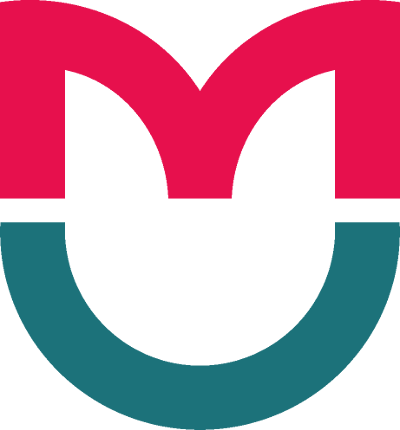
ORIGINAL RESEARCH
Changes in EEG patterns in the α-frequency band following BCI-based therapy in children with cerebral palsy
V.I. Vernadsky Crimean Federal University, Simferopol, Russia
Correspondence should be addressed: Vladimir B. Pavlenko
Pr. Vernadskogo, 4, Simferopol, 295007; moc.liamg@55vapv
Funding: the study was part of the state-funded project RFMEFI60519X0186 on the Development of a BCI-based hand exoskeleton with biological feedback for the rehabilitation of children with cerebral palsy. The study was supported by the Ministry of Science and Higher Education of the Russian Federation.
Author contribution: Larina NV, Korsunskaya LL, Vlasenko SV — data acquisition, manuscript preparation; Nacharova MA, Pavlenko VB — data analysis, manuscript preparation.
Compliance with ethical standards: the study was approved by the Ethics Committee of Vernadsky Crimean Federal University (Protocol № 53 dated December 06, 2018). Informed consent was obtained from the patients or their legal representatives.
In most economically developed countries, cerebral palsy (CP) is the leading cause of motor disability in children. CP affects 1 in 500 newborns; globally, there are 17 million people living with CP [1]. CP is an umbrella term for the group of chronic nonprogressive motor impairments (spastic paresis, hyperkinetic disorder, cerebellar ataxia, pseudobulbar palsy) that stem from brain damage or developmental delay before, during or after birth. In patients with CP, motor dysfunction usually co-exists with mental retardation, epilepsy, perceptual, learning or communication difficulties [2]. Because clinical manifestations of CP are very diverse, medications and physiotherapy may not always be effective. One of the most promising methods for managing motor dysfunction relies on the use of noninvasive brain-computer interfaces (BCI) and robotic devices with biological feedback [3]. They are capable of recognizing a patient’s motor intention from changes in his or her EEG during kinesthetic imagery followed by relevant activation of the robotic device. It is hypothesized [4] that robotic orthoses might significantly improve treatment outcomes: the patient receives feedback about how well the mental task is being performed whereas enhanced sensory input (tactile, proprioceptive or visual) stimulates brain structures that are fully or partially excluded from processing sensory information because to brain damage. This technology has been proved effective for post-stroke patients [5, 6].
Although it is reported that BCI might be a potent rehabilitation tool for children with CP [7, 8], few studies have addressed this possibility so far. It was reported that individuals with CP could benefit from BCI that rely on the analysis of the sensorimotor EEG rhythm [9]. Kinesthetic imagery affected the power of the μ and β EEG rhythms analyzed by a classifier. In another study, a BCI was used to, firstly, explore how the power ratio of the β- to θ-rhythms changed in patients who were mentally rehearsing a wrist extension movement and, secondly, trigger electrical stimulation of wrist extensors [10]. After a series of such BCI-based session, children with CP demonstrated improvement in their hand movement parameters.
In another study, a BCI-controlled robotic hand was tested in children with CP undergoing rehabilitation at a medical health resort [11]. By the end of the neurorehabilitation course, the majority of the participants had achieved a reduction in wrist muscle spasticity, improved muscle strength, expanded the range of wrist movements and the repertoire of daily routines they were able to perform. By contrast, the only positive trend observed in the patients undergoing conventional treatment without BCI-based training consisted in performing more daily tasks, but those improvements were statistically insignificant. At that point, EEG records were not analyzed.
