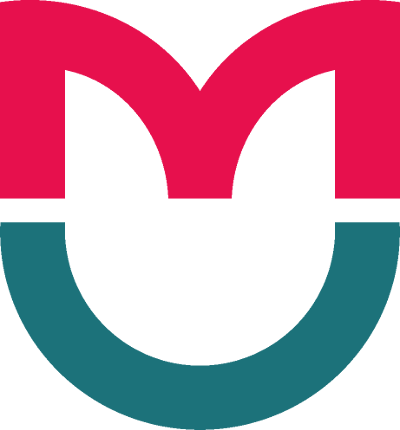
This article is an open access article distributed under the terms and conditions of the Creative Commons Attribution license (CC BY).
REVIEW
Genetic aspects of biliary atresia etiology
1 Academician V. I. Kulakov Research Center for Obstetrics, Gynecology and Perinatology, Moscow, Russia
2 Pirogov Russian National Research Medical University, Moscow, Russia
3 Sechenov First Moscow State Medical University, Moscow, Russia
Correspondence should be addressed: Medan Kh. Isaeva
Oparina street 4B, Moscow, 117513; ur.kb@aveyasi.nadem
Funding: the study was supported by grant № 075-15-2019-1789 of the Ministry of Science and Higher Education of the Russian Federation issued to ensure state support for the Center for Precision Genome Editing and Genetic Technologies for Biomedicine.
Author contribution: Isaeva MKh, Belova VA — literature analysis, manuscript writing; Korostin DO, Degtyareva AV — concept and structure of the review, manuscript editing.
- Davenport M, et al. Biliary atresia in England and Wales: results of centralization and new benchmark. Journal of Pediatric Surgery. 2011; 46 (9): 1689–94.
- Tiao MM, et al. Epidemiological features of biliary atresia in Taiwan, a national study 1996–2003. Journal of gastroenterology and hepatology. 2008; 23 (1): 62–66.
- Volynec GV, i dr. Differencial'naja diagnostika vrozhdennyh holestaticheskih boleznej u detej. Jeksperimental'naja i klinicheskaja gastrojenterologija. 2017; 8 (144): 67–74. Russian.
- Filippova EA, i dr. Jehograficheskie izmenenija organov brjushnoj polosti u detej s biliarnoj atreziej i sindromom Alazhilja v techenie pervyh 3 mesjacev zhizni. Pediatrija. Prilozhenie k zhurnalu Consilium Medicum. 2017; 4, 93. Russian.
- Razumovskij AYu, Degtjareva AV, Kulikova NV, Rachkov VE, Ratnikov S A, Filippova EA, i dr. Otdalennye rezul'taty lechenija detej s biliarnoj atreziej. Rossijskij vestnik perinatologii i pediatrii. 2019; 64 (1): 46–55. Russian.
- Kozlov YuA, i dr. Sovremennyj vzgljad na proishozhdenie biliarnoj atrezii. Annaly hirurgii. 2017; 22 (2): 73–80. Russian.
- Ortiz-Perez A, et al. Innate Immunity and Pathogenesis of Biliary Atresia. Frontiers in Immunology. 2020; 11: 329.
- Lampela H, et al. Native liver histology after successful portoenterostomy in biliary atresia. Journal of clinical gastroenterology. 2014; 48 (8): 721–8.
- Soufi N, Bazerbachi F, Deneau M. Post-transplant disease recurrence in pediatric PSC. Current gastroenterology reports. 2018; 20 (9): 44.
- Vij M, Rela M. Biliary atresia: pathology, etiology and pathogenesis. Future Science OA. 2020; 00: FSO466. https://www.future-science.com/doi/full/10.2144/fsoa-2019-0153
- Averbukh LD, Wu GY. Evidence for viral induction of biliary atresia: a review. Journal of Clinical and Translational Hepatology. 2018; 6 (49): 410.
- Rauschenfels S, et al. Incidence of hepatotropic viruses in biliary atresia. European journal of pediatrics. 2009; 168 (4): 469–76.
- Zani A, et al. Cytomegalovirus-associated biliary atresia: an aetiological and prognostic subgroup. Journal of pediatric surgery. 2015; 50 (910): 1739–45.
