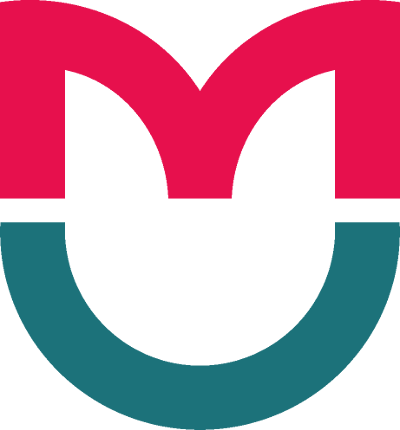
This article is an open access article distributed under the terms and conditions of the Creative Commons Attribution license (CC BY).
ORIGINAL RESEARCH
Polymorphism of the dtxR gene in the currently existing strains of Сorynebacterium diphtheriae
1 G. N. Gabrichevsky Research Institute for Epidemiology and Microbiology, Moscow, Russia
2 Pirogov Russian National Research Medical University, Moscow, Russia
Correspondence should be addressed: Andrey Viktorovich Chaplin
ul. Ostrovityanova, d. 1, Moscow, Russia, 117997; moc.liamg@kidemoloko
Funding: this study was conducted as part of two projects: The Study of the Role of Microbial Communities in Human Oropharynx and Blood in Diphtheria, Pertussis and Other Infectious Inflammatory Diseases (Project ID А16-116021550311-2) and The Development of Molecular-Genetic Methods for Laboratory Diagnosis of Diphtheria and Pertussis (Project ID АААА-А16-116101810127-7) supported by the Sectoral Research Program of the Russian Federal Service for Surveillance on Consumer Rights Protection and Human Wellbeing (Problem-oriented Research in Epidemiological Surveillance of Infectious and Parasitic Diseases in 2016-2020).
Contribution of the authors to this work:Chagina IA — analysis of literature, data collection and interpretation, drafting of a manuscript; Perevarova YuS, Perevarov VV — analysis of literature, data analysis and interpretation, drafting of a manuscript; Chaplin AV — research planning, data analysis and interpretation, drafting of a manuscript; Borisova OYu — research planning, data collection and interpretation, drafting of a manuscript; Kafarskaya LI — data interpretation, drafting of a manuscript; Afanas’ev SS, Aleshkin VA — research planning, drafting of a manuscript. All authors participated in editing of the manuscript.
In spite of successful vaccination strategies, sporadic cases of diphtheria still occur, and the infection remains a serious health issue. Virulence of Corynebacterium diphtheriae is associated with its ability to produce a diphtheria toxin encoded by the tox gene [1]. Its pathogenic mechanism is based on ADP ribosylation of the elongation factor 2 that disrupts protein synthesis in human cells [2]. It should be noted that the presence of the tox gene in the C. diphtheriae genome does not necessarily confer toxigenicity. There are nontoxigenic tox-bearing strains (NTTB strains) that have lost their ability to synthesize a fully functional toxin following a series of mutations [3, 4].
Although tox is a part of the phage genome, iron-mediated regulation of toxin expression is exerted by the iron-sensing regulator DtxR, the product of the chromosomal gene dtxR. Thus, tox transcription directly depends on iron homeostasis, as low iron levels trigger tox expression followed by synthesis of the diphtheria toxin [5].
The dtxR gene is present in both toxigenic and nontoxigenic strains [6] meaning that it has functions other than regulation of diphtheria toxin synthesis. The DtxR regulon is reported to contain 20 more loci, including genes responsible for iron metabolism. To date, the DtxR protein of C. diphtheriae is known to regulate siderophore synthesis, a high-affinity transport system (ciuABCDEFG) and transcription of 3 loci involved in heme-monooxygenase (hmuO) activity [1, 7]. DtxR may also have a role in regulating bacterial virulence [7].
Microorganisms need large amounts of iron which is not so easy to acquire. However, iron excess stimulates production of toxic reactive oxygen species. Mammals have developed a mechanism of nonspecific defense against infections that relies on reducing the levels of unbound iron by specific iron-binding proteins [8, 9].
Therefore, survival and dissemination of the pathogen in the host depends on its ability to acquire different metal ions from protein complexes. For that, the pathogen employs various uptake mechanisms. Gene expression is controlled by metalloregulatory proteins — highly conserved transcriptional regulators [10, 11]. Once they bind to a specific metal ion, these regulators change their conformation and trigger or repress binding of the active site to the gene operator [12].
DtxR is a typical example of a metalloregulatory protein. Crystallography demonstrates that in its inactive state DtxR is a monomer that consists of two domains. A large conserved N-terminal domain contains two binding sites for iron ions and a helix-turn-helix motif that can bind to DNA; a smaller, less conserved C-terminal domain resembles the SH3 domain of eukaryotes. Ferrous ions bind to the binding sites rendering the repressor active. Once it is activated, dimerization occurs [13].
