is used. This means that the integrated body composition indicators are calculated based mainly on the limb impedance estimates [4, 5].
It has been shown that quantitative assessment of subcutaneous fat thickness can be performed by transimpedance measurement between two pairs of electrodes mounted directly above the region, the researcher is interested in [5]. Several electrode placement schemes for the abdominal region have been also presented. The analysis of consistency between the subcutaneous fat thickness quantitative assessment by MRI and the local impedance value in the broad morphological range has shown strong correlation between these two values (r2 = 0.984) [6].
Two studies focused on assessing local BIA leads have been published in Russia [7, 8]. Our study was aimed to analyze the relationships between the impedance characteristics obtained from the local leads in the abdominal region and the complex of morphological and biochemical traits of adult males and females. We study the relationships between local BIA leads and the complex of anthropometric traits and indices characterizing the amount of fat and its topography and complement the estimates obtained by the data on the carbohydrate metabolism markers and sonographic estimates of subcutaneous fat thickness in the abdominal region.
METHODS
A total of 189 conditionally healthy volunteers (147 females aged 18–67 years and 42 males aged 20–73 years) were assessed. Examination was performed in the outpatient clinic of the Lopukhin Federal Research and Clinical Center of Physical-Chemical Medicine of FMBA of Russia between January 2022 and April 2023. Ethnicity, physical activity level, and the number of the diseases diagnosed were determined during the questionnaire survey. The vast majority of the assessed individuals specified their ethnicity as Russian (at least one parent was Russian). Inclusion criteria: the legal age males and females diagnosed with no chronic or acute disorders at the time of examination, who had passed all the stages of examination (questionnaire survey, anthropometric measurements, blood biochemistry test), were included in the study. Exclusion criteria: age under 18 years, pregnancy and lactation, pacemaker installed, metal implants in the trunk and/or limbs.
The assessment program included measuring standing height (SH) with the laser anthropometer (KAFA; Russia), body weight (BW) (Seca; Germany), waist (WC) and hip (HC) circumference with the non-elastic measuring tape. BIA was performed using the АВС-02 Medas system (Medas; Russia) with the probing current frequency of 50 kHz in two leads
- Using the standard tetrapolar scheme “wrist — ankle joint” on the right side of the body with the F3001 placement (FIAB; Italy) and the subject in supine position.
- To estimate subcutaneous fat in the abdominal region, the source electrodes were placed at a distance of 12 cm, and the measurement electrodes were placed at a distance of 7 cm to the left and right of the umbilicus when the subject was standing [6] (fig. 1А).
The АВС01-0362 software was used to determine active and reactive resistance (R, Ohm — for the integrated scheme; Rsc, Ohm — for the local scheme) and appropriate impedance values (Z50 and Z50sc). The integrated lead values were used to determine absolute and relative body fat mass (FM and PBF, respectively) and lean body mass (LM).
The BodyMetrix BX2000 ultrasound scanner (IntelaMetrix; USA) was used to determine subcutaneous fat thickness (mm) in two points of the abdominal region of each subject: near the umbilicus (SFL1) and above the iliac crest (SFL2), which corresponded to the suprailiac skinfold (fig. 1B) [9]. The Mediagel medium viscosity ultrasound gel (Geltek; Russia) was used as the contact medium.
Anthropometric traits were used to calculate the following indices: BMI — body mass index, WHR — waist-to-hip ratio, WHtR — waist-to-height ratio [10]. Nutritional status (underweight, normal weight, overweight or obesity) was determined based on BMI in accordance with the WHO criteria. Furthermore, obesity was diagnosed based on the PBF ≥ 30% for females and ≥ 25% for males. Central obesity was assessed based on WHtR ≥ 0.5 [10].
Blood biochemistry test was performed to estimate the carbohydrate metabolism parameters (insulin, fasting glucose, and glycated hemoglobin levels). These data were used to calculate the HOMA (Homeostasis model assessment) indices: HOMA-IR, HOMA-%B, HOMA-%S. Furthermore, HOMA2-IR, HOMA2-%B, and HOMA2-%S values were calculated using the online calculator (https://www2.dtu.ox.ac.uk/homacalculator/). HOMA-IR > 2.7 was considered to indicate insulin resistance.
