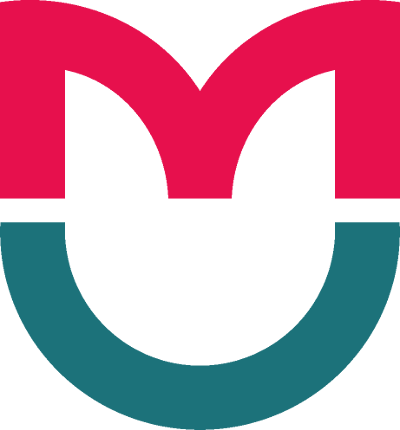
ORIGINAL RESEARCH
Bioluminescence: is it possible for a plant?
1 Laboratory of Chemistry of Natural Compounds,Pirogov Russian National Research Medical University, Moscow, Russia
2 Total Synthesis Laboratory,Shemyakin–Ovchinnikov Institute of Bioorganic Chemistry of the Russian Academy of Sciences, Moscow, Russia
3 Moscow South-West High School No. 1543, Moscow, Russia
Correspondence should be addressed: Elena Guglya
ul. Ostrovityanova, 1, Moscow, Russia, 117997; moc.liamg@aylguge
Funding: this work was supported by the Russian Science Foundation (Grant No. 14-50-00131).
Acknowledgements: the authors thank Lyudmila Abramova and Nikita Tikhomirov for their assistance in collecting and sorting plant samples. The present research was carried out at the facilities of the Shared Resource Center of Shemyakin and Ovchinnikov Institute of Bioorganic Chemistry.
Contribution of the authors to this work: Guglya EB — preparation of organic extracts of plant samples with BL activity, development and implementation of chromatographic separations, BL analysis, drafting of a manuscript; Kotlobay AA — preparation of organic extracts of plant samples during screening of plants collection, preparation of enzyme extract, BL analysis; Sekretova EK, Volkova PV — collection and taxonomic definition of a collection of plant samples; Yampolsky IV — research planning and organization, data interpretation, editing.
Bioluminescence (BL) is the emission of visible light by living organisms. The phenomenon was demonstrated in vitro more than a century ago by Raphaël Dubois [1]. He mixed “cold” and “hot” extracts obtained from the light organs of the beetle Pyrophorus noctilucus. The extract prepared with cold water comprised a heat-labile enzyme luciferase and the hot water extract contained a heat-stable luciferin. Therefore, light emission from the mixture of the two extracts was the result of a substrate-enzyme reaction. Bioluminescence as a term was first used by Harvey [2]. In all known BL systems oxygen is required for the reaction producing the oxidized luciferin. Relaxation of oxyluciferin from the excited to ground state is accompanied by light emission.
BL is widely spread among animal and fungi kingdoms, but not a one luminescent plant is known in nature [3]. The first attempt for a glowing plant engineering was undertaken more than 30 years ago [4]. Based on the well-studied BL system of the firefly, the bioengineered plant Nicotiana tabacum was obtained by inserting the luciferase gene. When mixing an extract of the plant with a solution of luciferin and ATP, or when immersing the intact plant in the same solution, BL light was detected. An image of the luminous plant was demonstrated by the exposure on an X-ray film. Later some more attempts were performed [5, 6] and even a project on the creation of a luminous plant was announced [7], but no fundamentally new achievements were obtained. Our recent investigation on BL of higher fungi allowed us to believe in the possibility of more successful results using the features of this enzyme-substrate system.
BL systems are very specific within animal taxa whereas higher fungi share a uniform BL mechanism [8]. Fungal BL was considered as a two-step process first in 1961 [9]. A luciferin precursor is reduced by an NAD(P)H-dependent enzyme to a true luciferin and then luciferin is oxidized by air under luciferase catalysis to produce visible light at 520–530 nm [10]. After multiple unsuccessful attempts [11, 12] luciferin precursor was isolated from the fruiting bodies of nonluminous fungus Pholiota squarrosa and recognized as hispidin (6-(3,4-dihydroxylstyryl)-4-hydroxy-2-pyrone) [13] — a well-known fungal and plant secondary metabolite [14] (tab. 1). Further, hispidin was enzymatically converted into luciferin whose structure has been established as 3-hydroxyhispidin [13] (fig. 1).
In the literature, there is a number of reports on isolation of hispidin and its derivatives from plants, including Alpinia zerumbet [15, 16, 17], Pistacia atlantica [18], Peganum harmala [19], Pteris ensiformis Burm [20], Cassia alata [21] , Rheum tataricum [22] and others (tab. 1). The wide occurrence of hispidin among fungal and plant species, along with the existence of similar compounds among plant metabolites have logically led us to an idea of challenging the possibility of engineering the self-luminescent plant by introducing a luciferase gene in a plant capable of biosynthesizing its substrate. Like other plant metabolites, hispidin derivatives are in the focus of modern investigations due to possible pharmaceutical applications. Hispidin and its derivatives are characterized by many bioactivities such as antioxidant, anti-cancer, anti-obesity and are regarded as potential leads for drug development [16, 17, 18, 19].