The two DtxR domains are linked by a proline-rich peptide segment. When the repressor is inactive, this segment binds to the SH3-resembling domain resulting in the formation of a prolylpeptide-SH3 complex (Pr-SH3) and stabilizing the repressor in its inactive state. After ferrous ions bind to the N-terminal domain triggering DtxR activation, the Pr-SH3 complex dissociates and the proline segment stabilizes helical segments of the N-terminal domain, which leads to dimerization of two protein subunits [14].
Considering the role of DtxR in C. diphtheriae survival, the dtxR gene must be studied to evaluate the pathogenic potential of C. diphtheriae, elucidate dynamics of circulating strains and assess feasibility of dtxR as a target for the PCR-based diagnosis of diphtheria or other infections associated with nontoxigenic C. diphtheria strains. The aim of this work was to identify genetic polymorphisms of DtxR and to analyze the population structure of C. diphtheriae strains circulating in Russia.
METHODS
We studied genotypic characteristics of 45 strains of C. diphtheriae (bv. gravis and bv. mitis) isolated in 2010–2015. The study was conducted at the Reference center for Measles, Parotitis, Rubella, Pertussis, and Diphtheria of Gabrichevsky Moscow Research Institute of Epidemiology and Microbiology. C. diphtheriae strains were obtained from bacterial laboratories of the institutions for disease prevention and centers for hygiene and epidemiology located in 14 different regions of Russia, where the strains had been isolated for diagnostic or preventive screening or for the purpose of epidemiological research. The following collection strains were used: C. diphtheriae (State Research Center for Applied Microbiology & Biotechnology, Obolensk, Russia) and C. diphtheriae PW 8 (Therapeutic Products Regulatory Research Center, Moscow, Russia). Besides, the following strains were used as PCR negative control, 1 strain per species: C. ulcerans, C. pseudotuberculosis, C. amycolatum, C. glucuronolyticum, C. xerosis, C. afermentans subsp. afermentans, C. afermentans subsp. lipophiium, C. coyleae, C. pseudodiphtheriticum, C. macifaciens, C. simulans, and C. durum from the collection of Gabrichevsky Research Institute.
The strains were isolated following the guidelines of the Laboratory Diagnosis of Diphtheria manual (Guidelines 4.2.698-98 and 4.2.3065-13). The isolates were seeded onto the solid tellurite blood agar base containing 2 % agar (Microgen, Russia), 10 % bovine blood (LeiTran, Russia), and 0.02 % potassium tellurite (State Research Center for Applied Microbiology & Biotechnology, Russia). Then the cultures were thermostated for 24–48 h at 37 °C. Morphological, toxigenic and biochemical profiles of the grown cultures were prepared according to Guidelines 4.2.698-98 and 4.2.3065-13 mentioned above using the biochemical test system DS-DIPH-CORYNE (Diagnostic Systems, Russia).
Chromosomal DNA was extracted by boiling from a freshly grown 24-hour old C. diphtheriae culture. A culture sample was picked up with a sterile loop and suspended in 100 µl of deionized water, incubated for 20 min at 95 °C and centrifuged. The supernatant was used for the PCR assay.
PCR amplification of the dtxR gene of C. diphtheriae was performed using a pair of primers to cover the entire region of the studied gene: a previously proposed F1 5’-GGGACTACAACGCAACAAGAA-3’ [15] and R1 5’-TCATCTAATTTCGCCGCCTTTA-3’ designed by the PerlPrimer application, v1.1.21 [16]. Specificity of primers was checked using BLASTn by comparing their sequences to similar sequences of other Corynebacterium species obtained from the NCBI Nucleotide database.
The reaction mix contained a PCR buffer with 10 mM Tris-HCl (pH 8.3), 50 mM KCl, 1.5 mM MgCl2, 0.2 µM of each primer, 200 µM of each dNTP, 1 µL of the DNA solution, and 1 unit/50 µL Taq DNA polymerase (Fermentas, Lithuania). Amplification was performed in the Tertsik amplifier (DNA Technology, Russia) operated in the automatic mode The amplified fragments were analyzed by 1.5 % agarose gel electrophoresis. Sequencing of the obtained fragments was performed by Evrogen, Russia.
