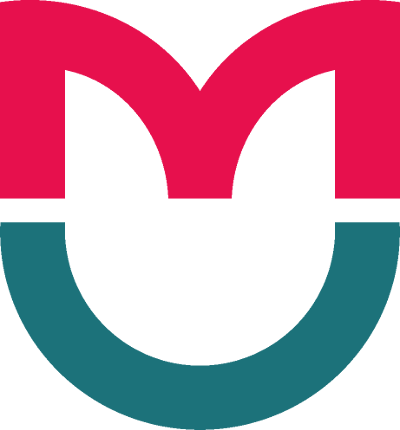
METHOD
Synthesis of 13С- and 14С-labeled linoleic acids for use in diagnostic breath tests for hepatobiliary system disorders
1 Russian State University of Physical Education, Sport, Youth and Tourism, Moscow, Russia
2 Skryabin Moscow State Academy of Veterinary Medicine and Biotechnology, Moscow
3 Vavilov Institute of General Genetics, Russian Academy of Sciences, Moscow, Russia
4 I. M. Sechenov First Moscow State Medical University, Moscow, Russia
5 Blokhin National Medical Research Center of Oncology, Moscow, Russia
6 Plekhanov Russian University of Economics, Moscow, Russia
Correspondence should be addressed: Yaroslav Y. Tynio
Sirenevyi bulvar, 4, Moscow, 105122; ur.liam@oinytyy
Author contribution: Tynio YY conceived and supervised the study; Morozova GV synthesized the final product by carboxylation of the Grignard reagent with 13С and 14С dioxides, did preparative calculations; Biryukova YuK conducted NMR-analysis of the final product; Sivokhin DA analyzed the literature and wrote the manuscript; Pozdniakova NV analyzed the literature; Zylkova MV measured the radioactivity of carbon atoms in the reagents, intermediate and final products; Bogdanova ES analyzed the NMR spectra of the final products; Smirnova MS determined the melting point of the final products; Shevelev AB provided reagents and instrumentation and revised the manuscript.
The prevalence of chronic liver diseases that progress to cirrhosis, including hepatitis B and C, alcoholic liver disease, toxic hepatitis, primary sclerosing cholangitis, etc., is on the rise [1].
The gold standard for evaluating the liver is a liver biopsy. However, being an invasive procedure, it is associated with the risk of complications and, therefore, cannot be used as a routine test [2]. Adoption of highly reliable, simple and safe noninvasive diagnostic tests into clinical practice [3] will allow clinicians to monitor the efficacy of treatment and estimate the functional reserve of the liver [2, 4]. Such tests have a strong advantage over elastography, as well as APRI [5] and FORNS [6] scores calculated from a patient’s laboratory data.
Breath tests rely on the body’s ability to metabolize 13С- and 14С-substrates into 13СO2 [7] or 14СО2 [8] that are subsequently transported in the blood to all organs and tissues and excreted through the lungs; 13СO2 or 14СО2 concentrations in exhaled breath can be reliably detected by mass spectrometry, nondispersive infrared spectroscopy (NDIRS) and cavity ring-down spectroscopy (CRDS), a spectroscopic technique for measuring absorption of laser light by a gaseous sample introduced into an optical cavity consisting of two high-reflectivity mirrors, where the laser pulse is reflected between them back and forth; in CRDS, absorption is calculated from the time of light decay (the ring-down time) [3, 9]. Thus, a diagnosis can be stablished by analyzing the pharmacokinetics of a radiolabeled compound, such as information about its metabolic pathways and rates and 14СО2 concentrations in exhaled breath [10].
Currently existing clinical breath tests used to evaluate liver function measure the metabolic activity of 13С-methacetin [11–13], 13С-galactose, cytochrome Р450 (the 13С-aminophenazone test) [14], 13C-phenylalanine [15, 16], 13C-caffeine [17, 18], and 13С3-trioctanoin (a triglyceride of 13С-labeled octanoic acid esters), which facilitates the diagnosis of exocrine pancreatic insufficiency [7, 19].
Breath tests that harness radiolabeled fatty acids, like linoleic acid, hold great promise for nuclear medicine. Linoleic acid plays an essential role in the energy metabolism of higher organisms and is a building block for a few lipid classes, including neutral fats, phosphoglycerides and cholesterol esters [20].
