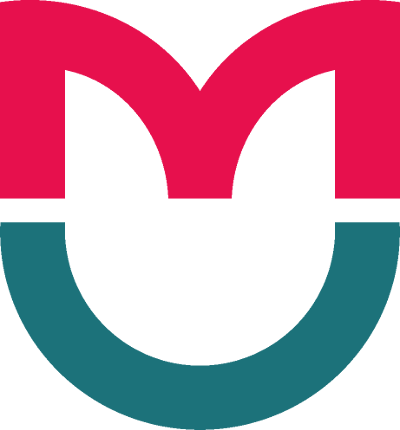
OPINION
Serine/threonine protein kinases of bacteria are potential targets for regulation of human microbiota composition
1 Laboratory of Bacterial Genetics, Vavilov Institute of General Genetics of RAS, Moscow
2 Department of Bioinformatics, Faculty of Biological and Medical Physics,Moscow Institute of Physics and Technology (State University), Dolgoprudny
Correspondence should be addressed: Natalia Zakharevich
ul. Gubkina, d. 3, Moscow, Russia, 119991; ur.xednay@hciverahkaz
All authors' contribution to this work is equal: selection and analysis of literature, planning of the manuscript's structure, data interpretation, drafting of the manuscript, editing.
Interactions between the gut microbiota and the host
The human microbiota is a microbial community that inhabits various body surfaces, such as the skin, the genitourinary system or the gastrointestinal tract (GIT), etc. Shaped by evolution, it has become an essential body organ. Our relationships with the microbiota are symbiotic. About 60 % of our symbionts live in the large intestine constituting the largest microbial population of the body [1, 2]. The functions and the composition of the microbiota depend on age, sex, diet, environmental factors and social conditions, individual’s health and the use of medications, including antibiotics [3, 4].
The microbiota in general and the gut microbiota in particular can significantly affect human health. Being the largest microbial reservoir of the body, the microbiota of the small and large intestines can influence the function of the GIT and other vital organs and systems [1, 5]. The gut microbiota forms a barrier against pathogens, maintaining colonization resistance of the host. The well adapted bacteria have developed this capacity through a continuous interaction with the human host under strong selection pressure. The gut microbiota can also activate the immune, endocrine and nervous systems of the host, including the brain. The immunomodulatory role of the microbiota is largely determined by the presence of bifidobacteria and lactobacilli [1, 6]. Residents of the gut flora produce bioactive substances, such as vitamins and hormones, participate in the metabolism of proteins, lipids, carbohydrates and nucleic acids, and regulate the composition of the intestinal gas [4].
Imbalances in the gut flora called dysbiosis are characterized by the increased abundance of potentially pathogenic microorganisms (pathobionts) [2, 7] and can lead to metabolic diseases, inflammation and visceral pain or induce changes in the central nervous system (CNS), which, in turn, can cause behavioral and cognitive disorders [2, 8, 9, 10]. Shifts in the microbiota composition were shown to accompany obesity, allergy, type 2 diabetes, cardiovascular and autoimmune diseases [2, 11]. The range of factors that negatively affect the composition and functions of the microbiota is continuously expanding; among them are environmental pollution (life in a megalopolis) [12], poor diet (the growing popularity of fast food) [13], and stress [14]. It is also known that dysbiosis can result from antibacterial therapies that kill the native microbial flora and therefore stimulate the unnatural selection of antibiotic-resistant pathogens [1].
A symbiotic relationship with bacteria is very important for the host. Highly responsive to a variety of factors, the microbial population of the GIT is a sensitive indicator of the host’s health [1, 4]. Therefore, the microbiota could serve as a potential therapeutic target for the treatment/management of different pathologies, including dysbiotic conditions. Various approaches and strategies are used to regulate the human microbiome: diets, intake of pro- and prebiotics, and surgical procedures. Some authors indicate that physical activity has a positive effect on the microbiota when combined with a balanced diet [15, 16]. In this work we propose the use of selective inhibitors that target certain bacterial genera or species and therefore suppress bacterial growth, reduce virulence of pathogenic and opportunistic microbes and contribute to the restoration of the taxonomic balance of the gut microbiota. Eukaryotic type serine/threonine protein kinases (STPKs) can serve as a target for such inhibitors.
Classification of STPKs of gram-positive bacteria
Phosphorylation and dephosphorylation of proteins are the major molecular mechanisms that regulate processes inside the cell. An average bacterial phosphoproteome contains about a hundred of phosphorylation sites. An exception here is the phosphoproteome of Mycobacterium tuberculosis with about 500 sites [17]. For eukaryotic proteomes, the number of phosphorylation sites is an order of magnitude higher [18].