C. diphtheriae strains were genotyped by multilocus sequence typing (MLST) according to the international protocol [17] using fragments of sequences of 7 housekeeping genes, namely atpA (encodes the α-subunit of ATP synthase), dnaE (encodes the α-subunit of the DNA polymerase III holoenzyme), dnaK (encodes the Hsp70 chaperone), fusA (encodes elongation factor G), leuA (encodes 2-isopropylmalate synthase), odhA (encodes components Е1 and Е2 of the 2-oxoglutarate dehydrogenase complex), and rpoB (encodes the β-subunit of RNA-polymerase). Allelic profiles were identified for each strain.
The obtained sequences were compared to the nucleotide sequences published in GenBank. The sequence of the dtxR gene of C. diphtheriae PW8 was used as a reference (Genbank NC_016789.1).
Nucleotide sequences were aligned using the MUSCLE algorithm [18]. Polymorphisms were mapped to the protein structure based on the PDB data. Queries to the NCBI Nucleotide database were run using BLASTn. To identify alleles of the housekeeping genes, the PubMLST software was used. To estimate selection pressure on the dtxR gene, we applied the Nei-Gojobori method and calculated the Ka/Ks ratio, where Ka is the number of nonsynonymous substitutions per site and Ks is the number of synonymous substitutions per site [19]. Fisher’s exact test was performed on contingency tables using the AS159 algorithm [20] and R 3.3.2. Phylogenetic trees were reconstructed by neighbor-joining based on the comparison of dtxR nucleotide sequences and included sequences of C. diphtheriae PW8, C. diphtheriae NCTC 13129 and C. diphtheriae 178-01 (Genbank NC_016789.1, BX248353.1 and NZ_JZUJ01000001.1). Evolutionary distances were computed using the Maximum Composite Likelihood method [21] and scaled as units of substitutions per site. Evolutionary analysis was performed by MEGA7 v.7.0.21 [22]
RESULTS
PCR amplification revealed the presence of the dtxR gene in all studied toxigenic and nontoxigenic strains of C. diphtheriae. Besides, PCR results came out negative for all allied species.
The samples of 45 C. diphtheriae strains were sequenced and the obtained sequences were compared to the dtxR sequences retrieved from GenBank revealing polymorphisms at 18 positions: 66, 126, 225, 273, 358, 402, 440, 474, 504,507, 516, 558, 564, 579, 639, 640, 654 and 685 (tab. 1).
The majority of substitutions relative to the reference sequence were synonymous. Specifically, the most frequent single nucleotide substitution at position 273 of the dtxR gene did not affect the protein sequence. This substitution was observed in 14 strains.
Polymorphisms at positions 440 and 640 that resulted in A147V and L2141substitutions, respectively, seemed to have no significant effect on the DtxR function. According to the 3D protein structure published in PDB (ID 2QQ9), amino acid at position 147 is found in the unstructured proline-rich (Pr) region. Since this segment participates in protein dimerization, we cannot rule out a possible effect of the amino acid substitution on DtxR activation. It appears that substitution of leucine for isoleucine at position 214 of the C-terminal domain does not have any effect on protein folding because these amino acids have similar properties. Thus, nonsynonymous substitutions are very likely to produce no effect on the DtxR function.
Many of the identified sequence variants are well known and were described previously by other researchers. However, we were able to identify a new single nucleotide polymorphism at position 358 that also has no effect on the amino acid sequence. A nucleotide query to the NCBI Nucleotide database performed by BLASTn returned no results.
We observed various combinations of polymorphisms in the dtxR gene relative to the reference sequence. Based on the discovered combinations of nucleotide sequences, C. diphtheriae isolates were distributed into several groups (tab. 2).
More than a half (55 %) of identified nucleotide sequences differed from the reference sequence. It should be noted that the new substitution at position 358 was observed in one strain only (group 5) that had the least number of substitutions compared to the reference sequence, including nonsynonymous polymorphisms.
Based on the alignment of dtxR sequences, we constructed a phylogenetic tree (see the figure).
The branching order is an approximation to some extent, due to the similarity of the analyzed sequences and hence a weak phylogenetic signal. The tree in the dendrogram is not rooted due to the lack of possibility to select an appropriate outgroup.
We also analyzed correlations between group composition and toxigenicity, biovars and sequence types (ST) determined by MLST (tab. 3). Profiles of NTTB strains were previously described in [23].
Based on the distribution of toxigenic and nontoxigenic strains of different biovars with regard to the allelic variants of dtxR, Fisher’s exact test was performed. The test was performed on 2 × 7 contingency tables to examine the association between a biovar type and the allelic variant of dtxR (p = 0.00078) and on 3 × 7 contingency tables to examine the association between toxigenicity and the allelic variant of dtxR (p = 2.8∙10−9). The obtained results prompt us to conclude that the associations between a biovar type/toxigenicity and the allelic variant of dtxR are not accidental and implicate a phylogenetic signal — a sum of associations between the allelic variant of the gene and biological characteristics of the strains. Distribution of sequence types was nonuniform, identical sequence types were rarely found in one group.