Linoleic acid is a long-chain water-insoluble compound. Bile secreted by the gall bladder catalyzes hydrolysis of linoleic acid in the small intestine; the reaction results in the formation of mixed micelles. The lack of bile salts in the bile caused by a hepatobiliary disorder slows absorption of a labeled fatty acid, which can be inferred from the isotopic composition of exhaled carbon dioxide [20].
The literature reports an 11-step method for stereospecific synthesis of [1-14C] isomers of monounsaturated fatty acids using olefin inversion [21]. Advantageously, this method allows obtaining monounsaturated fatty acids with a C=C double bond at different positions. However, it is very labor-intensive, involves preparative separation of stereoisomers upon epoxidation and requires an expensive and toxic source of 14С, such as cyanide. These factors impede adoption of the method in large-scale manufacturing.
So far, there are no published reports describing chemical synthesis of 13С- and 14С-labeled derivatives of most common dietary unsaturated fatty acids (linoleic and linolenic) from the most common source (СО2) Synthesis by biological methods involving protists and fungi, like Thraustochytrium and Mortierella alpina, has a low radiochemical yield under 60% [22, 23].
The residual isotope is disposed of as waste. Given that 14С is a long-lived radionuclide, this method of synthesis is a hazard to the environment. Another drawback of the method is distribution of labeled atoms along the entire carbon chain of the acyl group, which limits application of synthesized 13С- and 14С-labeled fatty acids in breath tests. The highest sensitivity, repeatability and safety of a liver function breath test can be achieved by using fatty acids in which 100% of labeled atoms are at position 1 (the carboxyl group). Biogenic synthesis often results in a mix of fatty acids differing in their composition. For instance, Thraustochytrium-based synthesis produces 10 different fatty acids with a yield range of 0.72 to 21.82%. Besides, 1.9% of the isotope is included in the structure of unidentified fatty acids [23].
This study aimed to develop a method of synthesis of linoleic acids labeled with 13С- and 14С-atoms at position 1 of the acyl group using CO2 as an isotope source for use in diagnostic tests for hepatobiliary disorders.
METHODS
Equipment
Purity of intermediate and final reaction products was controlled by means of thin-layer chromatography using precoated silica gel Kieselgel 60 F254 TLC plates (Merck; Germany) and Sorbfil plates PTLC-AF-V-UF. The ethyl acetate : n-hexane (1 : 1) eluent mixture was used as a mobile phase. The separated analytes were visualized by exposing the plates to iodine vapors. The structure of the final product was confirmed using nuclear magnetic resonance spectrometers AM-300, 300 MHz (Bruker; Germany) and DRX-500, 500 MHz (Bruker; Germany). Deuterated chloroform was used as a solvent for NMR analysis; NMR spectra were recorded at 300.1 mHz.
Specific activity of tracer carbon was measured by means of a DPM 7001 liquid scintillation counter (RadEk Scientific and Technical Center; Russia) equipped with 2 photomultiplier tubes. Microcalorimetric analysis of mixtures was carried out using a Setaram С80 Calvet calorimeter (SETARAM Instrumentation; France). Potentiometric pH measurements of aqueous solutions were taken with a Sartorius PB-11 basic meter (Sartorius; Germany).
Materials
The following reagents were used in the experiment:
– 1-bromo-8,11-heptadecadien CH3(CH2)3-(CH2CH=CH)2 (CH2)7Br (AppliChem; USA); molar mass 315.332 g/mol, melting point 3.4 °C, boiling point 112 °C [24];
– the source of a stable carbon isotope: anhydrous barium carbonate-13С (JSC Isotope; Russia); isotopic purity 99.32%; molecular weight 198.3359 g/mol; melting point 1,558 °C;
– the source of a radioactive carbon isotope: anhydrous barium carbonate-14С (Mayak Production Association, Rosatom; Russia); isotopic purity 97.8%; molar mass 199.3359 g/mol; melting point 1,566 °C; specific activity 66.92 mCi/g;
– among other reagents were high-purity dry argon and nitrogen (M-Gas; Russia); ethyl acetate, GOST 22300-76 rev.1-3 (Chimmed; Russia); n-hexane, specifications 2631-158- 44493179-13 (Lenreactiv; Russia); magnesium turnings, GOST 804-93 (Interchim; Russia); crystalline iodine, reagent grade (Lenreactiv; Russia); diethyl ether, specifications 2600-001- 45682126-13 (Chimmed; Russia); sulfuric acid, pure grade, GOST 4204-77 (Chimmed; Russia); hydrochloric acid, pure grade, GOST 3118-77 (Chimmed; Russia); sodium hydroxide 98% (Fluka; Switzerland; catalog number 71695); acetonitrile, specifications 6-09-3534-87 (Chimservice; Russia).