- Sergi CM. Genetics of biliary atresia: a work in progress for a disease with an unavoidable sequela into liver cirrhosis following failure of hepatic portoenterostomy. Liver Cirrhosis-Debates and Current Challenges. IntechOpen, 2019. Dostupno po ssylke: https://www.intechopen.com/books/liver-cirrhosis-debates-and-current-challenges/genetics-of-biliary-atresia-a-work-in-progress-for-a-disease-with-an-unavoidable-sequela-into-liver-.
- Lakshminarayanan B, Davenport M. Biliary atresia: a comprehensive review. Journal of autoimmunity. 2016; 73: 1–9.
- Lorent K, et al. Identification of a plant isoflavonoid that causes biliary atresia. Science translational medicine. 2015; 7 (286): 286ra67-286ra67.
- Zhao X, et al. Glutathione antioxidant pathway activity and reserve determine toxicity and specificity of the biliary toxin biliatresone in zebrafish. Hepatology. 2016; 64 (3): 894–907.
- Kobayashi K, et al. Mother-to-daughter occurrence of biliary atresia: a case report. Journal of pediatric surgery. 2008; 43 (8): 1566–8.
- Girard M, Panasyuk G. Genetics in biliary atresia. Current opinion in gastroenterology. 2019; 35 (2): 73–81.
- Xu X, Zhan J. Biliary atresia in twins: a systematic review and meta-analysis. Pediatric Surgery International, 2020. Available from: https://link.springer.com/article/10.1007/s00383-020- 04690-4.
- Gou Q, et al. Biliary atresia in twins’ population: a retrospective multicenter study in mainland China. Pediatric Surgery International. 2020; p. 1–8.
- Fabre A, Roman C, Roquelaure B. Somatic mutation, a cause of biliary atresia: A hypothesis. Medical Hypotheses. 2017; 102: 91–93.
- Leyva‐Vega M, et al. Genomic alterations in biliary atresia suggest region of potential disease susceptibility in 2q37. 3. American journal of medical genetics Part A. 2010; 152 (4): 886–95.
- Cui S, et al. Evidence from human and zebrafish that GPC1 is a biliary atresia susceptibility gene. Gastroenterology. 2013; 144 (5): 1107–15.
- Ningappa M, et al. The role of ARF6 in biliary atresia. PloS one. 2015; 10 (9): e0138381.
- Garcia-Barceló MM, et al. Genome-wide association study identifies a susceptibility locus for biliary atresia on 10q24. 2. Human molecular genetics. 2010; 19 (14): 2917–25.
- Tsai EA, et al. Replication of a GWAS signal in a Caucasian population implicates ADD3 in susceptibility to biliary atresia. Human genetics. 2014; 133 (2): 235–43.
- Kaewkiattiyot S, et al. Association of X‐prolyl aminopeptidase 1 rs17095355 polymorphism with biliary atresia in Thai children. Hepatology Research. 2011; 41 (12): 1249–52.
- Chen Y, et al. A genome-wide association study identifies a susceptibility locus for biliary atresia on 2p16. 1 within the gene EFEMP1. PLoS genetics. 2018; 14 (8): e1007532.
- Ke J, et al. Common genetic variants of GPC1 gene reduce risk of biliary atresia in a Chinese population. Journal of pediatric surgery. 2016; 51 (10): 1661–4.
- Citterio L, et al. Expression analysis of the human adducin gene family and evidence of ADD2 β4 multiple splicing variants. Biochemical and biophysical research communications. 2003; 309 (2): 359–67.
- Ku NO, et al. The cytoskeleton of digestive epithelia in health and disease. American Journal of Physiology-Gastrointestinal and Liver Physiology. 1999; 277 (6): G1108–G1137.
- Oshio C, Phillips MJ. Contractility of bile canaliculi: implications for liver function. Science. 1981; 212 (4498): 1041–2.
- Segawa O, et al. Actin and myosin deposition around bile canaliculi: a predictor of clinical outcome in biliary atresia. Journal of pediatric surgery. 1993; 28 (6): 851–6.