The purpose of this work was screening for the substrate(s) of fungal BL reaction in the extracts from the range of plants occurring in the European part of Russia and isolation of active compound(s).
MATERIALS AND METHODS
Plant extracts
All plant samples were collected in Tver region of Russia in June 2015 (tab. 1 in SI). Only leaves were collected. All samples were frozen and stored at –70°C, some of them were dried in vacuo.
Extraction of plant biomass is a well-designed technique [23]. Acetone was chosen for the initial screening of about 200 samples. Other solvents (methanol, ethanol, acetonitrile) were also tested for optimization of the extract conditions.
For preparation of a plant extract a piece of leave (30–500 mg) and 0.3–1.5 mL of a solvent were shaken in a 1.5 mL tube for 20 min on BioShake XP shaker at 1800 rpm at room temperature. The extract was centrifuged (5 °C, 10000 rcf on a 5424R Eppendorf centrifuge), and the solid residue was discarded. The extract could be further used for SPE or HPLC separation or, alternatively, dried in a vacuum centrifuge MiVac (SpScientific). For further operations dried residues were redissolved in an appropriate solvent and centrifuged. The solvent composition for the dried residue could be different from the solvent in the first extraction, in particular, a buffer solution was added to an organic solvent.
SPE technique with C18 pre-packed cartridges (500 mg, Phenomenex) was used: 0.5–1 mL of initial extract was eluted followed with 1 or 2 mL acetonitrile–water mixture 1 : 1, acetonitrile and acetone in this order.
Preparation of fungal luciferase is described in detail in our previous publication [13].
Bioluminescence assay
The bioluminescence assay comprised two components: fungal luciferase and plant organic extract. A diluted fungal enzymatic extract (3 µL) and a plant organic extract (3, 10 or 15 µl) were added to 100 μL of 0.2 M phosphate buffer, pH 7.5 with 1 mM DTT, in a test tube, immediately shaken and placed in a cuvette of a luminometer (Glomax 20/20, Promega). Luminescence was measured for 10 s with integration time 1 s (tab. 2). Extracts from leaves, extracts of their dried residues of the first extracts, fractions after SPE or the fraction after HPLC were tested as organic extracts containing a potential substrate.
HPLC separations
were performed on Nexera X2 (Shimadzu) instrument equipped with autosampler SIL-30AC, diode-array detector SPD-M20A and fraction collector FRC 10A. Columns of different types were applied (tab. 3). Columns and detector were maintained at room temperature, autosampler temperature was 5°C. Mobile phase: buffer A — 0.02 M ammonium acetate pH 5.5, buffer B — acetonitrile or methanol or acetone (mobile phase composition and gradient see in tab. 3). Solvents were of HPLC grade.
RESULTS
To discover a luciferin-like substrate in taxonomically diverse plants samples, the first task was a screening of the 200 species collection. Each plant extract was mixed with the fungal enzyme solution and luminescence was immediately measured. Totally, in 10 cases the luminescence (tab. 4) was observed. For the initial screening we used only acetone for extraction. The composition of the solvents varied to achieve the maximum luminescence of the extracts with activity found. As a result, some quantitative, but not qualitative differences in BL values were observed. Drying the extract and redissolving of the residue in a smaller volume allowed to concentrate the sample and to increase the BL values. A buffer solution with a certain pH value was added to the organic solvent in some cases, the pH value being critical for the preservation of the extract activity. Thus, the following conditions were the best for frozen leaves of P. natans: acetone extraction from leaves material (first extraction) and with mixture 7 : 3 of acetone and aqueous acetate buffer pH 6.5 for redissolving the dried residue (fig. 2).
The extracts of all active species were tested for stability. They were dried and dissolved again, then exposed for some hours at 0 °C or at room temperature. Measurements of BL activity after each operation demonstrated that all the substrates were unstable (tab. 4). Loss of activity during the drying process was 50 % and more. The highest activity (observed for P. natans extract) decreased by an order after exposure at room temperature.
When exposing the extracts on ice evident increase of luminescence was observed during some hours. This effect was noticed for many species, and particularly a 10x increase was observed for R. nigrum extracts (tab. 4, Fig. 3, A). The dependency of activity on the exposure time varied not only between species but also between initial extracts and SPE fractions of the extracts. The extracts from dried leaves of B. pendula seemed to be stable (fig. 3, B), while the extracts from fresh and frozen leaves showed a significant growth of BL (fig. 3, C). Furthermore, 10-s curves of the BL measurments for B. pendula extracts were often (but not always) of rising type instead of a usually observed declining type (fig. 4).
