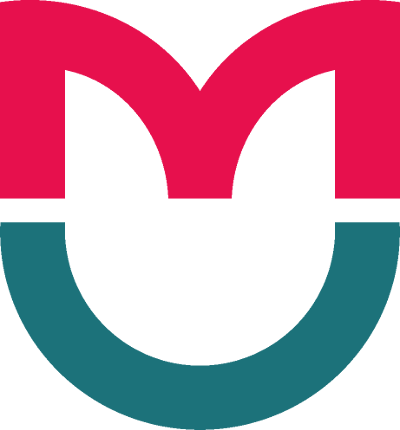
ORIGINAL RESEARCH
Fluorescence imaging of actin cytoskeleton changes in cancer cells upon chemotherapy
1 Research Institute of Biomedical Technologies,
Nizhny Novgorod State Medical Academy, Nizhny Novgorod, Russia
2 Lobachevsky State University of Nizhny Novgorod – National Research University, Nizhny Novgorod, Russia
3 Laboratory of Biophotonics, Department of Genetics and Postgenomic Technologies,
M. M. Shemyakin and Yu. A. Ovchinnikov Institute of Bioorganic Chemistry, Russian Academy of Sciences, Moscow, Russia
Correspondence should be addressed: Natalia Klementieva
pl. Minina i Pozharskogo, d. 10/1, Nizhny Novgorod, Russia, 603005; moc.liamg@aveitnemelkvn
Funding: this work was supported by the Russian Science Foundation (project no. 14-25-00129).
Acknowledgements: authors thank the IBCH Core Facility for the equipment.
Actin and actin-binding proteins are the cytoskeleton components forming microfilaments. They are involved in a variety of processes, such as cell growth, motility, division, transcription regulation, cell-matrix and cell-cell interactions. The actin cytoskeleton participates in signal transduction cascades and intracellular transport of proteins and organelles [1]. Energy-dependent polymerization and depolymerization of actin occur continuously inside the cell. The assembly of new actin filaments is accompanied by the formation of specialized structures, such as stress fibers, filopodia, lamellipodia. Actin cytoskeleton rearrangements can manifest cell dysfunction and its malignant transformation [2]. Cancer cells use various mechanisms including changes in adhesive and mechanical properties to support tumor survival and growth. The actin cytoskeleton structure can determine cancer cell stiffness, motility and invasion capacity [3].
Studying the structure of the actin cytoskeleton is essential for understanding the mechanism of antitumor drug action. For example, the effect of small interfering RNA inhibitors on cancer cell survival was demonstrated through visualization of actin cytoskeleton rearrangements [4]. The actin pattern also served as a criterion to assess the sensitivity of lung cancer cells to chemotherapy [5]. Moreover, actin itself can be used as a therapeutic target due to its potential to regulate tumor growth and metastasis formation [6, 7]. However, compounds that disrupt microfilament organization are highly toxic and have not been approved for clinical use yet.
Evaluation of actin cytoskeleton response to treatment with clinically approved anticancer drugs is a promising area of research. As a rule, malignant tumors are heterogeneous. Antitumor treatment outcome largely depends on the elimination of the most aggressive cell subpopulations. It has been shown that the most marked changes in the actin cytoskeleton occur in cells with metastatic capacity [8, 9]. Understanding the actin cytoskeleton dynamics in response to standard chemotherapy is important to detect potentially metastatic cells inside the tumor.
The aim of this work was to study structural changes in the actin cytoskeleton in cancer cells upon treatment with chemotherapy drugs paclitaxel and cisplatin widely used in clinical practice.
METHODS
We used HeLa Kyoto (human cervical cancer) cell line as a model system. The cells were cultured in Dulbecco's Modified Eagle's medium (PanEco, Russia) supplemented with 10 % fetal bovine serum (HyClone, GE Healthcare Life Sciences, USA), 2 mM glutamine (PanEco), 50 µg/ml streptomycin (PanEco), and 50 units/ml penicillin (PanEco) in an atmosphere of 5 % CO2 at 37 °C.
For our experiment, we selected paclitaxel (Taxol) by Bristol-Mayers Squibb, USA, and cisplatin (Cisplatin-Teva) by Teva Pharmachemie, Netherlands. Cytochalasin D (Enzo Life Sciences, USA), an inhibitor of actin polymerization, was used as a control compound. To calculate LC50,/sub> of the chemotherapy drugs we applied the MTT assay [10], with drug-incubation period of 24 hours. Optical density of staining was measured on a Synergy Mx plate reader (BioTek, USA) at the wavelength of 570 and 630 nm.