- Shteyer E, et al. Outcome after portoenterostomy in biliary atresia: pivotal role of degree of liver fibrosis and intensity of stellate cell activation. Journal of pediatric gastroenterology and nutrition. 2006; 42 (1): 93–99.
- Nagasaka T, et al. Immunohistochemical localization of placental leucine aminopeptidase/oxytocinase in normal human placental, fetal and adult tissues. Reproduction, fertility and development. 1997; 9 (8): 747–54.
- Hooper NM, et al. Protease-activated receptors: the role of cell-surface proteolysis in signalling. Essays in Biochemistry. 2002; 38: 169–83.
- Sharma JN. Hypertension and the bradykinin system. Current hypertension reports. 2009; 11 (3): 178–81.
- Zhao A, et al. Human kininogen gene is transactivated by the farnesoid X receptor. Journal of Biological Chemistry. 2003; 278 (31): 28765–70.
- Yang H, et al. Inflammation mediated down-regulation of hepatobiliary transporters contributes to intrahepatic cholestasis and liver damage in murine biliary atresia. Pediatric research. 2009; 66 (4): 380–5.
- Tsai EA, et al. Replication of a GWAS signal in a Caucasian population implicates ADD3 in susceptibility to biliary atresia. Human genetics. 2014; 133 (2): 235–43.
- Kaewkiattiyot S, et al. Association of X‐prolyl aminopeptidase 1 rs17095355 polymorphism with biliary atresia in Thai children. Hepatology Research. 2011; 41 (12): 1249–52.
- Cheng G, et al. Common genetic variants regulating ADD3 gene expression alter biliary atresia risk. Journal of hepatology. 2013; 59 (6): 1285–91.
- Zeng S, et al. Association between single nucleotide polymorphisms in the ADD3 gene and susceptibility to biliary atresia. PloS one. 2014; 9 (10): e107977.
- Wang Z, et al. The intragenic epistatic association of ADD3 with biliary atresia in Southern Han Chinese population. Bioscience reports. 2018; 38 (3). Dostupno po ssylke: https://portlandpress. com/bioscirep/article/38/3/BSR20171688/57837/The-intragenic-epistatic-association-of-ADD3-with.
- Laochareonsuk W, Chiengkriwate P, Sangkhathat S. Single nucleotide polymorphisms within Adducin 3 and Adducin 3 antisense RNA1 genes are associated with biliary atresia in Thai infants. Pediatric surgery international. 2018; 34 (5): 515–20.
- Jing Li, et al. Association between rs17095355 polymorphism on 10q24 and susceptibility to biliary atresia: a meta-analysis. The Journal of Maternal-Fetal & Neonatal Medicine. 2017; 30 (15): 1882–6.
- Tang, Vivian, et al. Loss of a candidate biliary atresia susceptibility gene, add3a, causes biliary developmental defects in zebrafish. Journal of pediatric gastroenterology and nutrition. 2016; 63 (5): 524.
- Iguchi H, et al. cAMP response element-binding protein (CREB) is required for epidermal growth factor (EGF)-induced cell proliferation and serum response element activation in neural stem cells isolated from the forebrain subventricular zone of adult mice. Endocrine journal. 2011: 1106100583-1106100583.
- Sarró E, et al. Phosphoinositide 3-kinase inhibitors protect mouse kidney cells from cyclosporine-induced cell death. Kidney international. 2008; 73 (1): 77–85.
- Hu ZZ, et al. GEP100/Arf6 is required for epidermal growth factor-induced ERK/Rac1 signaling and cell migration in human hepatoma HepG2 cells. PloS one. 2012; 7 (6): e38777.
- Sabe H, et al. The EGFR-GEP100-Arf6-AMAP1 signaling pathway specific to breast cancer invasion and metastasis. Traffic. 2009; 10 (8): 982–93.
- Azar G, et al. Atypical morphologic presentation of biliary atresia and value of serial liver biopsies. Journal of pediatric gastroenterology and nutrition. 2002; 34 (2): 212–5.
- Landing BH, Wells TR, Ramicone E. Time course of the intrahepatic lesion of extrahepatic biliary atresia: a morphometric study. Pediatric pathology. 1985; 4 (3–4): 309–19.
