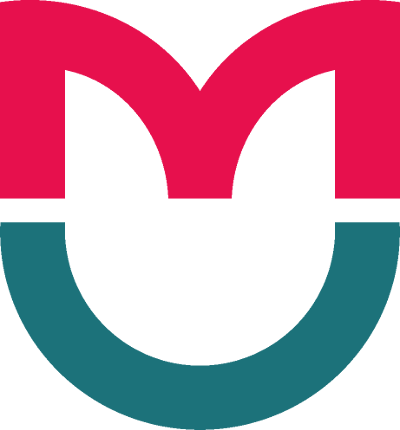
OPINION
Poly(3-hydroxyalkanoate)-based drug formulations: the micro- and nanostructure
1 Faculty of Biology, Lomonosov Moscow State University, Moscow
2 Bakh Institute of Biochemistry, Research Center of Biotechnology of the Russian Academy of Sciences, Moscow
Correspondence should be addressed: Anton P. Bonartsev
Leninskie gory, 1 bl. 12, Moscow, 119234; ur.liam@ranob_tna
Funding: this work was supported by the grant of the Russian Science Foundation (project 17-74-20104, section 1) and the grant of the Russian Foundation for Basic Research (project 15-29-04856, section 2).
The use of poly(3-hydroxyalkanoates) in the design of pharmaceutical drugs
The use of micro- and nanoparticles (NP) derived from the biodegradable polymers known as poly(hydroxyalkanoates) (PHAs) holds great promise for the design of injectable systems for sustained drug release. Immobilization of drug substances (DS) in polymer matrices composed of micro-or nanoparticles facilitates extended release of a drug and helps to maintain its optimal concentrations in a target organ or tissue for up to several months, ensuring the desired pharmacological response. Maintaining constant therapeutic yet nontoxic DS concentrations over a long period is one of the ways to overcome the adverse effects typically associated with conventional drugs, including increased toxicity, poor stability, unsteady rates of release, and the inefficient use of active ingredients in the manufacturing process. Polymer drug delivery platforms enable drug release at a target site at a predetermined rate, which is essential in treating chronic diseases. At present, the following PHAs polymers are used both in clinical practice and scientific research: a group of synthetic polymers including poly(2-hydroxypropanoic) acid (polylactic acid, PLA, or polylactide), poly(2-hydroxyacetic) acid also known as polyglycolic acid (PGA) or polyglycolide, and poly(6- hydroxycaprolactone) (PCL); and a group of naturally occurring polymers consisting of poly(3-hydroxybutyric) acid referred to as poly(3-hydroxybutyrate) (PHB), poly(4-hydroxybutyric) acid (P4HB), poly(3-hydroxyvaleric) acid (poly(3-hydroxyvalerate)), poly(3-hydroxyhexanoate), poly(3-hydroxyoctanoate), their copolymers, and polymers with a similar structure, such as poly(p-dioxanone) (PDO). The diversity of currently available PHA-based drugs is enormous; more are underway. PHAs ensure targeted delivery, extended release, reduced toxicity, and increased stability of a drug substance. Since 1981, PHA-based medications have been permeating the pharmaceutical market. Among them are poly(lactic-co-glycolic acid)-based prolonged-release dosage forms of peptide hormones for the treatment of prostate cancer (Zoladex, Lupron Depot, Trelstar, Eligard), acromegaly (Sandostatin LAR, Somatuline), and dwarfism (Nutropin depot); prolonged-release dosage forms of antibiotics encapsulated in microparticles (Atridox, Arestin); a prolonged-release antitumor medication against glioblastoma multiforme produced in the form of an implantable membrane (Gliadel); prolonged-release anti-inflammatory agents encapsulated in microparticles used for treating macular edema (Ozurdex); prolonged-release DS to fight alcoholism and drug abuse (Vivitrol); prolonged-release atypic antipsychotic DS encapsulated in microparticles for patients with schizophrenia (Risperdal Consta), and many others [1].
