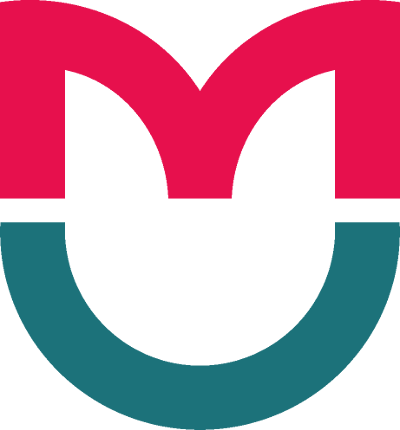
ORIGINAL RESEARCH
Vitamin status of urban and rural school children and specifics of free radical reactions in their blood serum
1 Hygiene and Epidemiology Department, Institute of Professioanl Education,Orenburg State Medical University, Orenburg, Russia
2 Chemistry and Pharmaceutical Chemistry Department, Pharmaceutical Faculty,Orenburg State Medical University, Orenburg, Russia
Correspondence should be addressed: Ekaterina V. Bulycheva
ul. Sovetskaya, d. 6, Orenburg, Russia, 460000; ur.liam@aninsos-e
Change in parameters of homeostasis, particularly the level of free radical reactions in the body, may indicate stress in modern school children studying in urban and rural schools [1, 2, 3, 4, 5]. It has been established that in stress, neurohumoral regulation systems are activated under the influence of a "primary mediator" [6]. Free radicals and lipid peroxidation products [7] are act as primary mediators.
Change in redox balance triggers protective and adaptive reactions in the body, which involve antioxidant vitamins [8]. Vitamin A (retinol), E (tocopherol) and C (ascorbic acid) are the classical antioxidants [9, 10, 11, 12]. In particular, tocopherol is the universal protector of cell membranes, preventing oxygen from contacting with unsaturated fatty acids of membrane lipids and lipid peroxidation. Due to the presence of double bonds in the molecule, retinol can react with various reactive oxygen species. The antioxidant function of ascorbic acid is due to its ability to easily give two hydrogen atoms required for neutralization of free radicals. This vitamin is very effective in high concentrations [13]. B vitamins may have indirect influence on free radical oxidation and antioxidant system. This assumption is based on the fact that the body’s antioxidant system consists of various protective mechanisms [14]. These include superoxide dismutase, catalase, peroxidase, and other enzymes. Proper amino acid metabolism, including metabolism regulated by thiamine (vitamin B1), riboflavin (vitamin B2) and pyridoxine (vitamin B6) is important for these enzymes.
The study aims at conducting a comparative analysis of the vitamin status of urban and rural school children with levels of free radical reactions in their blood serum.
METHODS
Two experimental groups were created. The first group included urban school children living in the city of Orenburg (n = 250), while the second group consisted of school children living in villages located in Orenburg region (n = 200). Subgroups — 12–14 year olds (n = 150 and n = 100 among urban and rural school children respectively) and 15–17 year olds (n = 100 in both groups) — were marked out from each of the two experimental groups. The study included school children aged 12–17 years, who have no chronic diseases, have not been sick for a month before the study and residing in an area with the same anthropogenic load (within the group). The exclusion criterion here was unwillingness by the children or their parents to participate in the study. The Ethics Committee of Orenburg State Medical University approved the study. The parents of the school children gave a written consent for the participation of their children in the study. The samples for the study in the two groups were obtained simultaneously in three months after the children have commenced classes at the school.
Venous blood and urine were the biological material for the study. Blood was collected in the morning before the children could take their breakfast. 10 ml of blood was collected in Vacuette (BD, USA) vacuum tubes without an anticoagulant. It took 2 hours to deliver the blood samples to the lab. Transportation and storage was carried out at a temperature of 18–25 °C. Urine was collected in the morning after sleep or no earlier than after 2–3 hours after the previous urination. The urine samples (not less than 20 ml in volume) were collected in dry sterile vials. Transportation and storage took 3 hours. The biomaterial was transported and stored at a temperature of 18–25 °C.
