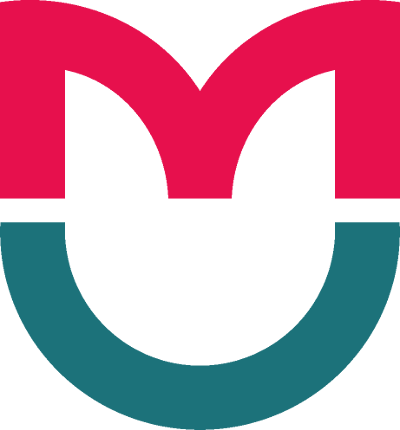
ORIGINAL RESEARCH
Complexes of fluconazole with alanine, lysine and threonine: mass spectrometry and theoretical modeling
Kulakov National Medical Research Center for Obstetrics, Gynecology and Perinatology, Moscow, Russia
Correspondence should be addressed: Vitaly V. Chagovets
Oparina, 4, Moscow, 117198; moc.liamg@stevogahcvv
Funding: the study was carried out as a part of the convention № 05.604.21.0241, Development of a Technology for Personalized Treatment of Mothers and Newborns With Infectious and Inflammatory Diseases Caused by Multi-resistant Strains of Microorganisms, Based on Genotyping of Pathogens and Therapeutic Drug Monitoring of Antimicrobial Drugs (item 1,2, queue 1), of the Ministry of Science and Higher Education of the Russian Federation. Programme for Research and Development in Priority Areas of Development of the Russian Scientific and Technological Complex for 2014-2020. Project ID: RFMEFI60419X0241.
Author contribution: Chagovets VV, Frankevich VE, Starodubtseva NL — study concept and design, data processing; Chagovets VV — statistical analysis; Chagovets VV, Starodubtseva NL — manuscript writing; Frankevich VE — editing.
Cytochrome P450 sterol 14α-demethylase (CYP51), the product of ERG11 gene, is a target site for triazoles. The CYP51 enzyme converts lanosterol into ergosterol being a part of the fungal cell membrane main component biosynthesis pathway [1]. Ergosterol biosynthesis inhibition slows down the mycelia cells growth due to toxic steroids (such as methylated sterols) accumulation [2–4], violating the membrane integrity, fluidity and permeability [5]. Fluconazole (Fluc) acts as a competitive inhibitor of CYP51. Currently, it is effective for treatment of many fungal infections.
The development of new classes of compounds is necessary for the treatment of mycoses and antifungal drugs resistance. Antifungal drugs are relatively difficult to develop compared to antibacterial drugs due to eukaryotic type of cells. Besides, the triazole derived antifungal agents (such as fluconazole) eventually become insufficient for treatment of many infections. The need to develop new medicinal products is an incentive to the triazole drugs inhibition molecular mechanisms study enabling to understand the molecular basis for the fluconazole affinity and specificity.
Previously, the molecular modeling was used to search for pyrazole analogues. The search was based on the CYP51-Ca protein structure [6, 7]. Furthermore, the molecular modeling made it possible to investigate the features and patterns of various inhibitors interaction with CYP51 [8], as well as the role of mutations in the stability and affinity of enzyme binding [9, 10]. Molecular dynamics also made it possible to study the factors of the fluconazole greater affinity for fungal enzymes rather than mycobacterial and human orthologs [11]. A review of the modeling strategies used to design the P450 binding ligands was recently published [12].
Database analysis revealed a number of conserved amino acids (AA) [13, 14]. Of those, three amino acids playing a vital part in protein–inhibitor biding were selected using the molecular dynamics modeling: threonine (Thr77), alanine (Ala258) and lysine (Lys454) [8].
Although the molecular mechanics studies may help to predict amino acids involved in the protein–inhibitor complex assembly, it is important to have a number of experimental methods allowing one to verify the resulting model. Experimental approach using electrospray ionization mass spectrometry (ESI-MS) may be a powerful means for the host–guest complexation forecasting and complementary theoretical calculation using the molecular mechanical models.
ESI-MS is widely used for studying of noncovalent interactions between proteins and other biomolecules [15–17]. The soft ionization procedure permits the molecular complexes to retain “conformational memory” of their solution state structures in the gas phase [18]. Over the past decades, the rapidly increasing number of papers on the noncovalent interactions assessment using MS indicated that MS had become an important method due to high throughput, sensitivity and small sample volumes [19]. MS was used for the host–guest complexation assessment [20–25].
The noncovalent interactions between fluconazole and amino acids as elementary stages of the protein–inhibitor complex formation have not been previously studied.