All PHAs possess a unique combination of properties paving the way for their application in clinical practice. PHA polymers are biodegradable: they decompose safely in the human body without producing any toxic agents. PHAs are biocompatible with human organs and tissues. They are also characterized by good thermoplasticity and have specific diffusion properties. The process of PHA production is advantageously efficient. Nevertheless, not every PHA enjoys wide use in the pharmaceutical industry. The greatest share of the pharmaceutical market belongs to synthetic PHAs, including polylactic and polyglycolic acids and their copolymers (poly(lactic-co-glycolic) acids). These copolymers are synthetic analogs of naturally occurring poly(3-hydroxyalkanoates), polyesters of 3-hydroxyalkane acids; accordingly, PHB is a linear polyester of 3-hydroxybutyric acid. Depending on the side radical, a few different P3HA types are distinguished: PHB, poly(3-hydroxyvalerate), poly(3-hydroxyhexanoate), poly(3- hydroxyoctanoate), etc. They all differ considerably in their physicochemical properties, specifically in crystallinity, melting and glass transition temperatures, hydrophobicity, plasticity, elastic modulus, etc. [2].
Naturally occurring PHAs hold promise as polymer platforms for controlled-release drugs. PHB and its copolymers can be loaded with a wide range of drug substances: model DS (2,7- dichlorofluorescein, FITC-dextran, methyl red, 7-hydroxyethyltheophylline, calcein, Sudan Red 5B (Oil Red O), rhodamine B isothiocyanate, thymoquinone), antibiotics and antibacterial DS (rifampicin, tetracycline, cefoperazone, gentamycin, sulperazone, duocid, sulbactam, cefoperazone, fusidic acid, nitrofural, norfloxacin, azithromycin, ceftiofur), anticancer drugs (5-fluorouracil, 2',3'-diacyl-5-fluoro-2'-deoxyuridine, paclitaxel, docetaxel, rubidomycin, tacrolimus, chlorambucil, etoposide, doxorubicin), anti-inflammatory drugs (indocid, flurbiprofen, ibuprofen, triamcinolone acetate), pain relievers (morphine, hydroxymorphine, codeine, bupivacaine, tramadol), antiplatelet agents (dipyridamole, nitric oxide donors, nimodipine, felodipine), antihypertensive drugs (manidipine hydrochloride), immunosuppressants (fingolimod), birth control (levonorgestrel), model and therapeutic proteins and peptides (bovine serum albumin, hepatocyte growth factor, mycobacterial proteins for vaccine production, bone morphogenetic protein, nafarelin, and insulin) [1].
PHA-based micro- and nanoparticles for DS loading can be obtained using a variety of different methods: precipitation, one-, two-, or multiple-step emulsification, spray-drying, layer-by-layer self-assembly in a solution, dialysis, precipitation in the presence of supercritical carbon dioxide, and electrospraying. There have been successful attempts to use PHB homopolymers, copolymers and composites as components of medicinal products loaded with active pharmaceutical ingredients; the list of such products comprises experimental films, prototypes of medical devices, micro- and nanoparticles, and pharmaceutical formulations [1].
A number of such PHA-based medicinal products have been tested both in vitro and in vivo for their pharmacological activity, specifically their antibacterial and antitumor effects and cytotoxicity in mammalian cancer cells; their safety and therapeutic efficacy has been studied in laboratory rats and mice [1].
Micro- and nanostructure of PHA-based drug systems
The inner structure and surface morphology of biopolymer microparticles are determined by a number of factors, including the method of their synthesis and the physiochemical properties of the encapsulated DS and the polymer itself. Biopolymer microparticles will be homogenous and nonporous only if the encapsulated drug substance is well soluble in a solvent used to dissolve the polymer. fig. 1A shows homogenous PHB-based microspheres, which were synthesized during one of our experiments, loaded with paclitaxel. Unlike the spheres doped with doxorubicin (fig. 1B), paclitaxel-loaded microparticles have a smooth surface.
If a loaded DS is not well soluble in the solvent, it can organize into crystals inside the polymer matrix of a microparticle, as well as on its surface (fig. 1С) [3].
Poor solubility of a loaded DS in the solvent may stimulate formation of defective microparticles with lamellar structure, undesired porosity and irregular shape regardless of the synthesis technique applied (fig. 1D).
Doping PHA-derived microparticles with high molecular weight bioactive agents (therapeutic proteins) yields a composite consisting of polymers with very different physical and chemical properties. In fact, doping leads to the formation of complex supramolecular structures characterized by unintended porosity, roughness, and irregular shape.
