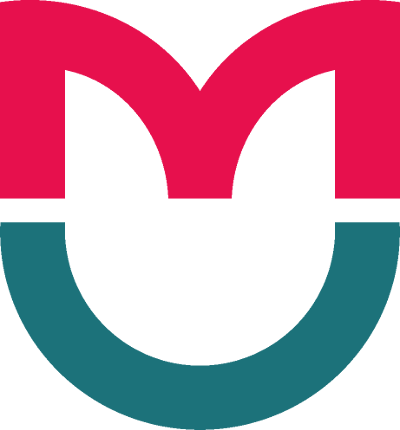
ОБЗОР
Генетические аспекты этиологии билиарной атрезии
1 Научный центр акушерства, гинекологии и перинатологии имени академика В. И. Кулакова, Москва, Россия
2 Российский национальный исследовательский медицинский университет имени Н. И. Пирогова, Москва, Россия
3 Первый Московский государственный медицинский университет имени И. М. Сеченова, Москва, Россия
Для корреспонденции: Медан Хасановна Исаева
ул. Академика Опарина, д. 4Б, г. Москва, 117513; ur.kb@aveyasi.nadem
Финансирование: работа была поддержана грантом №075-15-2019-1789 Министерства образования и науки РФ, выделенным на осуществление государственной поддержки создания и развития «Центра высокоточного редактирования и генетических технологий для биомедицины».
Вклад авторов: М. Х. Исаева, В. А. Белова — изучение литературы, написание обзора; Д. О. Коростин, А. В. Дегтярева — вклад в концепцию и структуру обзора, редактирование.
- Davenport M, et al. Biliary atresia in England and Wales: results of centralization and new benchmark. Journal of Pediatric Surgery. 2011; 46 (9): 1689–94.
- Tiao MM, et al. Epidemiological features of biliary atresia in Taiwan, a national study 1996–2003. Journal of gastroenterology and hepatology. 2008; 23 (1): 62–66.
- Волынец Г. В. и др. Дифференциальная диагностика врожденных холестатических болезней у детей. Экспериментальная и клиническая гастроэнтерология. 2017; 8 (144): 67–74.
- Филиппова Е. А. и др. Эхографические изменения органов брюшной полости у детей с билиарной атрезией и синдромом Алажиля в течение первых 3 месяцев жизни. Педиатрия. Приложение к журналу Consilium Medicum. 2017; 4, 93.
- Разумовский А. Ю., Дегтярева А. В., Куликова Н. В., Рачков В. Е., Ратников С. А., Филиппова Е. А. и др. Отдаленные результаты лечения детей с билиарной атрезией. Российский вестник перинатологии и педиатрии. 2019; 64 (1): 46–55.
- Козлов Ю. А. и др. Современный взгляд на происхождение билиарной атрезии. Анналы хирургии. 2017; 22 (2): 73–80.
- Ortiz-Perez A, et al. Innate Immunity and Pathogenesis of Biliary Atresia. Frontiers in Immunology. 2020; 11: 329.
- Lampela H, et al. Native liver histology after successful portoenterostomy in biliary atresia. Journal of clinical gastroenterology. 2014; 48 (8): 721–8.
- Soufi N, Bazerbachi F, Deneau M. Post-transplant disease recurrence in pediatric PSC. Current gastroenterology reports. 2018; 20 (9): 44.
- Vij M, Rela M. Biliary atresia: pathology, etiology and pathogenesis. Future Science OA. 2020; 00: FSO466. https://www.future-science.com/doi/full/10.2144/fsoa-2019-0153
- Averbukh LD, Wu GY. Evidence for viral induction of biliary atresia: a review. Journal of Clinical and Translational Hepatology. 2018; 6 (49): 410.
- Rauschenfels S, et al. Incidence of hepatotropic viruses in biliary atresia. European journal of pediatrics. 2009; 168 (4): 469–76.
- Zani A, et al. Cytomegalovirus-associated biliary atresia: an aetiological and prognostic subgroup. Journal of pediatric surgery. 2015; 50 (910): 1739–45.