To assess selection pressure on the dtxR gene, we calculated the Ka/Ks ratio (0.0526). The obtained value (Ka/Ks < 1) indicates a strong negative selection, i. e., selection pressure is aimed at maintaining the current protein sequence [24].
DISCUSSION
Our study confirmed the essential role of DtxR in the viability of toxigenic and nontoxigenic strains of C. diphtheriae. The observed polymorphisms provide new information of the variability of its strains in Russia. dtxR–related nucleotide and amino-acid substitutions were studied previously under various conditions with regard to diphtheria dissemination [25, 26, 27]. This work was conducted against the background of sporadic incidence. We performed a comprehensive analysis of nucleotide sequences and assessed their correlation with strain toxigenicity, biovars and sequence types. This approach allowed us to better understand the structure of the population of currently circulating C. diphtheriae strains.
The hypothesis about the significant effect of horizontal gene transfer on the structure of C. diphtheriae population was discussed earlier [17]. There are works describing transfer mechanisms for genes conferring antibiotic resistance [28] and virulence [29]. Components of the DtxR regulon may vary in different strains of C. diphtheriae due to the loss, acquisition, or partial deletion of genes responsible for iron provision and hence tox expression [1, 30]. The discovered correlation between a sequence type and the allelic variant of dtxR proves the idea that homologous recombination in C. diphtheriae does not completely block the phylogenetic signal [17]. At the same time, the analysis of the population structure did not reveal any direct correlation between a biovar and the dtxR allele meaning that there is no phylogenetic basis for such classification, which is mainly determined by the frequency of horizontal gene transfer [31].
Less than a half (45 %) of the studied sequences were found to be identical to the reference sequence. Of 13 polymorphisms, only 1 was identified as new (position 358), but it did not affect the amino acid sequence. The most frequent was the synonymous polymorphism at position 273 observed in 14 strains. Our work demonstrates that Russian strains carry a smaller range of variants of the primary DtxR structure [26, 27].
It should be noted that although dtxR affects bacterial resistance to oxidative stress, under normal conditions it is not a critical gene for C. diphtheria [32], as was proved in the experiment with the mutant DtxR-defective strain [33]. However, we did not observe any significant changes in the studied sequences that could result in the synthesis of a functionally inactive protein. There were two nucleotide polymorphisms that did result in amino acid substitutions, but the analysis of PDB data showed that those substitutions did not affect protein folding. Perhaps, functionally important polymorphisms of the dtxR gene may impair strain adaptation to the mammalian host limiting distribution of alleles that lead to the synthesis of the defective protein among the C. diphtheriae population. The value of the Ka/Ks ratio proved that DtxR is controlled by the stabilizing selection.
The pathogenic potential of C. diphtheriae does not always depend on strain’s ability to produce the diphtheria toxin. For example, nontoxigenic strains of C. diphtheriae are becoming an increasing source of severe infection causing endocarditis [34], arthritis [35] and osteomyelitis [36]. Nontoxigenic strains are especially dangerous for patients with compromised immunity [37, 38]. This necessitates a more comprehensive approach to C. diphtheriae identification as currently existing methods are aimed at detecting toxigenic strains only.
Our findings confirmed the presence of the dtxR gene in both toxigenic and nontoxigenic strains of C. diphtheriae proving the feasibility of PCR-based identification proposed earlier [39]. Sequencing demonstrated that polymorphisms occurred mainly in the C-terminal domain of DtxR [15]. However, nucleotide substitutions were also observed in other gene regions (positions 66, 126, 225, 273, and 358). One of such substitutions (position 126) was found in 5 strains (group 2) in the region corresponding to the primer that had been proposed earlier for PCR- dtxR [15, 39]. The pair of primers used in this study proved their high specificity confirmed by zero false-positive results. This, PCR-based identification of C. diphtheriae is a promising technique for the diagnosis of diphtheria and infections associated with nontoxigenic strains.
CONCLUSIONS
We analyzed the structure of C. diphtheriae population based on the allelic variants of the dtxR gene. The analysis revealed that 55 % of strains had sequences different from the reference sequence. The majority of the discovered polymorphisms were synonymous. The absence of wild strains with defective DtxR and a high similarity of the analyzed nucleotide sequences indicate a strong negative selection aimed to maintain the currently existing repressor sequence.
Homologous recombination attenuates the phylogenetic signal but does not block it completely. We discovered associations between the allelic variants of dtxR and toxigenicity/ biovar type. The obtained results allow us to conclude that dtxR represents a conserved sequence. We recommend PCR-dtxR as an accurate method for identification of toxigenic and nontoxigenic strains of C. diphtheriae.