Serine/threonine protein kinases of both bacteria and human are an essential component of signal transduction [19, 20]. Eukaryotic type STPKs were first identified in bacteria [21, 22, 23, 24, 25] about 20 years ago. To date, we know that they are involved in the regulation of cell division and growth [26, 27, 28], biofilm formation [29], response to oxidative stress [30], and sporulation [31]. STPKs play an important role in the development of virulence and pathogenicity in bacteria [21, 32, 33]. They also participate in the pathogenesis of microbial diseases caused by M. tuberculosis, Streptococcus pneumoniae, Staphylococcus aureus, and other microorganisms [20, 25].
STPK inhibitors are ATP-competitive. Therefore, it appears tempting to employ selective inhibition of STPKs of pathogenic and opportunistic microorganisms by ATP-competitive inhibitors for the restoration of the compositional and functional balance of the human microbiota
Previously, we proposed a classification of eukaryotic type STPKs of gram-positive and gram-negative bacteria [19]. We analyzed a number of microbial STPKs found in pathogenic and non-pathogenic Mycobacterium, Staphylococcus, Actinomyces (and some others) and in probiotic members of the symbiotic human microbiota, namely Bifidobacterium and Lactobacillus. Our classification was based on the physiochemical properties of 9 amino acid residues that line the surface of the adenine-binding pocket. The amino acid residues were selected based on the visual analysis of 3D structures of bacterial STPKs available in the Protein Data Bank [34]. Amino acid sequences of bacterial STPKs were analyzed in parallel; we also performed multiple alignment of all sequences of the studied bacterial protein kinases [19].
The analysis of STPKs’ structures identified amino acid residues that interact with ATP. We were particularly interested in the residues of the adenine-binding pocket, since this region is more variable than phosphate- and ribose-binding sites. Conserved residues and residues that contribute to ATP binding only with their backbone atoms were excluded from consideration, since they were of no practical value for our classification. While analyzing the STPKs of gram-positive bacteria, we disregarded highly conserved amino acid residues typical for eukaryotic and bacterial protein kinases, but instead focused on the substitutions in the positions that are conserved in eukaryotes and do not affect the functional activity of bacterial kinases. Of particular interest was catalytic subdomain V (STPKs have 12 conserved subdomains that are typical for both eukaryotic and bacterial STPK [35, 36]). Compared to other subdomains, the V-subdomain of eukaryotic protein kinases is quite variable and does not contain any conserved motif. However, this domain harbors amino acid residues that constitute the hinge region linking 2 lobes of a catalytic domain. By changing orientation of the catalytic and protein-binding regions, STPK activity can be regulated. Therefore, the V-subdomain, or, to be more precise, the hinge sequence can be a basis for STPK classification.
To sum up, we visually analyzed the structures of STPKs and their amino acid sequences and selected 9 variable amino acid residues whose side chains are exposed into the inside of the adenine-binding pocket: Leu17, Val25, Ala38, Val72, Met92, Tyr94, Val95, Met145, and Met155 (the residues are numbered according to the PknB sequence of M. tuberculosis) (fig. 1).
The residues we selected constitute a signature sequence for the adenine-binding pocket. Conservation of the studied signature residues varied. Based on the analysis of the signature formed by 9 amino acid residues, we drafted a classification of STPKs of gram-positive bacteria. According to the proposed classification, all studied kinases were organized into 20 groups (fig. 2). Of note, the main criterion for grouping was the presence of a specific combination of a hydrogen bond donor/acceptor and aromatic residues in specific positions of the adenine-bonding pocket signature. Of 20 groups, 13 were species-specific. A few of the remaining 7 groups were characteristic of pathogens only [19]. Thus, the STPK classification allowed us to organize the studied bacterial genera and species into groups. In each group the configuration of the adenine-binding site (shape, volume, and depth) was specific, therefore we hypothesized that selective inhibitors targeting kinases from one group will not interact with (or will weakly interact with) kinases from other groups. In this light, our classification may find its practical application in the development of ATP-competitive inhibitors of bacterial eukaryotic-type STPKs.
Practical application of the proposed classification: selective inhibitors of STPKs
The composition of the gut microbiota is being actively studied. According to preliminary estimates, the large intestine is home to over 400 bacterial species that belong to a few taxonomic groups [37, 38]. As a rule, the gut microbiota of an adult human is dominated by Firmicutes, Bacteroides, Actinobacteria and Proteobacteria [39]. The microbial community seems to be unique for each individual [37, 40, 41] and can undergo significant changes due to exposure to various internal and external factors.