The study was aimed to investigate the fluconazole-amino acid complexes in the gas phase using ESI-MS, as well as to assess the complexes structural and energy parameters using molecular dynamics and ab initio quantum chemistry methods.
METHODS
Fluconazole (Fluc), alanine (Ala), lysine (Lys), threonine (Thr), and methanol (HPLC grade) were obtained from Merck (Merck; Germany). Deionized water was prepared using the Milli-Q Reference Water Purification System (Merck; France). Each amino acid and fluconazole stock solution was prepared in methanol at a concentration of 10 mmol/mL. For mass spectrometry of individual compounds, the stock solutions were diluted with water to a concentration of 1 mmol/mL. The samples for the fluconazole-amino acid complexes analysis by tandem mass spectrometry were prepared by mixing the stock solutions of fluconazole and one of the amino acids in the 1 : 1 ratio (v : v). The solutions so obtained were diluted with water to a concentration of 1 mmol/mL for each component and stirred during 30 s. The sample for comparison of the fluconazole– amino acid complexes relative peak intensities was prepared by mixing the equal volumes of fluconazole, alanine, lysine and threonine stock solutions. The resulting solution was diluted with water to a concentration of 1 mmol/mL for each component and stirred during 30 s. All samples were prepared at room temperature.
The experiment was carried out using the MaxisImpact hybrid quadrupole time-of-flight (QTOF) mass spectrometer (BrukerDaltonics; Germany). The following parameters were used in the positive ion mode: mass range (m/z) 50–1000, needle voltage 4.5 kV, nebulizer gas pressure 0.6 bar, drying gas flow rate 5.0 L/min, drying gas temperature 200 °С. The tandem mass spectrometry experiments were carried out using the isolation window 3 m/z and the collision energy variation. The sample was injected directly using the KD Scientific syringe pump (KD Scientific; USA), the flow rate was 3 μL/min.
The molecular dynamics study of the Fluc + AA complexes was performed using the NAMD software [26]. The force field for the studied complexes modeling was created using the CHARMM General Force-Field (v3.1) basis [27, 28]. The types of atoms and partially the charges of the fluconazole atoms were selected by analogy. The missing parameters of bonds, angles and dihedral angles, as well as the missing charges of the fluconazole atoms were optimized using the Force Field Toolkit (ffTK), the VMD software plugin [26].
The quantum chemistry calculations of the fluconazole– amino acid complexes structure and energy parameters were performed using the GAMESS software package [29]. The complexes geometry optimization aimed at structural and energy properties study was carried out using the density-functional theory (DFT) by B3LYP calculations (3-21G and 6-311++G** basis sets with diffuse and polarization functions).
RESULTS
During the ESI-MS analysis of individual amino acids, the peaks corresponding to protonated and sodiated amino acids were identified. ESI-MS experiments with aqueous solutions containing both amino acid and fluconazole revealed the associates of amino acid and drug molecules, which indicated the sufficient stability of such complexes in the gas phase.
fig. 1 provides the positive ion ESI mass spectrum of the aqueous solution containing all three amino acids and fluconazole at equimolar concentrations. The possibility of the associates formation is indicated by the following peaks:
[Fluc + Ala + H]+ (m/z 396), [Fluc + Lys + H]+ (m/z 453) and [Fluc + Thr + H]+ (m/z 426). Taking into account the corresponding peaks intensities, the following sequence can be formed (tab. 1): [Fluc + Ala + H]+ < [Fluc + Thr + H]+ < [Fluc + Lys + H]+. Since the mass spectral peaks intensity is in proportion to the number of corresponding ions reaching the detector, it can be assumed that the described sequence is also valid for the interaction energy of the complex. However, in addition to stability, the intensity of the associate ion is also contributed by its ionization efficiency, stability of individual components of the complex, etc. For the additional experimental assessment of the complexes stability and interaction energy, the tandem mass spectra obtained as a result of the studied associates collision-induced dissociation have been acquired and analyzed. fig. 2 provides the complexes [Fluc + Ala + H]+ (fig. 2А), [Fluc + Thr + H]+ (fig. 2B) and [Fluc + Lys + H]+ (fig. 2С) fragmentation mass spectra. The complexes peaks, as well as the complexes fragments peaks resulting from protonated fluconazole (in case of alanine and threonine associates fragmentation) and protonated lysine (in case of fluconazole associate fragmentation) have been identified in the mass spectra. Thus, at this stage, it can be concluded that the studied complexes fragmentation proceeds along two basic lines: either the neutral amino acid, or the neutral fluconazole loss. Apparently, that is due to the described molecules different proton affinity. To quantify the studied complexes stability, the peak intensities of the tandem mass spectra with the collision energy variation have been analyzed. fig. 3 provides the correlation between the intensity of the precursor ion and the collision energy. The relative stability of fluconazole and various amino acids associates was evaluated by comparison of energy needed for the precursor ion peak intensity reduction by 50%. These values were 23, 25 and 29 eV for the studied complexes containing alanine, lysine and threonine respectively (:tab_1).