- Sergi CM. Genetics of biliary atresia: a work in progress for a disease with an unavoidable sequela into liver cirrhosis following failure of hepatic portoenterostomy. Liver Cirrhosis-Debates and Current Challenges. IntechOpen, 2019. Доступно по ссылке: https://www.intechopen.com/books/liver-cirrhosis-debates-and-current-challenges/genetics-of-biliary-atresia-a-work-in-progress-for-a-disease-with-an-unavoidable-sequela-into-liver-.
- Lakshminarayanan B, Davenport M. Biliary atresia: a comprehensive review. Journal of autoimmunity. 2016; 73: 1–9.
- Lorent K, et al. Identification of a plant isoflavonoid that causes biliary atresia. Science translational medicine. 2015; 7 (286): 286ra67-286ra67.
- Zhao X, et al. Glutathione antioxidant pathway activity and reserve determine toxicity and specificity of the biliary toxin biliatresone in zebrafish. Hepatology. 2016; 64 (3): 894–907.
- Kobayashi K, et al. Mother-to-daughter occurrence of biliary atresia: a case report. Journal of pediatric surgery. 2008; 43 (8): 1566–8.
- Girard M, Panasyuk G. Genetics in biliary atresia. Current opinion in gastroenterology. 2019; 35 (2): 73–81.
- 20. Xu X, Zhan J. Biliary atresia in twins: a systematic review and meta-analysis. Pediatric Surgery International, 2020. Доступно по ссылке: https://link.springer.com/article/10.1007/s00383- 020-04690-4.
- Gou Q, et al. Biliary atresia in twins’ population: a retrospective multicenter study in mainland China. Pediatric Surgery International. 2020; p. 1–8.
- Fabre A, Roman C, Roquelaure B. Somatic mutation, a cause of biliary atresia: A hypothesis. Medical Hypotheses. 2017; 102: 91–93.
- Leyva‐Vega M, et al. Genomic alterations in biliary atresia suggest region of potential disease susceptibility in 2q37. 3. American journal of medical genetics Part A. 2010; 152 (4): 886–95.
- Cui S, et al. Evidence from human and zebrafish that GPC1 is a biliary atresia susceptibility gene. Gastroenterology. 2013; 144 (5): 1107–15.
- Ningappa M, et al. The role of ARF6 in biliary atresia. PloS one. 2015; 10 (9): e0138381.
- Garcia-Barceló MM, et al. Genome-wide association study identifies a susceptibility locus for biliary atresia on 10q24. 2. Human molecular genetics. 2010; 19 (14): 2917–25.
- Tsai EA, et al. Replication of a GWAS signal in a Caucasian population implicates ADD3 in susceptibility to biliary atresia. Human genetics. 2014; 133 (2): 235–43.
- Kaewkiattiyot S, et al. Association of X‐prolyl aminopeptidase 1 rs17095355 polymorphism with biliary atresia in Thai children. Hepatology Research. 2011; 41 (12): 1249–52.
- Chen Y, et al. A genome-wide association study identifies a susceptibility locus for biliary atresia on 2p16. 1 within the gene EFEMP1. PLoS genetics. 2018; 14 (8): e1007532.
- Ke J, et al. Common genetic variants of GPC1 gene reduce risk of biliary atresia in a Chinese population. Journal of pediatric surgery. 2016; 51 (10): 1661–4.
- Citterio L, et al. Expression analysis of the human adducin gene family and evidence of ADD2 β4 multiple splicing variants. Biochemical and biophysical research communications. 2003; 309 (2): 359–67.
- Ku NO, et al. The cytoskeleton of digestive epithelia in health and disease. American Journal of Physiology-Gastrointestinal and Liver Physiology. 1999; 277 (6): G1108–G1137.
- Oshio C, Phillips MJ. Contractility of bile canaliculi: implications for liver function. Science. 1981; 212 (4498): 1041–2.
- Segawa O, et al. Actin and myosin deposition around bile canaliculi: a predictor of clinical outcome in biliary atresia. Journal of pediatric surgery. 1993; 28 (6): 851–6.
- Shteyer E, et al. Outcome after portoenterostomy in biliary atresia: pivotal role of degree of liver fibrosis and intensity of stellate cell activation. Journal of pediatric gastroenterology and nutrition. 2006; 42 (1): 93–99.
- Nagasaka T, et al. Immunohistochemical localization of placental leucine aminopeptidase/oxytocinase in normal human placental, fetal and adult tissues. Reproduction, fertility and development. 1997; 9 (8): 747–54.