Statistical analysis
Parameters of the studied traits’ distribution position, variation, and shape were assessed using the PAST tool (https://www.nhm.uio.no/english/research/resources/past/). The Shapiro– Wilk, Anderson–Darling, Lilliefors, and Jarque–Bera tests were used to test consistency of the data with normal distribution. The Monte Carlo algorithm was implemented in the calculation procedures for all tests, except the Shapiro–Wilk test. When performing statistical comparison, we did not limit ourselves to calculating p-values, but assessed the Cohen's standardized effect size (dC) for pairwise comparison and the ϵ2 measurement for the Kruskal–Wallis test. The effect sizes, the lower limits of 95% confidence intervals (CI) of which exceeded dС = 1, were accepted as noteworthy [11].
The Welch's t-test, Mann–Whitney U test, and Kruskal– Wallis test were used to assess the differences between groups. The Benjamini–Hochberg procedure was applied when performing multiple comparisons. The analysis of differences between groups and the correlation analysis were performed using Past and JASP (JASP Team (2024). JASP (Version 0.18.3) [Computer software]), as well as the following software packages: “ggpubr” (Kassambara A (2023). _ggpubr: 'ggplot2' Based Publication Ready Plots_. R package version 0.6.0,
RESULTS
The subgroups of males and females did not differ in age, BMI, WHtR, absolute body fat mass, Z50sc, subcutaneous fat thickness in the abdominal region, biochemical parameters, and HOMA2 indices. The traits, for which significant differences (p < 0.001) between the subgroups of males and females have been reported, are provided in tab. 1.
According to the data provided (tab. 1), no sexual dimorphism is reported for the values of subcutaneous fat thickness in the abdominal region and local impedance of the abdominal region. In the context of sex-related differences in WC and WHR, the lack of sexual dimorphism in the SFL1, SFL2, and Z50sc values can indicate differences in the amount of visceral fat and the possibility of visceral fat quantification by local BIA methods. That is why the examination should be complemented by the local lead in the sagittal area of the abdomen. The correlation analysis of the complex of studied traits (fig. 2) revealed significant correlations of Z50sc with WHtR (0.739 [0.667; 0.797]), body weight (0.609 [0.510; 0.691]), waist circumference (0.700 [0.510; 0.766]), BMI (0.731 [0.657; 0.791]), FM and body fat percentage (0.724 [0.648; 0.786] and 0.662 [0.573; 0.735]), subcutaneous fat thickness in the abdominal region (0.692 [0.608; 0.761] and 0.743 [0.671; 0.802]), insulin concentration (0.541 [0.428; 0.637]), HOMA2-IR (0.539 [0.425; 0.636]), and HOMA2-%S (–0.539 [–0.636; –0.425]).
A broad spectrum of morphological traits represented in the studied sample makes it possible to conduct comparative analysis of Z50sc values in various subgroups: by the nutritional status determined based on BMI, by the fact of having obesity determined based on the PBF value, as well as by the fact of having abdominal obesity determined based on the WHtR value (tab. 2).
The increased values of the traits associated with the risk of cardiometabolic disorders (abdominal obesity, insulin resistance, and body fat percentage) are correlated to the higher local impedance values. The differences between subgroups are confirmed not only by the significance level, but also by the large effect size (tab. 2).
Then ROC analysis was performed for these traits in order to assess the possibility of using Z50sc as a diagnostic criterion for abdominal obesity, bidy fat percentage, and insulin resistance (fig. 3). The highest AUC values are reported for the WHtR index. The AUC value reported for HOMA-IR is lower, however, it is significantly different from the “don’t care” value (AUC = 0.5), which suggests the need for further study of Z50sc involving the groups diagnosed with prediabetes and type 2 diabetes mellitus.
Thus, higher values of the traits associated with the increased fat accumulation and the risk of comorbidities correspond to the high local impedance values (fig. 3). The AUC values suggest that Z50sc can be potentially used as a diagnostic test, while certain Z50sc threshold values can constitute an additional noninvasive express method for assessment of cardiometabolic risk in the future.