Reactivity of the α- and μ-rhythms is an attractive field for research, especially in the context of analysis of CNS activity in patients with motor impairment undergoing neurorehabilitation. The amplitude of the α- and μ-rhythms can be attenuated or enhanced by physical or mental activity. These phenomena are called event-related desynchronization and synchronization, respectively. A study demonstrated that in healthy volunteers, certain patterns of α-rhythm reactivity indicate involvement of various neocortical regions in processing sensory input from different modalities [12], whereas patterns of μ-rhythm reactivity are indicative of activation in the somatosensory and motor cortex during motor execution and imagery [13, 14]. The μ-rhythm occurs in the alpha frequency range, which has a contribution from the occipital α-rhythm during motor imagery; the occipital α-rhythm is partially retained when the eyes are open and is characterized by high reactivity during cognitive activities [15]. So, the terms “the α-rhythm” or “alpha rhythmic activity” used below will refer to the activity resulting from the α- and μ-rhythms.
It was reported that these rhythms undergo synchronization or desynchronization in poststroke patients during motor imagery [16]. There were changes in the patterns of amplitude attenuation and enhancement following the rehabilitation course with a BCI-controlled robotic hand. The researchers proposed that such reactivity might be indicative of the reorganization of neural circuits during neurorehabilitation. Today, the patterns of α- and μ- rhythm reactivity in children with CP remain understudied.
The aim of this paper was to describe the reactivity patterns of the EEG α-rhythm during a series of neurorehabilitation sessions with a non-invasive BCI-controlled robotic hand allowing biological feedback in CP-stricken children with a paretic upper limb.
METHODS
Demographics
The study was conducted at the Psychiatry and Neurology Unit of E.P. Glinka Yevpatoria Clinical Sanatorium for Children. The study recruited patients aged 10 to 18 years undergoing neurorehabilitation with a noninvasive BCI-controlled robotic hand (Exohand-2) developed by Android Technics in collaboration with Pirogov Russian National Research Medical University and Institute of Higher Nervous Activity and Neurophysiology (Russia). The following inclusion criteria were applied: CP (according to ICD-10), hemiparesis, GMFCS (Gross Motor Function Classification System for Cerebral Palsy) level III or below. Patients at GMFCS level > III, with plegic upper limbs, aphasia, pharmacologically refractory epilepsy, or visual impairments preventing them from reading instructions on the computer screen, and those with moderate, severe or profound mental retardation (F71–73 according to ICD-10) were excluded from the study.
We shortlisted 32 patients, who were then referred to the medical health resort for rehabilitation. The patients were stratified into 2 groups: those with left-side hemiparesis (16 patients, including 10 boys and 6 girls) and those with right-side hemiparesis (16 children, including 9 boys and 7 girls). Of them, 21 patients were at GMFCS level II and 11 at GMFCS level III. There were 11 children aged 10–12 years, 12 children aged 13–15 years, and 9 patients aged 16–18 years. The mean age was 13.6 ± 2.5 years. The groups did not differ significantly in terms of age.
Rehabilitation, EEG recording and analysis
The device relies on the analysis of EEG patterns during motor imagery by a Bayesian classifier that analyzes covariance matrices of EEG signals [17].
During the sessions, the patients remained seated in armchairs at a 1.5 m distance from the computer screen where visual instructions were displayed. Their hands were inside the “glove” of the robotic hand. A round white fixation mark was displayed in the center of the screen, with 3 arrows around it that changed their color to present a task. The tasks included resting (the top arrow was activated for 10 s) and kinesthetic imagery of right- or left-hand finger extension (the right or left arrows were activated for 10 s). If the task was performed successfully, the fixation mark went green and the robotic hand extended passively. Thus, the patient received both visual and kinesthetic feedback.