Absolute ether was prepared as described below. Briefly, diethyl ether was washed in the saturated solution of calcium chloride (50 ml of the solution per 1 L of ether) and dried for 48 h over calcium chloride precalcined at +120 °С for 24 h (130 g of calcinated calcium chloride per 1 L of ether). The reagent was filtered through a fluted paper filter into a dry flask; then, sodium metal (1 g per 1 L of the reagent) was added to the flask. The flask was closed with a holed stopper holding a calcium chloride drying tube. Absolute ether was used to carboxylate the Grignard reagent once hydrogen was no longer released after sodium addition.
RESULTS
Linoleic acid labeled with 13С and 14С at position 1 was synthesized in two steps: 1) preparation of the Grignard reagent; 2) carboxylation of the Grignard reagent with 13С and 14С dioxides.
Preparation of the Grignard reagent
The apparatus for synthesizing the Grignard reagent was set up as shown in fig. 1 (adopted from [25]).
A 250 ml three-necked flask was clamped on a stand; a reflux condenser with a calcium chloride drying tube and a pressure-equalizing dropping funnel were fitted into the side necks of the flask. An electric stirrer was introduced into the middle neck through an oil seal. Magnesium turnings (3.0 g) and an iodine crystal were put inside the flask. High purity grade argon was blown through the apparatus for 20 min. Then, 160 ml of absolute ether was added into the flask through the dropping funnel, the stirrer was switched on, and 3.78 g (12 mmol) of the 1-bromo-8,11-heptadecadien solution in diethyl ether (80 ml) was added under weak argon flow. To initiate the reaction, the flask was warmed over a water bath until the ether started to boil. Once the reaction started, the water bath was switched off and stirring continued until the magnesium was completely consumed.
Carboxylation of the Grignard reagent with 13С and 14С dioxides
Isotopically labeled acids were obtained through carboxylation of the Grignard reagent using a high vacuum manifold with ports for connecting a reaction flask, a СО2 source, a mercury column manometer, and tubes for nitrogen inlet/outlet to the line. A cone-shaped three-necked reaction flask resistant to freezing was equipped with a magnetic stirrer that allowed carrying out reactions in vacuum at low temperatures (fig. 2; adopted from [25]).
The source of 13С and 14С dioxides was represented by 5.4 mmol of isotopically labeled barium carbonate (a weighted amount of 1.071 g for the 13С isotope and a weighted amount of 1.076 g for the 14С isotope) placed in a round-bottom flask. The flask was connected to a pressure-equalizing dropping funnel filled with concentrated sulfuric acid. This part of the apparatus was connected to the vacuum manifold via a desiccant-containing tube.
First, the apparatus was evacuated to 0.1 mmHg using an oil pump and filled with dry nitrogen. Then, the Grignard reagent solution prepared from 6 mmol of 1-bromo-8,11- heptadecadien (half of the total synthesized amount) was taken up into a prewashed dispenser pipette filled with nitrogen and quickly injected into the flask. The free side neck of the flask was closed with a stopper. The flask was cooled in liquid nitrogen, and the apparatus was evacuated to 0.1 mmHg. Then, the solution in the reaction vessel was thawed to –77 °С in a mixture of dry ice and acetone, frozen in liquid nitrogen, and the apparatus was again evacuated to remove nitrogen.