Iron-initiated chemiluminescence was used to assess the level of free radical reactions in the blood serum. After collecting blood, it was held for 30 minutes and then centrifuged at 1500 rpm for 15 minutes. The resulting serum was diluted with phosphate buffer (2.72 g KH2PO4 and 7.82 g KCl in 1 liter of distilled water, pH 7.4). The resulting solution was titrated with saturated KOH solution until pH 7.45 was achieved. Chemiluminescence registration was performed according to Farkhutdinov technique [15] on Chemiluminometer-3 device (Lumex, Russia). The sensitivity of the device is about 200 photons/sec. The device was calibrated before commencement of work on reference light source (luminescent uranium glass JS-19). The maximal intensity of the fast signal, lightsum of the slow signal (S) and the tangent of the backside angle of the chemiluminescence signal were determined. Indicator values were given in relative units, having calculated their ratio for experimental and blank control samples.
The level of excretion of B vitamins and vitamin C in the urine and the content of vitamins A and E in the blood serum were examined.
Analysis of specimens for the presence of retinol and tocopherol was performed using bioliquid analyzer Fluorat 02-ABLF (Lumex, Russia). In preparing the samples, 1 ml of blood serum and 1 ml of ethanol were placed in one tube for centrifugation, while 1 ml of distilled water and 1 ml of ethanol (calibration pattern) were placed in the other tube. Both tubes were shaken in Vortex apparatus for 30 seconds after which 5 ml of hexane was added and again shaken for 1 minute. After shaking the tubes, they were centrifuged for 10 minutes at 1500 rpm. The separated hexane layer (extract) was used for fluorimetric analysis. Content of vitamin A or E (X, μg/ml) in the blood serum was calculated using the formula:
X = Cvit × VE × Q / Vс,
where Cvit is vitamin concentration in the extract (measured in μg/ml); VE is the extract volume (measured in ml); Vc is the blood serum volume taken for analysis (measured in ml); Q is the coefficient reflecting extract dilution.
Content of ascorbic acid and B vitamins was determined by the level of their excretion in urine. Visual titration with Tillman’s reagent (2,6-dichlorophenol sodium) was used for vitamin C. 10 ml of urine, 10 ml of distilled water and 1 ml of 10 % HCl solution were measured in two conical flasks. The contents of each flask was stirred and titrated with 0.001 N solution of Tileman’s reagent until pink color appeared and remained for 30 seconds. Vitamin C content (X, mg/h) was calculated by the formula:
X = 0,088 × А × C / B,
where 0.088 is the coefficient reflecting the amount of ascorbic acid, which is equivalent to 1 ml of 0.001 N solution of 2,6-dichlorophenol sodium (measured in mg); A is the arithmetic average of results of titration of 0.001 N solution of 2,6-dichlorophenol sodium of two urine samples (measured in ml); B is the amount of urine taken for titration (in ml); C is the average daily amount of urine (1500 ml for boys and 1200 ml for girls).
Thiamine excretion in the urine was determined by Wang & Harris technique. Riboflavin levels in urine were estimated by Maslennikova & Gvozdeva method, while those of pyridoxine were calculated by fluorimetric method [16].
The actual nutrition by the school children was estimated based on the meal taken by them in the last 24 hours [17]. This was done using a questionnaire. After that, the biological values of the diets were estimated using tables of chemical composition of food products proposed by Skurikhin & Tutelian [18]. The data obtained were compared with physiological norms for each age group [19].
The sample size was calculated using Sanetliyev’s formula (1968) where n is the number of observations, t is the confidence coefficient, p is the prevalence indicator, q = 100 % – p, ∆ is the confidence interval. Considering that in medical research, 95% is the minimum confidence level, which corresponds to a confidence factor t = 1.96, we took p equal to q, i. e. 50 %, in order to maximize the product of p and q, while 100 % was taken as the confidence interval. The amount of minimum sample calculated in this way (ensuring representativeness) was n = 100. Student’s test was applied with subsequent calculation of significance (p). This was to identify statistically significant differences in the experimental groups. The data were statistically processed using software package Statistica 5.0, which automatically calculated the mean values, standard deviation and standard error of the mean.