- Hooper NM, et al. Protease-activated receptors: the role of cell-surface proteolysis in signalling. Essays in Biochemistry. 2002; 38: 169–83.
- Sharma JN. Hypertension and the bradykinin system. Current hypertension reports. 2009; 11 (3): 178–81.
- Zhao A, et al. Human kininogen gene is transactivated by the farnesoid X receptor. Journal of Biological Chemistry. 2003; 278 (31): 28765–70.
- Yang H, et al. Inflammation mediated down-regulation of hepatobiliary transporters contributes to intrahepatic cholestasis and liver damage in murine biliary atresia. Pediatric research. 2009; 66 (4): 380–5.
- Tsai EA, et al. Replication of a GWAS signal in a Caucasian population implicates ADD3 in susceptibility to biliary atresia. Human genetics. 2014; 133 (2): 235–43.
- Kaewkiattiyot S, et al. Association of X‐prolyl aminopeptidase 1 rs17095355 polymorphism with biliary atresia in Thai children. Hepatology Research. 2011; 41 (12): 1249–52.
- Cheng G, et al. Common genetic variants regulating ADD3 gene expression alter biliary atresia risk. Journal of hepatology. 2013; 59 (6): 1285–91.
- Zeng S, et al. Association between single nucleotide polymorphisms in the ADD3 gene and susceptibility to biliary atresia. PloS one. 2014; 9 (10): e107977.
- Wang Z, et al. The intragenic epistatic association of ADD3 with biliary atresia in Southern Han Chinese population. Bioscience reports. 2018; 38 (3). Доступно по ссылке: https:// portlandpress.com/bioscirep/article/38/3/BSR20171688/57837/ The-intragenic-epistatic-association-of-ADD3-with.
- Laochareonsuk W, Chiengkriwate P, Sangkhathat S. Single nucleotide polymorphisms within Adducin 3 and Adducin 3 antisense RNA1 genes are associated with biliary atresia in Thai infants. Pediatric surgery international. 2018; 34 (5): 515–20.
- Jing Li, et al. Association between rs17095355 polymorphism on 10q24 and susceptibility to biliary atresia: a meta-analysis. The Journal of Maternal-Fetal & Neonatal Medicine. 2017; 30 (15): 1882–6.
- Tang, Vivian, et al. Loss of a candidate biliary atresia susceptibility gene, add3a, causes biliary developmental defects in zebrafish. Journal of pediatric gastroenterology and nutrition. 2016; 63 (5): 524.
- Iguchi H, et al. cAMP response element-binding protein (CREB) is required for epidermal growth factor (EGF)-induced cell proliferation and serum response element activation in neural stem cells isolated from the forebrain subventricular zone of adult mice. Endocrine journal. 2011: 1106100583-1106100583.
- Sarró E, et al. Phosphoinositide 3-kinase inhibitors protect mouse kidney cells from cyclosporine-induced cell death. Kidney international. 2008; 73 (1): 77–85.
- Hu ZZ, et al. GEP100/Arf6 is required for epidermal growth factor-induced ERK/Rac1 signaling and cell migration in human hepatoma HepG2 cells. PloS one. 2012; 7 (6): e38777.
- Sabe H, et al. The EGFR-GEP100-Arf6-AMAP1 signaling pathway specific to breast cancer invasion and metastasis. Traffic. 2009; 10 (8): 982–93.
- Azar G, et al. Atypical morphologic presentation of biliary atresia and value of serial liver biopsies. Journal of pediatric gastroenterology and nutrition. 2002; 34 (2): 212–5.
- Landing BH, Wells TR, Ramicone E. Time course of the intrahepatic lesion of extrahepatic biliary atresia: a morphometric study. Pediatric pathology. 1985; 4 (3–4): 309–19.
- Chen Y, et al. A genome-wide association study identifies a susceptibility locus for biliary atresia on 2p16. 1 within the gene EFEMP1. PLoS genetics. 2018; 14 (8): e1007532.
- De Vega S, Iwamoto T, Yamada Y. Fibulins: multiple roles in matrix structures and tissue functions. Cellular and molecular life sciences. 2009; 66 (11–12): 1890–902.