Each patient received 10 rehabilitation sessions on alternate days, consisting of 3 8-minute long trials with at least 5-minute breaks. For each hand, the movement was mentally rehearsed 24 times during one session. Apart from BCI sessions, the 21-day-long rehabilitation course included conventional therapy [11], specifically physical exercise, massage of the paretic muscles, peloid therapy, kinesiotherapy in thermal water, electrical stimulation of the antagonists of the paretic muscles.
EEG was recorded in a monopolar fashion using a Neurovisor BMM electroencephalograph (Medical Computer Systems; Russia). Electrodes were placed according to the international 10-10 system over the frontal (Fpz, Fp1, Fp2, Fz, F1, F2, F3, F4), central (Cz, C1, C2, C3, C4), parietal (Pz, P1, P2, P3, P4), and occipital (Oz, O1, O2) lobes. An ear electrode was used as a reference. The cutoff values for high and low frequencies were 5 and 30 Hz, respectively; the sampling rate was 500 Hz.
EEG fragments with an amplitude over 250 μV or containing a lot of artifacts pertaining to the electrical activity of forehead muscles were excluded from the analysis. EEG fragments recorded during mental rehearsal of the right/left hand movement and the resting stage (eyes open; baseline) that were 10 s long and free of artifacts were selected for further analysis. There were 10 to 15 such fragments for each task per session. These EEG fragments were processed with Fast Fourier transform (epoch time 2.5 s, epoch overlap 50%). The data were tapered using the Blackman window. For each 10-s long EEG fragment, we calculated the mean alpha amplitude for the frequency band of 8 to 13 Hz and the reactivity indices (RI) using the formula:
RI = 100% × [(В – A) / A],
where B is the mean α-rhythm amplitude during the 10-s long motor imagery task and A is the mean α-rhythm amplitude in the resting phase (baseline condition) preceding the motor imagery task. For the right and left hands, mean RI values were calculated separately.
Positive RI values indicated an increase in the α-rhythm amplitude during motor imagery relative to its baseline value (synchronization), whereas negative RI values meant a reduction in the α-rhythm amplitude (desynchronization). RI values from the 1st and 10th sessions were averaged and used in the subsequent analysis.
Statistical analysis
Statistical analysis was carried out in STATISTICA 10.0 (StatSoft Inc.; USA). The Shapiro-Wilk test revealed that the distribution of alpha RI values for all EEG leads was normal. Therefore, repeated measures ANOVA was applied to the obtained data. Using a 2 × 2 × 21 design, we evaluated the effects of one intersubject factor GROUP (assignment to the group of subjects with right/left hemiparesis) and two intrasubject factors: HAND (mental rehearsal of the right/left hand movement) and LOCUS (position of one of 21 electrodes). Changes in α-rhythm reactivity following rehabilitation were analyzed using ANOVA with 3 intrasubject factors: SESSION (1st and 10th), HAND and LOCUS. To assess RI changes at each of electrode position, a priori contrasts were applied (the F-distribution test). Differences were considered significant at р < 0.05, but due to the small sample size, we also kept track of trends (р < 0.10).
RESULTS
Previously, we demonstrated that rehabilitation with a noninvasive BCL controlled robotic hand significantly improved motor function of the upper limbs in children with CP [11]. Patients who received only traditional rehabilitation therapy were unable to achieve statistically significant improvements. At that point, no analysis of EEG records was conducted.
Alpha-rhythm reactivity at the beginning of the rehabilitation course
Bidirectional modulations of the α-rhythm amplitude were noted during motor imagery in the first session of BCI-based therapy (fig. 1, fig. 2, red columns). Event-related synchronization was observed at most electrode sites in both hemispheres. Patterns of α-rhythm modulations were different between children with right-side and left-side hemiparesis. The effect produced by the interaction of GROUP × HAND × LOCUS was close to statistically significant (F20, 600 = 1.45; р = 0.09). The a priori contrasts analysis revealed significant differences in α-rhythm reactivity at P2 (the right parietal electrode; F1, 30 = 5.10; p < 0.05) during mental practice of the left-hand movement (fig. 1) At P2, α-rhythm synchronization was very pronounced in children with left-side hemiparesis (fig. 1A), whereas children with right-side hemiparesis demonstrated only a slight change in the α-rhythm amplitude (fig. 1B).