The combination of BMI and PBF can confirm the diagnosis of obesity and select individuals with normal-weight obesity [12] and individuals with the increased BMI (excess body weight), but normal fat percentage. Fig. fig. 4 presents Z50sc values in the subgroups with increasing body weight and body fat percentage: underweight (UW) — BMI < 18.5 kg/m2; normal body weight and PBF (NWNO) — BMI within the range of 18.5–24.9 kg/m2 and PBF < 25% for males and < 30% for females; normal-weight obesity (NW_O) — BMI within the range of 18.5–24.9 kg/m2 and PBF ≥ 25% for males and ≥ 30% for females; excess body weight based on BMI and normal PBF (OWNO) — BMI within the range of 25.0–29.9 and PBF < 25% for males and < 30% for females; excess body weight based on BMI and obesity based on PBF (OWO) — BMI within the range of 25.0–29.9 and PBF ≥ 25% for males and ≥ 30% for females; obesity based on BMI and PBF (OB) — BMI > 29.9 and PBF ≥ 25% for males and ≥ 30% for females.
The findings suggest that local impedance estimates in the abdominal region increase with increasing BMI and PBF. We revealed significant differences between subgroups with normal body weight and the subgroup with normal-weight obesity (NWNO vs. NW_O; p = 0.029), along with the lack of significant differences between the subgroups with normal-weight obesity and excess body weight and obesity (NW_O vs. OWO; p = 0.097), as well as between the NWNO and OWNO subgroups (p = 0.118). The subgroup with obesity (OB) significantly differs from other groups (p < 0.005). There are no differences in the waist circumference, subcutaneous fat thickness near the umbilicus, and WHtR between NWNO and NW_O, however, there are significant differences in SFL2 between these subgroups (p = 0.015).
DISCUSSION
The search for the best characteristics describing individual obesity phenotype has shifted from anthropometric measurements to separate assessment of body fat mass and lean body mass and nowadays to quantitative assessment of various fat depots and the features of fat distribution [13, 14]. There are incomparably more studies focused on assessing the possibility of visceral fat quantification by BIA, than that focused on subcutaneous fat in the abdominal region [15].
In our study we conducted analysis of the correlation of impedance in the abdominal region determined using the Russian bioimpedance analyzer (АВС-02 Medas) with subcutaneous fat thickness in the abdominal region and the biochemical carbohydrate metabolism markers. The results obtained confirm a close relationship between the estimates obtained in the local BIA lead and the complex of morphological and metabolic traits characterizing subcutaneous fat accumulation in the abdominal region. It should be noted that foreign manufacturers of bioimpedance equipment produce serial products (Y-scope InBody, Korea and Maltron BioScan 920-II, UK) allowing one to quantify subcutaneous and visceral fat in the local leads. However, manufacturers have not published the estimation equations. That is why, in our opinion, investigation of the capabilities of domestic BIA, widely used in the medical and research institutions of the RF, for estimation of subcutaneous and visceral fat using local schemes, is particularly relevant.
No sexual dimorphism is reported for Z50sc, in contrast to WC and PBF. Coefficients of Z50sc correlation with WHtR, BW, WC, BMI, SFL1, and SFL2 are higher, than that for PBF determined using the integrated BIA scheme. However, it should be noted that the 95% CI of the correlation coefficients of Z50sc, PBF and WC for almost all pairs of traits overlap, which suggests that there are no significant differences between these correlational pairs in the studied sample (fig. 5). The correlations of Z50sc and PBF with insulin levels were stronger, than the correlation of insulin with WC, however, local assessment has no advantage over the PBF calculated using the conventional scheme.
The correlation estimates obtained in the study for the pairs Z50sc–PBF and Z50sc–WC are close to the estimates obtained in the earlier study [7]. While the estimates obtained for the group of Altai people turned out to be lower for the pair Z50sc–WC and similar for the pairs Z50sc–WHtR and Z50sc–PBF [8].