- Timpl R, et al. Fibulins: a versatile family of extracellular matrix proteins. Nature reviews Molecular cell biology. 2003; 4 (6): 479–89.
- Hu B, et al. Fibulin-3 promotes glioma growth and resistance through a novel paracrine regulation of Notch signaling. Cancer research. 2012; 72 (15): 3873–85.
- Bai MR, et al. Association of common variation in ADD3 and GPC1 with biliary atresia susceptibility. Aging (Albany NY). 2020; 12 (8): 7163.
- Tian L, et al. Biliary atresia relevant human induced pluripotent stem cells recapitulate key disease features in a dish. Journal of pediatric gastroenterology and nutrition. 2019; 68 (1): 56.
- Rajagopalan R, et al. exome Sequencing in individuals with isolated Biliary Atresia. Scientific reports. 2020; 10 (1): 1–8.
- Zhao X, et al. Impaired redox and protein homeostasis as risk factors and therapeutic targets in toxin-induced biliary atresia. Gastroenterology. 2020. Available from: https://www. sciencedirect.com/science/article/pii/S0016508520347545.
- Lam WY, et al. Whole exome sequencing reveals a wide spectrum of ciliary gene mutations in nonsyndromic biliary atresia. medRxiv. 2020. Доступно по ссылке: https://www.medrxiv.org/content/1 0.1101/2020.05.05.20091504v1.full.pdf.
- Petersen C, Davenport M. Aetiology of biliary atresia: what is actually known? Orphanet journal of rare diseases. 2013; 8 (1): 128.
- Cui S, et al. Evidence from human and zebrafish that GPC1 is a biliary atresia susceptibility gene. Gastroenterology. 2013; 144 (5): 1107–15.
- Rock N, McLin V. Liver involvement in children with ciliopathies. Clinics and research in hepatology and gastroenterology. 2014; 38 (4): 407–14.
- Miethke AG, Huppert SS. Fishing for biliary atresia susceptibility genes. Gastroenterology. 2013; 144 (5): 878.
- Zhao D, Long XD, Xia Q. Recent advances in etiology of biliary atresia. Clinical pediatrics. 2015; 54 (8): 723–31.
- Campbell KM, et al. High prevalence of α-1-antitrypsin heterozygosity in children with chronic liver disease. Journal of pediatric gastroenterology and nutrition. 2007; 44 (1): 99–103.
- Sangkhathat S, et al. Variants associated with infantile cholestatic syndromes detected in extrahepatic biliary atresia by whole exome studies: a 20-case series from Thailand. Journal of pediatric genetics. 2018; 7 (2): 67.
- Kohsaka T, et al. The significance of human jagged 1 mutations detected in severe cases of extrahepatic biliary atresia. Hepatology. 2002; 36 (4): 904–12.
- Dědič T, et al. Alagille syndrome mimicking biliary atresia in early infancy. PLoS One. 2015; 10 (11): e0143939.
- Linton KJ. Lipid flopping in the liver. Biochemical Society Transactions. 2015; 43 (5): 1003–10.
- Groen A, et al. Complementary functions of the flippase ATP8B1 and the floppase ABCB4 in maintaining canalicular membrane integrity. Gastroenterology. 2011; 141 (5): 1927–37.
- Chen HL, et al. Expression of hepatocyte transporters and nuclear receptors in children with early and late-stage biliary atresia. Pediatric research. 2008; 63 (6): 667–3.
- Chen HL, et al. Jaundice revisited: recent advances in the diagnosis and treatment of inherited cholestatic liver diseases. Journal of biomedical science. 2018; 25 (1): 75.
- Wagner M, et al. Role of farnesoid X receptor in determining hepatic ABC transporter expression and liver injury in bile duct-ligated mice. Gastroenterology. 2003; 125 (3): 825–38.
- Stedman CAM, et al. Nuclear receptors constitutive androstane receptor and pregnane X receptor ameliorate cholestatic liver injury. Proceedings of the National Academy of Sciences. 2005; 102 (6): 2063–8.
- Wagner M, et al. CAR and PXR agonists stimulate hepatic bile acid and bilirubin detoxification and elimination pathways in mice. Hepatology. 2005; 42 (2): 420–30.