The a priori contrasts analysis uncovered a tendency to more pronounced α-rhythm synchronization at P2 during right-hand movement imagery in children with right-side hemiparesis (F1, 30 = 2.81; p = 0.10), as compared to those with left-side hemiparesis (fig. 2A, B).
Alpha-rhythm reactivity at the end
of the rehabilitation course
The pattern of α-rhythm reactivity observed in our patients during the final session differed from that observed at the beginning of the rehabilitation course.
The impact of the SESSION × HAND × LOCUS combination (F20, 300 = 1.84; p < 0.05) on α-rhythm reactivity was significant in children with left-side hemiparesis. Synchronization of the α-rhythm during left-hand movement imagery observed at Fp1, Fp2, P2, and Oz at the beginning of the rehabilitation course now gave way to desynchronization (fig. 1А). Differences in RI values between the 1st and 10th sessions were statistically significant at P2 (F1, 15 = 10.02; р < 0.01) and Oz (F1, 15 = 7.68; р < 0.05) and close to statistically significant at Fp1 (F1, 15 = 3.96; р = 0.07). Significant differences in α-rhythm reactivity were detected at C1 (F1, 15 = 6.57; р < 0.05) in children with left-side hemiparesis during mental rehearsal of the right-hand movement. By the end of the rehabilitation course, synchronization had taken over desynchronization (fig. 2A).
RI values did not differ significantly between the 1st and 10th sessions in children with right-side hemiparesis imagining right- and left-hand movements (fig. 1B, fig. 2B). The impact of the SESSION × HAND × LOCUS combination on α-rhythm reactivity was insignificant (F20, 300 = 0.86; p = 0.64).
After the rehabilitation course was over, the differences in α-rhythm reactivity between children with right- and left-side hemiparesis observed during motor imagery became much less pronounced: the impact of the GROUP × HAND × LOCUS combination was now statistically insignificant (F20, 600 = 0.88; р = 0.61).
DISCUSSION
This study analyzes changes in EEG signals during BCI-based neurorehabilitation of patients with CP. The EEG amplitude modulations in the alpha frequency band measured in children with CP during motor imagery was surprisingly low. At most electrode sites, RI values did not exceed 3%. To some extent, this can be explained by the atypical modulation of EEG rhythms observed in patients with CP. Event-related μ-rhythm synchronization and desynchronization responses during motor activity were weak in patients with CP, as compared to healthy individuals. Those responses did not have distinct localization in patients with CP: EEG signal changes were observed in different neocortical regions, including the parietal and occipital lobes [:lit_18–20]. Besides, although our patients were asked to imagine movements continuously for 10 seconds, changes in α-rhythm amplitude were not always solid. In healthy adults performing motor imagery tasks μ-rhythm changes follow a multiphase pattern characterized by alternating synchronization and desynchronization [14]. The same alternating pattern of the μ- and α-rhythms was observed during motor imagery in adult poststroke patients undergoing rehabilitation with robotic devices [16]. It is possible that in our study event-related synchronization and desynchronization responses appeared smoothed out because we analyzed the EEG amplitude for the entire period of motor imagery.
The pattern of α-rhythm reactivity during motor imagery was bilateral. We saw the amplitude grow and decline not only in the contralateral but also in the ipsilateral hemisphere (relative to the hand the subject was focused on). Such atypical bilateral patterns of motor activity in children with CP were previously reported by other authors [21]. Using functional MRI, the researchers studied activation of CNS structures during motor imagery (grasping a ball) and recorded hand muscle responses to transcranial magnetic stimulation. The authors concluded that more than half of children with CP control the paretic hand with either ipsilateral or both hemispheres. Such unusual brain organization is probably compensatory; it develops after brain damage at early age and relies on the plasticity of the nervous system.