In dysbiosis, the abundance of probiotic flora diminishes and pathogenic bacteria start to grow leading to pathology [5]. So far, the association has been established between the GIT microbiota and a number of diseases. Morgan et al. have found a correlation between imbalances in the gut microbiota composition and ulcerative colitis and Crohn’s disease [42]. While the gut microbiota of a healthy individual is dominated by 4 bacterial phyla [37, 43], the microbiota of patients with ulcerative colitis and Crohn’s disease is characterized by lower taxonomic diversity and reduced abundance of Firmicutes. Besides, in Crohn’s disease the abundance of Clostridia also changes [42, 44]. Reduced taxonomic diversity of the gut microbiota was also demonstrated in patients with psoriasis. Compositional shifts in the microbiota of psoriatic patients were associated with the increased abundance of 4 bacterial genera, including Corynebacterium, Staphylococcus, and Streptococcus [45].
The literature extensively reports on the association between different metabolic disorders and compositional changes in bacterial populations inhabiting the GIT. For example, Larsen et al. estimated differences between the gut microbiota of patients with type 2 diabetes and healthy individuals [46], revealing significantly lower abundances of Firmicutes and Clostridia and an increased abundance of Lactobacillus in diabetics as compared to healthy individuals [46]. Lactobacilli constitute a heterogenic group of microorganisms with immunomodulatory properties [47]. These properties seem to be a prerequisite of inflammation in patients with diabetes mellitus [46]. Tana et al. discovered that Lactobacilli were more abundant in patients with irritable bowel syndrome (IBS) than in the controls [48]. However, the presence of certain strains of Lactobacillus is very beneficial for patients with Crohn’s disease and ulcerative colitis. The analysis of inflamed mucosa samples collected from such patients revealed the presence of some microorganisms (including Lactobacillus and Bifidobacterium) that can actually protect the intestinal mucosa from inflammation. The explanation here is that some Lactobacillus strains, in particular L. casei and L. plantarum, inhibit expression of key proinflammatory cytokines and chemokines and neutralize proinflammatory effects of Escherichia coli [49].
Considering the above, our classification comes handy when there is a need to restore a normal taxonomic composition of the microbiota; this can be achieved by the selective targeting of certain groups of bacteria: Lactobacillus (XIII) [19] in patients with type 2 diabetes or IBS, and Corynebacterium, Staphylococcus and Streptococcus (IV, X) in psoriatic patients.
Besides, the majority of STPKs are key regulators of bacterial growth: their inhibition can result in suppressed microbial growth. Selective inhibition of STPKs, including the STPKs of symbionts, can alter the composition of the microbiota without killing the indigenous microbes, though their growth and activity will be slightly affected. A good example here is growth inhibition of opportunistic Actinomyces and Corynebacterium belonging to groups III and IV, according to our classification [19]. Thus, a good STPK inhibitor can correct the composition of the human microbiota.
Because of the structural similarity of ATP-binding sites in human and bacteria, there is a chance of unintended inhibition of human protein kinases. Although catalytic domains of bacterial and human kinases are only 30 % identical, human protein kinases should be taken into account when developing selective inhibitors of bacterial STPKs. The human kinome encompasses 518 protein kinases. Using multiple alignment, we established that 324 of them contain Hanks subdomains usually found in eukaryotic STPKs. To distribute human kinases into groups according to our classification scheme, we identified 9 signature amino acids in the selected 324 kinases. It turned out that human kinases can be attributed to only 4 groups: I, II, VIII, and XII (fig. 3). Of all analyzed human protein kinases, only 8.6 % ended up in “bacterial” groups. It should be noted that inhibition of unclassified human protein kinases by the compounds targeting classified STPKs is unlikely due to certain differences in their binding sites.
The need may arise to obtain selective inhibitors of STPKs of pathogens. Such inhibitors must be selective towards both human protein kinases and STPKs of microbial symbionts. Therefore, three clusters should be formed based on the groups that contain STPKs of pathogens, STPKs of symbionts; these clusters should be screened for pathogen-specific kinases. For this purpose, we built a Venn diagram (fig. 3).
Fig. 3 shows that kinases belonging to groups VII, X, XVIII, and XIX (see the table) should be considered when developing selective inhibitors of STPKs found in pathogens. These groups include only kinases of pathogens, and the inhibitors targeting these groups are very unlikely to interact with human protein kinases or STPKs of symbiotic bacteria.
Groups VII and XIX include STPKs of M. tuberculosis, namely PknK and PknI. PknK is involved in the translation control at different stages of bacterial growth, contributes to adaptation and pathogenicity mechanisms in mycobacteria [50, 51]. It was demonstrated experimentally that pknK expression is higher in the virulent strain H37Rv of M. tuberculosis than in the avirulent strain H37Ra [50]. PknI has strong homology to Stk1 of Streptococcus agalactiae promoting virulence [52]. Having said that, we assume that inhibition of these kinases will suppress M. tuberculosis growth and diminish pathogenicity and virulence of its strains.