200,000 cells were seeded on 35 mm glass-bottom dishes (Fluorodish by WPI Inc., USA). The next day the culture medium was replaced with fresh medium containing chemotherapy drug (LC50), and cells were incubated for 24 hours under standard conditions. Cells without the adding drug were used as a control. Cells were then washed once with DPBS solution (PanEco), placed in 1 ml of FluoroBrite DMEM culture medium (Thermo Fisher Scientific, USA) and stained.
SiR-actin probe (Spirochrome, Switzerland) at 0.5 µM concentration and Hoechst 33342 (Molecular Probes, Thermo Fisher Scientific) at 5 µg/ml concentration were used to stain actin and nuclei, respectively. After 30-minute staining, fluorescence imaging was performed. SiR-actin [11] allowed for endogenous actin labeling in live cells without the need for fixation or wash procedure. The control and three experimental samples (with paclitaxel, cisplatin or cytochalasin D) were imaged; each sample was assessed in 10 fields of view.
For alpha-actinin labeling, we used pTagRFP-actinin expression vector (Evrogen, Russia). Cells were transiently transfected with pTagRFP-actinin using the X-tremeGene 9 reagent (Roche, USA) according to the manufacturer’s protocol. The day after transfection, cells were incubated with the chemotherapy drugs (LC50) for 24 hours. Cells were washed in DPBS solution and placed into 1 ml MEM culture medium (Sigma-Aldrich, USA). Then, fluorescence microscopy was performed. The control and three experimental samples (with paclitaxel, cisplatin and cytochalasin D) were studied. Each sample was assessed in 5–6 fields of view.
Fluorescence imaging was carried out on a Nikon Eclipse Ti inverted microscope (Nikon, Japan) equipped with 100X Apo TIRF/1.49 oil objective (Nikon) and EM-CCD-camera iXon3 DU-897 (Andor, UK). To collect a fluorescence signal from SiR-actin, we used a C-NSTORM QUAD filter (Nikon) and 640-nm laser (power density of 7.8 W/cm2). To detect Hoechst 33342 fluorescence, a Nikon Intensilight fluorescence lamp and a BV-2A filter were used. Actin cytoskeleton was visualized by total internal reflection fluorescence microscopy (TIRF). TIRF-mode allows for the observation of thin layers (less than 200 nm) close to the cell membrane and ensures the highest signal-to-noise ratio; it is optimal for super-resolution single- molecule localization microscopy. Data processing was done with Fiji software [12]. TagRFP-based super-resolution microscopy was performed as described previously [13].
RESULTS
First, we determined the concentrations of chemotherapy drugs for treatment. Based on MTT assay results, viability/drug concentration curves for HeLa Kyoto cell culture were obtained. LC50 values for paclitaxel, cisplatin and cytochalasin D were 45 µM, 7 µM and 12.5 µM, respectively.
Then, we assessed the effect of chemotherapy drugs on the actin cytoskeleton of cancer cells using fluorescence microscopy. fig. 1A shows typical HeLa Kyoto cells stained with SiR-actin (the control sample). Their actin cytoskeleton represents an extensive network of long stress fibers running across the cytoplasm in various directions. There are protrusions at the leading edge of the cell, as actin is enriched in the cell cortex. The image also shows a web-like meshwork of thin microfilaments that fill the entire cytoplasm. Cells under cytochalasin D treatment do not contain typical stress fibers. Their formation is driven by assembly of microfilaments from actin monomers, but in the presence of cytochalasin D this process is impeded. Here, short filaments along with dotted actin structures evenly distributed over the cytoplasm are typical. Stress fibers are rare and can be found near the plasma membrane only. Cells are more rounded and do not have protrusions at the leading edge (fig. 1B). No significant damage to the microfilament organization is observed in cells treated with cisplatin. Here, HeLa Kyoto cells have a typical irregular shape with numerous stress fibers commonly found in spread cells. However, unlike the control sample, cells do not contain a network of thin microfilaments; no actin enrichment is found in the cell cortex after cisplatin treatment (fig. 1C). The paclitaxel sample reveals a more dramatic reorganization of the actin cytoskeleton, as compared to the cisplatin sample. For example, cells have almost no cytoplasmic protrusion and tend to be more round. Actin is mainly accumulated in the cell cortex. Microfilament fibers are rare, and stress fibers are absent (fig. 1D). Notably, the majority of cells are multinucleated.