Based on the highest value of initial luminescence, we chose P. natans as a first candidate to continue with HPLC separation of the BL substrate. Mobile phase conditions for HPLC separation of the extracts were optimized as follows: buffer A 0.02 M aqueous acetate buffer at pH 5.5, elution with gradient buffer B content up to 95 %. HPLC fractions were dried in vacuo, dissolved again with 20 times concentration and their luminescence activities were measured (fig. 5). We observed two zones in the HPLC profile, which comprised active components, along with many components with different UV spectra, indicating that the extract contained more than one substrate and the components were not completely separated.
Looking for isolation of higher amounts of the unstable substrates we varied our technique and tried other species. Fig. 6 represents the results of these attempts. B. pendula was chosen as a source. Extract of fresh leaves (400 mg in 1500 µL of methanol, 1000 µL of extract taken) was fractionated on C18 cartridges. Activities of the fractions were measured and 800 µL of the most active fraction was loaded without drying onto a semi-preparative C18 column. Assaying BL activity of the fractions revealed many active zones on a 45 min chromatogram. It can be mentioned, that green color (absorption around 650 nm) always accompanied the activity. We could ascertain the presence of many unresolved peaks in the most active fractions at the beginning of the chromatograms, some active fractions at the end and the absence of correlation of major chromatographic peaks with activity. Second step separation of some fractions on the same column was performed, but activities disappeared due to chemical instability.
In another attempt we used acetone as an unusual mobile phase component. Acetone is not very suitable for UV detection in lower UV region (300 nm and less). However, we supposed that active BL substrates should probably absorb in the region above 300 nm, making possible the use of acetone. The chromatogram and the activity profile are shown on fig. 7. The profile indicated the presence of several active components. The activities and the amounts of active substances were not high enough to perform the next chromatographic step.
In another attempt we applied faster separation of P. natans extracts on a polar column as a first step combined with separation of the most active fraction on a reverse phase column as a second step (fig. 8). To prevent activity losses extracts and fractions were not dried before BL measurements. The most active 500 µL fraction (between 3 and 3.5 min) from the first column was concentrated to 100 µL and loaded onto the second column. We could reveal some active fractions after the second separation. Two peaks were distinguished on the cromatogram in the region of 11.5 and 12.0 min but separation was not complete and the activities were low.
We estimated the losses of the activity at each stage of the separation by BL measurement (tab. 5). Total activity of the solutions was calculated for initial extracts and fractions after separations. These data showed that the losses occurred at each operation. As a result, only 0.2 % of the initial activity remained after final step. Thus, in all cases activity losses during separation did not allow us to isolate any individual substance for further characterization.
DISCUSSION
The study of 10 plants containing BL active compounds showed that our hypothesis about the presence of hispidin or 3-hydroxyhispidine in the leaves samples was wrong. In all cases NAD(P)H was unnecessary for the luminescence reaction, indicating similarity of the active compound to fungal luciferin, but not to hispidin. We applied the same chromatography conditions as those previously developed for isolation of the fungal luciferin [13]. However, activity profiling of HPLC fractions showed no activity and therefore the absence of 3-hydroxyhispidin.
The presence of many active fractions after chromatographic separation of the organic extracts indicates that each plant synthesizes not one but many substrates for the fungal luciferase. In addition, a variety of chromatographic conditions for separation of different plants extracts showed the diversity of their active compound sets. Anomalous increase of bioluminescent activity during exposure of organic extracts on ice suggests the possibility of chemical reactions resulting in decrease of some active compounds concentration and increase in the concentration of others. Unfortunately, no stable substrates could be detected, as the luminescence of the extracts of all 10 plants decreased at room temperature.
We have applied many modifications for organic extracts preparation and their HPLC separation to satisfy the contradictory requirements of maximum recovery, maximum stability and selective isolation of the individual unstable compound(s) from complex mixtures. Some extracts were fractionated with SPE prior to HPLC, and the others were immediately loaded onto the chromatography column. We tried to dry the extracts for additional concentration, the same was applied to the solutions of the chromatographic fractions before measuring luminescence. Various organic solvents were used as mobile phase components for HPLC; two-dimensional chromatography was performed on columns with different polarity.
But ultimately, the stability of all the active compounds found was insufficient to achieve the substrate isolation in an amount sufficient to establish the compounds’ structure and properties.
CONCLUSIONS
Bioluminescent plants do not exist in nature, making their creation by the means of synthetic biology a challenge. This research has revealed the ability of some plant components to react with fungal luciferase to produce luminescence. Thus, the present study may be considered as a first step to creation of self-luminescent plants. Within the species range covered in this work, all potential substrates were chemically unstable, making their isolation and structural characterization yet unsuccessful. However, the following conclusions may be considered in the future attempts: the luminescent luciferase substrates present in the plant biomass extracts are not identical to fungal luciferin (3-hydroxyhispidin); the species tested produce many different active substances. Probably, future work towards self-consistent bioluminescent plants should focus on finding the genes responsible of 3-hydroxyhispidin biosynthesis and expression of these genes in a transgenic plant together with fungal luciferase.