Group X includes STPKs of Staphylococcus. Staphylococcal STPKs modulate the cell wall structure and are involved in promoting bacterial virulence [53]. Consequently, inhibition of kinases belonging to this group will reduce virulence of Staphylococcus.
Group XVIII is represented by the YbdM protein kinase of Bacillus that phosphorylates the two-component system DegS/U. In turn, this system affects biofilm formation, formation of complex colonies and microbial motility [54]. Therefore, selective inhibitors of STPKs of this group will disrupt the above mentioned processes.
Human protein kinases whose signatures coincided with the signatures of bacterial protein kinases belong to the following kinase classes: AGC[1], CAMK[2], CMGC[3], STE[4], and also to the families of yet unclassified protein kinases, such as IKK and NEK. The role of these protein kinases in the human body is varied. For example, kinases of the ROCK family participate in the Rho-induced formation of actin stress fibers and focal adhesion formation, as well as in platelet activation, smooth muscle contraction, neutrophil chemotaxis, etc. [55]. РАK kinases phosphorylate some cytoskeletal proteins and regulate their activity. Some authors indicate their role in the regulation of MAPK signal pathways in mammalian cells [56, 57]. Kinases of the NDR family were shown to have a role in embryonic development, neurological processes and cancer mechanisms [58]. However, it does not mean that inhibition of these human kinases should be avoided in every single case. For example, the increased expression of ROCK kinases is associated with a number of disorders (bladder cancer, breast carcinoma, etc.), and PAK1 kinase is associated with joint diseases (osteoarthritis, rheumatoid arthritis) because activation of certain signaling cascades in which this kinase is a major mediator leads to the increased expression of marker genes linked to osteoarthritis [59].
Thus, regulation of the taxonomic composition of the human microbiota is a complex task and requires a high level of expertise in different research fields. A good knowledge of microbial genetics and processes inside the human cells is a must. Bioinformatics and chemistry will also be involved, as huge data arrays will have to be analyzed and 3D structures built of the members of at least major kinase groups.
CONCLUSIONS
Recently researchers have come to realize that the human microbiota is crucial for human health. The most diverse and large population of microorganisms inhabits the gastrointestinal tract. Its residents have been co-evolving with human. Taxonomic imbalances in the gut microbiota can lead to pathology, including ulcerative colitis, Crohn’s disease, diabetes, etc.
Approaches to restoring the taxonomic composition of the microbiota should consider the effect and selective capacity of the compounds intended to inhibit microbial growth. It is important that correction of taxonomic imbalances should target both pathogens and symbionts. Therefore, the effect of kinase inhibitors on the gut flora should be strong enough to suppress the growth of pathogens and reduce their virulence and at the same time gentle enough to only minimally affect human protein kinases. Selective inhibitors of STPKs seem to be ideal for this purpose.
An idea of using target STPK inhibitors is not novel [20, 60, 61]. In our previous work we compared human kinases and kinases of pathogens (Plasmodium, Trypanosoma, Leishmania) and showed that in spite of quite conserved structure of the catalytic domain and binding sites in particular, creation of selective inhibitors is possible [62]. The proposed classification of STPKs is the first step towards developing effective selective inhibitors of bacterial protein kinases.
We propose the following algorithm: (1) a comparative analysis of taxonomic composition of microbiotas in healthy and diseased individuals and identification of those bacterial groups (species or genera) that are overabundant in pathology; (2) the analysis of groups that include STPKs of the identified microbes; (3) the in silico search of inhibitors ( low molecular weight compounds) of protein kinases belonging to the identified groups (modeling of 3D structures, molecular docking); (4) tests of the obtained compounds. Steps (2) and (3) imply consideration of: functions of target STPKs; non-target STPKs bacteria included into the selected groups; 3D structure(s) of a typical protein kinase representative from each group to expedite selection of good inhibitors.
[1] AGC — a class of protein kinases whose activity is regulated by cyclic GMP/AMP. This class includes the so-called protein kinases C; their activity can be regulated by diacylglycerol, phospholipids and calcium ions.
[2] CAMK — calcium/calmodulin-dependent protein kinases.
[3] CMGC — a class of protein kinases consisting of cyclin-dependent protein kinases (C), the so-called МАР-kinases (М) and enzymes that can phosphorylatie glycogen synthase (G).
[4] STE — serine/threonine kinases first identified in yeasts.