A more accurate analysis of actin cytoskeleton changes was performed using super- resolution single-molecule localization fluorescence microscopy. We visualized a fine structure of the actin-binding protein alpha-actinin. In the control sample, cells show a typical pattern of alpha-actinin distribution along the actin bundles. Alpha-actinin completely fills the cytoplasm forming slight thickening at focal adhesion sites (fig. 2A). Cytochalasin D triggers complete disorganization of alpha-actinin, which is in accordance with actin aggregation and the absence of long stress fibers observed by TIRF microscopy. Isolated alpha-actinin structures of 200-250 nm in diameter are evenly distributed throughout the cell (fig. 2B). Cells treated with paclitaxel become round and lack protrusions and stress fibers. Alpha-actinin is accumulated in the cell cortex. The subdiffraction-resolution image shows substantial thickening and convergence of alpha-actinin fibrils at focal adhesion sites (fig. 2C). The least conspicuous changes are induced by cisplatin. Here, cells have an irregular shape and a common dotted cytoskeleton (fig. 2D). The structural changes include a more dense packing of alpha-actinin.
DISCUSSION
In this work, we have studied the effect of chemotherapy drugs paclitaxel and cisplatin on the actin cytoskeleton structure.
Paclitaxel belongs to the taxane family. Its mechanism of action is associated with the disruption of tubulin system; stabilization of microtubule assembly inhibits cell proliferation. Murakami et al. [14] have shown that taxane-based therapy reduces alpha-actin content in the stromal cells of breast tumors. Our findings demonstrate that, besides microtubules, microfilament system of cervical cancer cells also responds to paclitaxel treatment. Significant reorganization of actin structures observed (the appearance of rare thin curved filaments in the absence of stress fibers) is in agreement with the results obtained with MCF7 breast cancer cells treated with taxanes [15].
Interestingly, the majority of cells became multinucleated after incubation with paclitaxel. Its binding to beta-tubulin increases the number of cells in the G2/M phase and induces apoptosis and polyploidy [16, 17]. Actin skeleton rearrangements detected in HeLa Kyoto cell line could be indirectly related to the multinucleated phenotype. It is known that in normal multinucleated cells, such as osteoclasts, actin undergoes significant changes and represents isolated podosome-forming structures [18]. Giant multinucleated cells have been observed in HeLa-derived cell culture where actin is mainly accumulated in microspikes at the ventral face of the cell and in dotted podosome-like structures [19].
No direct link between the therapeutic effect of cisplatin and its impact on the actin filament rearrangement has been established. The mechanism of cisplatin action is associated with the ability of platinum ions to form intrastrand cross-links with DNA purine bases. As a result, DNA repair is inhibited and DNA damage induces apoptosis in cancer cells [20]. Nevertheless, the issue of cancer cell resistance to cisplatin and the role of actin in this process have been studied. For example, cisplatin-resistance cancer cells demonstrate changes in the expression of some cytoskeletal proteins, including actin, manifesting as abnormal actin-filamin dynamics [21, 22]. Sharma et al. observed conspicuous difference in the organization and mechanical properties of actin filaments in cisplatin-resistant ovarian cancer cells (OVCAR5), compared to the sensitive cells [23]. In their work, super-resolution microscopy was used for actin visualization that allowed them to detect specific patterns at the subdiffraction level, such as individual bundles or extensions of the cell at focal adhesion sites. According to our findings, cisplatin has a weaker effect on the actin cytoskeleton of HeLa Kyoto cells, compared to paclitaxel. At the same time, super-resolution microscopy data indicate a high packing density of microfilaments that can potentially disrupt normal functioning of cancer cells.
CONCLUSIONS
We have studied the effect of two chemotherapy drugs (paclitaxel and cisplatin) on the actin cytoskeleton in HeLa Kyoto cervical cancer cells using fluorescence microscopy. We have found that paclitaxel leads to massive reorganization of the actin skeleton expressed as disassembly of stress fibers, actin accumulation in the cell cortex, thickening and convergence of focal adhesion sites. Cisplatin caused smaller changes, namely, reduction in the number of thin microfilament bundles and denser packing of alpha-actinin. We observed structural changes of actin cytoskeleton induced by chemotherapy drugs not specifically targeting it. In this light, actin might be considered as an additional target for anticancer therapy. Such reorganization of the microfilament system may affect metastatic and invasive capacities of cancer cells within solid tumor in vivo. We are planning to study the effect of chemotherapy on the actin cytoskeleton structure in a mouse tumor model.