Thus, in the mass spectra of the fluconazole and alanine, threonine, lysine containing solutions the [Fluc + AA + H]+ clusters were identified. Since the experimental evaluation of the complexes stability was possible, it was of interest to estimate the energy of fluconazole interaction with each of the studied amino acids, which was the subject of the molecular dynamics modeling and quantum chemical calculations described below.
The initial structure selection plays a vital part in the molecular geometry optimization by quantum chemistry. Since both amino acids and fluconazole have a large number of degrees of freedom and are conformationally mobile, in our study, the selection of the initial structure for quantum-chemical calculations was carried out using molecular dynamics by simulated annealing. The selection of the molecule (fluconazole or amino acid) being a proton acceptor was performed based on the tandem mass spectra (see fig. 2). In the [Fluc + Ala + H]+ and [Fluc + Thr + H]+ complexes the fluconazole was protonated. In the [Fluc + Lys + H]+ complex the proton was localized at the lysine. The resulting structures obtained by simulated annealing were optimized using the B3LYP method (first with 3-21G basis set, and then with 6-311++G** basis set). The complex structures obtained by optimization are provided in fig. 4. In the [Fluc + Ala + H]+ and [Fluc + Thr + H]+complexes the geometry optimization led to the proton shift towards fluconazole. In the [Fluc + Lys + H]+ complex the proton was localized at the lysine, which was consistent with the hypothesis announced based on the experimental data. Such amino acids behavior might be due to amino acids and fluconazole different proton affinity, as well as to pI value, which was 9.74 for lysine, and 6 and 5.6 for alanine and threonine respectively. Based on the modeling, the complex formation energies were calculated using the formula IE = EAB – EA – EB, where EAB was the complex energy, EA and EB were the complex components energies. tab. 2 provides energy values obtained by quantum chemistry calculations using the B3LYP/3-21 and B3LYP/6-311++G** methods. The interaction energies between the components of the complex calculated using the B3LYP/6-311++G** method matched the sequence experimentally obtained. When using the B3LYP/3-21 method, the energy of fluconazole interaction with lysine was higher than that with threonine. That may indicate the importance of using diffuse and polarization functions when modeling the noncovalent complexes.
DISCUSSION
Previously, the importance of understanding the range of amino acids involved in binding of triazine compounds and the character of interactions determining the stability of the protein–drug complexes was shown [11]. The same paper provided the comparison of fluconazole interactions with various CYP51 enzymes. In such complexes, the interaction energy resulted from the competitive binding of ligand with amino acid residues of the protein, heme and water molecules. The protein–fluconazole binding proved to be more effective in mitochondrial CYP51 due to polar interaction with the arginine residue. However, in fungal enzymes these interactions are substituted by ligand–heme interactions, which compensate for the loss of the polar bond and result in the more energetically favored binding. Therefore, the development of ligand with increased specificity to fungal enzymes should focus on the strengthening the polar ligand–heme interactions. The larger hydrophobic ligands are probably best suited to target the mycobacterial and human enzymes [11]. Such studies demonstrate the importance of understanding and considering the interactions between the drug and the various components of the target. This paper provides the approach, which may be used at the early stages of the new drugs development, making it possible to estimate the drug–amino acid complexes stability both from the experimental and theoretical point of view.
CONCLUSION
ESI-MS and molecular dynamics were used for the study of fluconazole–amino acid interactions, which, according to literary sources, played a vital part in the protein–inhibitor binding. The noncovalent complexes turned out to be stablein the gas phase, which made it possible to identify the corresponding signals in the mass spectra. Tandem mass spectrometry with varying collision energy was used for the studied complexes stability assessment. The following stability sequence was obtained: [Fluc + Ala + H]+ (23 eV) < [Fluc + Lys + H]+ (25 eV) < [Fluc + Thr + H]+ (29 eV). Molecular dynamics and quantum chemical modeling made it possible to define the complexes structure and interaction energy. The interaction energy values determined using the B3LYP/6-311++G** method formed the associates stability sequence similar to that obtained by experiment. Such approach combining the experimental mass spectrometry studies and the quantum chemical modeling may be used at the early stages of the new drugs development, and to search for the drug action molecular mechanisms.