Carboxylation of the Grignard reagent was performed at –20 °С under continuous stirring. To initiate liberation of labeled СО2, concentrated sulfuric acid was slowly added to barium carbonate through the dropping funnel, making sure that the pressure did not exceed 500 mmHg. To finish off liberation of labeled СO2, the reaction flask was carefully heated until barium carbonate was completely dissolved. After the Grignard reagent was depleted, manometer readings indicated that СO2 pressure in the apparatus was no longer decreasing. For both 13СO2 and 14СO2 the reaction was completed in 15 minutes.
The reaction flask with the Grignard reagent was cooled in liquid nitrogen to collect the remaining labeled СO2; the stopcock connecting the apparatus to the source of labeled СO2, was closed and the reaction mass was stirred for 15 min at –20 °С until labeled СO2 was fully absorbed. Then, the apparatus was filled with nitrogen and connected to the air inlet tube. The obtained complex was decomposed by diluted hydrochloric acid. The acidified mixture was extracted in ether. The resulting ether extract was treated with 100 mM NaOH, and the obtained alkaline solution was acidified to achieve рН = 7.0. The released acid was filtered. The precipitate was collected, washed in water and recrystallized from acetonitrile at –20 °С (for linoleic acid, t freez was assumed to be –11 °С).
The total mass of the synthesized 13С-linoleic acid equaled 1,459 mg (5.184 mmol). The final chemical yield of 1-bromo- 8,11-heptadecadien was 86.4%; the 13СO2 yield was 96.0%. For the final product, the empirically measured freezing point was –11.0 °С.
The total mass of the synthesized 14С-linoleic acid was 1,480 mg (5.243 mmol). The yield of 1-bromo-8,11- heptadecadien was 87.39%; the 13СO2 yield was 97.1%. The specific activity of 14С-linoleic acid was measured using the scintillation counter and equaled 45.36 ± 0.02 mCi/g. Thus, the total radiation and chemical yield was 96.0%. The final reaction product had a freezing point at –10.7 °С.
The synthesized 13С- and 14С-linoleic acids were analyzed by means of thin layer chromatography using Kieselgel 60 F254 plates; the ethyl acetate : n-hexane (1 : 1) eluent mixture was used as a mobile phase (see Methods); the obtained linoleic acids contained admixtures. 13С-linoleic acid made up 98.2% of the dry product weight after elution.
The 1H NMR spectrum of 14С-linoleic acid was compared to the reference spectrum of 1S/C18H32O2/c1-2-3-4-5-6-7-8-9- 10-11-12-13-14-15-16-17-18(19)20/h6-7, 9-10H, 2-5, 8, 11- 17H2, 1H3, (H, 19, 20)/b7-6-, 10-9 described in the literature [26]; the synthesized compound matched the structure of the linoleic acid (fig. 3).
DISCUSSION
The proposed method of synthesis of 13С- and 14С-labeled linoleic acids has a few strengths, including shorter reaction time, reduced loss of labeled СО2 and increase in the total chemical and radiation yield. Importantly, the isotopically labeled atoms are not distributed along the entire carbon chain of acyl but instead occur only at position 1.
Shorter reaction time due to the optimization of reagents ratios and measurements of the СО2 pressure in the system taken to check the completion of Grignard reagent carboxylation ensured operational simplicity and cost-effectiveness of the method. The method significantly increases the radiation and chemical yield of the product in comparison with other known techniques [21–23]. The labeled isotope is almost entirely included in the final reaction product, meaning that the amount of radioactive waste is near zero. This makes the proposed method appealing in terms of cost reduction, given that disposal of long-lived radioactive waste is difficult and expensive.
The amount and purity of the synthesized 13С- and 14C-linoleic acids make them suitable for use in preclinical trials of acute, subchronic, chronic and other types of toxicity [27]. Once the safety of the compounds has been confirmed, they can be recommended for clinical trials of hepatobiliary function breath tests.
CONCLUSIONS
This paper describes a method of synthesis of 13С- and 14C-linoleic acids with carbon isotopes occurring at position 1. The method is advantageously simple and consists of 2 steps instead of 11 reported in other works. For 14С, the radiochemical yield of the method is very close to quantitative (96%), which almost rules out the issues associated with radioactive waste disposal. The method requires no sophisticated analytical and preparative instrumentation, which may facilitate its adoption to batch production.