The ROC analysis results (fig. 3; HOMA-IR) suggest that Z50sc can be used as the additional criterion for assessment of insulin resistance in adults, however, it is necessary to increase the sample size and include individuals with confirmed cardiometabolic disorders in the group. It is also necessary to assess the Z50sc diagnostic value in the groups formed based on sex. Quantitative assessment of abdominal fat is also important in terms of studying the white adipose tissue remodeling, for both assessment of the development of metabolic disorders associated with obesity and estimation of the efficacy of a number of drugs [13, 16]. The relationship between two quantitative characteristics of subcutaneous fat (local BIA and subcutaneous fat thickness determined by ultrasonography) makes it possible to develop the equation for calculation of subcutaneous fat thickness or cross-sectional area based on the АВС-02 Medass local lead indicators. With appropriate verification by reference methods, such equations can replace CT and MRI in the large-scale screening studies [6, 16].
The estimates obtained in local BIA leads (impedance, active and reactive resistance) can be used to develop various indices, such as indices derived from active resistance [8, 17]. We have also analyzed the subcutaneous fat index (Rsc*WC, m2). SFI was proposed earlier [7] by analogy with the visceral fat index reported in the earlier study [17]. To date, these indices have not been validated as the criteria for assessment of the risk of comorbidities in obesity. The correlations of SFI with WHtR (0.87 [0.71; 0.90]), WC (0.87 [0.81; 0.91]) and WHR (0.66 [0.59; 0.73]) are higher, than the correlation of Z50sc with these indices (0.74 [0.67; 0.80], 0.70 [0.62; 0.77], and 0.49 [0.38; 0.59], respectively). The correlation of Z50sc with SFI is 0.95 [0.92; 0.97]. In general, SFI has shown no fundamental differences from Z50sc, except for significant differences between subgroups of females and males (36.2 (29.5) vs. 47.9 (35.3) Ohm/m2; p = 0.021). The SFI correlation etimates are consistent with the earlier reported data [7], however, the median values and SFI correlations with morphological traits turned out to be significantly lower in the group of ethnic Altaians [8].
It is interesting that among all stidied traits only Z50sc and SFL2 make it possible to distinguish people with normalweight obesity from the group with the same BMI having normal body fat percentage. Furthermore, groups with high body fat percentage and different BMI show no differences in Z50sc, while significant differences in WC, WHtR, and SFL1 are reported for these groups. Similarly, the subgroups having no obesity based on PBF, but different BMI, show no differences in Z50sc. This is due to the fact that Z50sc to the greater extent reflects the fat component, while WC and WHtR serve as the surrogate estimates of abdominal fat, and high values of these traits can be associated with the development of skeletal muscles in physically active people. Or, alternatively, normal WC and WHtR values result from the muscle tissue replacement with fat together with the preserved low waist circumference, as in normal-weight obesity. In general, the pattern of local impedance assessment in the abdominal region in these groups corresponds to PBF determined in the integrated scheme, but the correlation of Z50sc with PBF in the combined sample of males and females is 0.662 [0.573; 0.735], i.e. it is not high.
The study limitations include small size of the group of underweight adults (BMI < 18.5 kg/m2), as well as the group of individuals with low fat percentage and well developed skeletal muscles.
CONCLUSIONS
The study has shown the correlation of the Z50sc trait obtained in the local BIA lead using domestic bioimpedance equipment with the complex of anthropometric and biochemical traits in males and females. In general, high Z50sc values correspond to the more pronounced fat deposition and its abdominal topography. Correlations of Z50sc with subcutaneous fat thickness in the abdominal region make it possible to develop equation for recalculation of Z50sc into subcutaneous fat thickness in the abdominal region (cross-sectional area of subcutaneous fat). The results of the ROC analysis of Z50sc with the HOMA-IR index make it possible to consider the local BIA lead as a simple noninvasive criterion for assessment of carbohydrate metabolism disorders associated with excess fat accumulation in the abdomen in the future. However, further research focused on determining quantitative characteristics of such test for assessment of the Z50sc diagnostic value is required: assessment of the threshold value, sensitivity and specificity. The quantitative estimates of fat depots are used to develop the criteria (for example, adjusted for BMI and sex) for differential assessment of the risk of comorbidities [18]. In prospect there is a possibility of developing equations for quantitative assessment of the subcutaneous and visceral fat depot area based on the data obtained in the local BIA leads and the indices based on these data. Such estimates can become the new additional diagnostic criteria for assessment of individual risk of cardiometabolic disorders or personalized approach to treatment of those depending on the patients’ sex and age.