RESULTS
The retinol content and tocopherol content in the blood serum of urban schoolchildren aged 12–14 was less than the physiological norm (hereinafter compared with a lower bound) by 26.7 % and 12.8 % respectively, and 20.0 % and 9.8 % respectively in children aged 15–17 (tab. 1). The content of retinol and tocopherol in the serum of rural schoolchildren was slightly higher, but also was less than the physiological norm: 6.7 % and 6.8 % respectively for children aged 12–14, and 10.0 % and 9.8% respectively for children aged 15–17. Vitamin C excretion in the urine of urban schoolchildren of both age subgroups corresponded with the lower bound of the physiological norm. In rural children, the figure was 14.8 % and 12.1 % below the norm for the students aged 12–14 and 15–17 respectively.
The low urinary excretion levels of thiamine compared with the norm were established only for the rural schoolchildren in both age subgroups (tab. 2). Insufficient urinary excretion of riboflavin was observed in all the schoolchildren, except for rural children aged 15–17 (14.2 ± 0.24 mkg/h — matches the lower bound of the physiological norm). Low urinary excretion of pyridoxine was observed only in rural children: in a group of children aged 12–14, vitamin B6 excretion was by 5.2 % below the norm, 15–17 years — by 6.5 %.
Analysis of the daily diets of the schoolchildren showed that vitamins A and E were seriously lacking in the diets of urban and rural school children (tab. 3). In the 12–14-year age sub group, the content of retinol in their diet was by 88.3 % and 66.7 % below the norm in urban and rural school children respectively. In the 15–17-year age subgroup, the figures were 78.0 % and 83.0 % respectively. Tocopherol deficiency was 59.2 % and 71.7 % in urban and rural school children respectively, aged 12–14, and 66.0 % and 70.7 % for those aged 15–17 years. Ascorbic acid content in the diets of all groups was within the norm. Thiamine deficiency was detected in urban and rural school children aged 15–17 years, riboflavin — in all groups, except the urban group, aged 12–14 years, pyridoxine — in all groups.
The values of all chemiluminescence parameters were significantly higher for both age subgroups of urban schoolchildren (p < 0.05). For instance, the value of the maximal intensity of the fast signal of blood serum in the urban children aged 12–14 and 15–17 were respectively 4.3 and 5.9 times higher than in the rural children. This indicates a higher content of lipid hydroperoxides in the blood serum of the urban children; the value of the lightsum (lipid peroxidation) was 2.2 and 4.2 times higher; the value of the tangent of the backside angle of the chemiluminescence signal (speed of lipid oxidation) was 3.8 and 7.6 times higher (tab. 4).
DISCUSSION
The low vitamin status of modern schoolchildren has been also confirmed by other researchers [21, 22, 23]. The identified difference in the level of free radical reactions in the blood serum of urban and rural school children is consistent with the findings about their vitamin status: the antioxidant vitamin A and E status of urban school children is slightly lower than that of rural children. The level of free radical reactions in the blood serum of urban children is higher than rural children.
The diet of urban children is typically more balanced than the diet of children in rural areas. According to Ushakov & Sokolova [5], about 70 % of urban schoolchildren in winter take butter, cheese, meat, fowl, fish, raw vegetables and fruits almost every day or 2–3 times a week. On the other hand, less than 40 % of rural schoolchildren take similar diet. Predominance of potatoes, pork and milk in the diet of the schoolchildren has a positive correlation with ownership of a kitchen garden and part-time farms by the rural population. This explains why the serum levels of vitamin C in urban school children corresponds with the physiological norm but are deficient in the body of rural children. At the same time, according to Leshchenko, 53.5 % of urban schoolchildren and 35.5 % of rural schoolchildren eat only 1–2 times a day [24]. This eating frequency cannot provide the body with adequate amount of vitamins. This could explain the fact why even at eating more diverse meals, the vitamin A and E status of the urban school children still remained lower.
CONCLUSIONS
The blood serum of urban and rural schoolchildren was found to have vitamins A and E deficiency. However, the deficiency is more pronounced in urban schoolchildren. In all likelihood, this is due to higher content of oxidation products in their blood serum, as evidenced by the chemiluminescence indicator values that are by 2.2–7.6 times higher than in the rural children. Analysis of diets confirmed the findings: it was established that there is deficiency of vitamins A, E and pyridoxine in the diets of all the groups of students and that there is deficiency of thiamine in the diets of older students. The foregoing necessitates adjustment of nutrition in schools.