- Makishima M. Nuclear receptors as targets for drug development: regulation of cholesterol and bile acid metabolism by nuclear receptors. Journal of pharmacological sciences. 2005; 97 (2): 177–83.
- Boyer JL. Nuclear receptor ligands: rational and effective therapy for chronic cholestatic liver disease? Gastroenterology. 2005; 129 (2): 735–40.
- Keppler D. The roles of MRP2, MRP3, OATP1B1, and OATP1B3 in conjugated hyperbilirubinemia. Drug Metabolism and Disposition. 2014; 42 (4): 561–5.
- Schroeder RJ, et al. Cholesterol and sphingolipid enhance the Triton X-100 insolubility of glycosylphosphatidylinositol-anchored proteins by promoting the formation of detergent-insoluble ordered membrane domains. Journal of Biological Chemistry. 1998; 273 (2): 1150–7.
- Guyot C, Stieger B. Interaction of bile salts with rat canalicular membrane vesicles: evidence for bile salt resistant microdomains. Journal of hepatology. 2011; 55 (6): 1368–76.
- Linton KJ. Lipid flopping in the liver. Biochemical Society Transactions. 2015; 43 (5): 1003–10.
- Groen A, et al. Complementary functions of the flippase ATP8B1 and the floppase ABCB4 in maintaining canalicular membrane integrity. Gastroenterology. 2011; 141 (5): 1927–37.
- Wang H, et al. Endogenous bile acids are ligands for the nuclear receptor FXR/BAR. Molecular cell. 1999; 3 (5): 543–53.
- Parks DJ, et al. Bile acids: natural ligands for an orphan nuclear receptor. Science. 1999; 284 (5418): 1365–8.
- Makishima M, et al. Identification of a nuclear receptor for bile acids. Science. 1999; 284 (5418): 1362–5.
- Modica S, Gadaleta RM, Moschetta A. Deciphering the nuclear bile acid receptor FXR paradigm. Nuclear receptor signaling. 2010; 8 (1): nrs. 08005. Available from: https://journals.sagepub. com/doi/pdf/10.1621/nrs.08005.
- Mezina A, et al. 845 Whole Exome Sequencing Identifies ABCB4 Gene Variants As Modifiers of Biliary Atresia Outcomes. Gastroenterology. 2014; 146 (5): S-928.
- Ramachandran P, et al. RNA-seq reveals outcome-specific gene expression of MMP7 and PCK1 in biliary atresia. Molecular biology reports. 2019; 46 (5): 5123–30.
- Matthews RP, et al. DNA hypomethylation causes bile duct defects in zebrafish and is a distinguishing feature of infantile biliary atresia. Hepatology. 2011; 53 (3): 905–14.
- Dong R, Zhao R, Zheng S. Changes in epigenetic regulation of CD4+ T lymphocytesin biliary atresia. Pediatric research. 2011; 70 (6): 555–9.
- Dong R, et al. Abnormal DNA methylation of ITGAL (CD11a) in CD4+ T cells from infants with biliary atresia. Biochemical and biophysical research communications. 2012; 417 (3): 986–90.
- Udomsinprasert W, et al. Global methylation, oxidative stress, and relative telomere length in biliary atresia patients. Scientific reports. 2016; 6: 26969.
- Li K, et al. Foxp3 promoter methylation impairs suppressive function of regulatory T cells in biliary atresia. American Journal of Physiology-Gastrointestinal and Liver Physiology. 2016; 311 (6): G989–G997.
- Cofer ZC, et al. Methylation microarray studies highlight PDGFA expression as a factor in biliary atresia. PloS one. 2016; 11 (3): e0151521.
- Liu F, et al. PDGFA gene rs9690350 polymorphism increases biliary atresia risk in Chinese children. Bioscience reports. 202; 40 (7). Available from: https://portlandpress.com/bioscirep/ article/40/7/BSR20200068/225782/PDGFA-gene-rs9690350- polymorphism-increases.
- Yang Y, et al. MicroRNA-29b/142-5p contribute to the pathogenesis of biliary atresia by regulating the IFN-γ gene. Cell death & disease. 2018; 9 (5): 1–9.
- Ye Y, et al. Downregulation of microRNA-145 may contribute to liver fibrosis in biliary atresia by targeting ADD3. PloS one. 2017; 12 (9): e0180896.