Our study was carried out in two groups of patients: with right- and left-side hemiparesis. This allowed us to compare EEG responses to motor imagery in the relatively intact and profoundly damaged hemispheres. At the beginning of the neurorehabilitation course, the patterns of α-rhythm attenuation or enhancement during motor imagery differed between the groups. RI values were significantly different in the parietal lobe of the right hemisphere (Р2). After the sessions, differences in the degree of α-rhythm reactivity during motor imagery between children with left- and right-side hemiparesis became much less pronounced, probably indicating positive changes in CNS activity.
These changes are primarily associated with significant EEG reactivity in the alpha frequency band observed in patients with left-side hemiparesis at the end of the rehabilitation course. During the first BCI session, pronounced desynchronization (RI > 0.25%) was observed only at one electrode site (Сz) in this group of children, but during the final session, desynchronization was present in 6 regions of both hemispheres (fig. 1А). Although changes in EEG reactivity patterns observed at the end of the course in children with right-side hemiparesis were not statistically significant, there still was an increase in α-rhythm attenuation. During the first session, there were no signs of desynchronization at any of the electrode sites when the children were mentally rehearsing the movement of the paretic hand. However, in the final session, desynchronization was recorded at 6 electrode locations, mostly in the ipsilateral hemisphere (fig. 2B). An increase in event-related α-rhythm desynchronization suggests increased neocortical activation induced by neurorehabilitation [12–14].
An increase in desynchronization at Р2 (fig. 1A, B) is most noteworthy. The EEG signal at this location reflects the activity of the precuneal neural circuits [22]. The precuneus is profoundly involved in performing the broad spectrum of highly integrated tasks, including visuospatial motor imagery and first person perspective taking [23]. Increased activity of this brain region following neurorehabilitation may play a key role in the optimization of planning or executing complex hand movements and improved performance of daily activities.
Increased desynchronization in some neocortical regions was accompanied by event-related α-rhythm enhancement in other cortical areas. Thus, there was a significant increase in the α-rhythm amplitude in the primary motor (С2, С4) and premotor (F2, F4) cortices in children with left-side hemiparesis as they were imagining the movement of the paretic hand. These changes occurring in the alpha frequency band are evident of active inhibition aimed at preventing the overinvolvement of the damaged contralateral hemisphere in motor activity control.
Because children with CP had significantly improved their motor function by the end of the rehabilitation course [11], the observed changes in EEG patterns in the alpha frequency band can be regarded as mirroring positive reorganization of neural circuits. This study is preliminary in nature. We cannot rule out the impact of factors associated with traditional rehabilitation modalities on the pattern of EEG reactivity. We are planning to conduct a randomized cross-sectional study to evaluate the impact of those factors and to analyze the link between EEG changes and motor improvements in children with CP. We think that this and future studies will help to elaborate new approaches to improving the efficacy of neurorehabilitation.
CONCLUSIONS
Neurorehabilitation of children with CP involving the use of noninvasive BCL controlled robotic hand significantly improves motor function of the upper limbs and changes the pattern of α-rhythm reactivity during motor imagery. At the beginning of the rehabilitation course, the patterns of α-rhythm reactivity differed significantly between children with left- and right-side hemiparesis. By the end of the course, the differences became much less pronounced, suggesting positive changes in CNS activity. These improvements result from a statistically significant change in EEG reactivity patterns in patients with left-side hemiparesis. On the whole, children with left- and right-side hemiparesis demonstrated an increase in the proportion of α-rhythm desynchronization responses, indicating increased neocortical activity following neurorehabilitation. The observed changes in the EEG patterns in the alpha frequency band reflect increased plasticity of the neural circuits responsible for planning and executing complex hand movements.