Synthesis of NP from PHA is another important area of nanotechnology [4]. Nanoparticles are particles with a diameter of less than 1 μm. The small size of polymer NP determines their biological and physiochemical properties, such as the ability to overcome physical barriers, to be taken up by the mucosa of the bronchi, nasal pharynx, oral cavity, and stomach, and to permeate the cell membrane via endocytosis. The lack of interaction with immune cells, such as macrophages and lymphocytes, ensures increased bioavailability of DS encapsulated in polymer NP [5, 6]. As a rule, amphiphilic PHA copolymers are used for NP synthesis so as to extend the time of NP circulation in the blood stream; they also allow covalent conjugation of NP to the ligands capable of producing a target effect [7, 8].
The kinetics of DS release from microparticles derived from PHB or its copolymers is largely affected by a partially crystalline structure of the polymer. As a rule, PHB can organize into lamellar crystals of 0.3–2 μm and 5–10 μm in dimensions for the short and long axis, respectively (fig. 2A).
The thickness of PHB crystals varies from 4 to 10 nm depending on the molecular weight, the solvent used, and crystallization temperature [9]. fig. 2B shows a stack of parallel lamellae of 11–40 nm in width. According to some reports, the lamellar thickness of similar films measured by small-angle X-ray scattering is 6.4 nm [10]. Such difference in the lamellar thickness can be explained by the fact that lamellar planes can be spatially positioned at different angles to the film surface (fig. 2B).
The diversity of crystalline structures that PHB can assemble into has been demonstrated using PHB-based ultrathin films and encompasses crystals resembling seaweeds in morphology and two-dimensional spherulites (fig. 2C). On average, these structures rise 4.5 nm above the substrate and take different growth directions from the crystal core. Unlike “seaweeds”, 2D spherulites are uniform in their growth direction.
The observed partially crystalline PHB structures are analogous to the structures occurring in ultrathin films of other polymers, such as polyethylene oxide, polylactide, and polystyrene [11].
Controlled release of DS from a polymer matrix derived from PHB or its copolymers indirectly depends on the natural properties of this biopolymer. In nature, PHB is synthesized in PHA granules or carbonosomes of a bacterial cell where it exists in a mobile amorphous state [12]. It is hypothesized that water that constitutes a small part (5–10%) of native PHB granules plays the role of a plasticizer that allows the biopolymer to maintain its amorphous state [12, 13]. Once water is removed from native granules, the polymer chains organize into a lamellar-crystalline structure. An assumption was made that water molecules promote formation of cross-linking hydrogen bonds between the carbonyl groups of polyether chains. Such molecular structure can explain the mobile amorphous state that PHB has in vivo [14]. The nanostructure of PHB samples precipitated from a solution promotes diffusion of water deep into the polymer matrix once the polymer is again submerged into an aqueous medium; this stimulates plasticization (to a very small degree, though) similar to that occurring in the PHA granules of bacteria. At the same time, even if PHB is in its mobile amorphous state, its specific structure protects it against hydrolytic destruction by water that plasticizes it in the granules [15–18]. Water that plasticizes PHB also promotes diffusion of DS encapsulated in the medicinal products based on PHB-derived micro- or nanoparticles: PHB polymer chains are actively synthesized and cleaved in carbonosomes; this process is accompanied by the intense diffusion of PHB monomer precursors, ATP, NADP/NADPH, and other low molecular weight compounds in the matrix of the amorphous polymer. This means that the mechanism of synthesis and degradation of PHB in the bacterial cell involves diffusion of low molecular weight compounds in the polymer matrix and that the interactions between the biopolymer and proteins occurring in the polymer granule (carbonosome) of the bacterial cell render immobilization of proteins and other biomolecules in polymer micro- and nanoparticles possible.
CONCLUSIONS
The functional properties of PHA-based drug systems may depend on the biomimetic nano- and microstructure of PHA that, in turn, is dictated by the natural function the polymer exerts in bacterial cells. New biomimetic PHA-based drugs will help to overcome the drawbacks of traditional drug formulations that often exhibit unsatisfactory physiochemical performance.