- 55. Chen Y, et al. A genome-wide association study identifies a susceptibility locus for biliary atresia on 2p16. 1 within the gene EFEMP1. PLoS genetics. 2018; 14 (8): e1007532.
- De Vega S, Iwamoto T, Yamada Y. Fibulins: multiple roles in matrix structures and tissue functions. Cellular and molecular life sciences. 2009; 66 (11–12): 1890–902.
- Timpl R, et al. Fibulins: a versatile family of extracellular matrix proteins. Nature reviews Molecular cell biology. 2003; 4 (6): 479–89.
- Hu B, et al. Fibulin-3 promotes glioma growth and resistance through a novel paracrine regulation of Notch signaling. Cancer research. 2012; 72 (15): 3873–85.
- Bai MR, et al. Association of common variation in ADD3 and GPC1 with biliary atresia susceptibility. Aging (Albany NY). 2020; 12 (8): 7163.
- Tian L, et al. Biliary atresia relevant human induced pluripotent stem cells recapitulate key disease features in a dish. Journal of pediatric gastroenterology and nutrition. 2019; 68 (1): 56.
- Rajagopalan R, et al. exome Sequencing in individuals with isolated Biliary Atresia. Scientific reports. 2020; 10 (1): 1–8.
- Zhao X, et al. Impaired redox and protein homeostasis as risk factors and therapeutic targets in toxin-induced biliary atresia. Gastroenterology. 2020. Available from: https://www. sciencedirect.com/science/article/pii/S0016508520347545.
- Lam WY, et al. Whole exome sequencing reveals a wide spectrum of ciliary gene mutations in nonsyndromic biliary atresia. medRxiv. 2020. Dostupno po ssylke: https://www.medrxiv.org/content/10 .1101/2020.05.05.20091504v1.full.pdf.
- Petersen C, Davenport M. Aetiology of biliary atresia: what is actually known? Orphanet journal of rare diseases. 2013; 8 (1): 128.
- Cui S, et al. Evidence from human and zebrafish that GPC1 is a biliary atresia susceptibility gene. Gastroenterology. 2013; 144 (5): 1107–15.
- Rock N, McLin V. Liver involvement in children with ciliopathies. Clinics and research in hepatology and gastroenterology. 2014; 38 (4): 407–14.
- Miethke AG, Huppert SS. Fishing for biliary atresia susceptibility genes. Gastroenterology. 2013; 144 (5): 878.
- Zhao D, Long XD, Xia Q. Recent advances in etiology of biliary atresia. Clinical pediatrics. 2015; 54 (8): 723–31.
- Campbell KM, et al. High prevalence of α-1-antitrypsin heterozygosity in children with chronic liver disease. Journal of pediatric gastroenterology and nutrition. 2007; 44 (1): 99–103.
- Sangkhathat S, et al. Variants associated with infantile cholestatic syndromes detected in extrahepatic biliary atresia by whole exome studies: a 20-case series from Thailand. Journal of pediatric genetics. 2018; 7 (2): 67.
- Kohsaka T, et al. The significance of human jagged 1 mutations detected in severe cases of extrahepatic biliary atresia. Hepatology. 2002; 36 (4): 904–12.
- Dědič T, et al. Alagille syndrome mimicking biliary atresia in early infancy. PLoS One. 2015; 10 (11): e0143939.
- Linton KJ. Lipid flopping in the liver. Biochemical Society Transactions. 2015; 43 (5): 1003–10.
- Groen A, et al. Complementary functions of the flippase ATP8B1 and the floppase ABCB4 in maintaining canalicular membrane integrity. Gastroenterology. 2011; 141 (5): 1927–37.
- Chen HL, et al. Expression of hepatocyte transporters and nuclear receptors in children with early and late-stage biliary atresia. Pediatric research. 2008; 63 (6): 667–3.
- Chen HL, et al. Jaundice revisited: recent advances in the diagnosis and treatment of inherited cholestatic liver diseases. Journal of biomedical science. 2018; 25 (1): 75.
- Wagner M, et al. Role of farnesoid X receptor in determining hepatic ABC transporter expression and liver injury in bile duct-ligated mice. Gastroenterology. 2003; 125 (3): 825–38.
- Stedman CAM, et al. Nuclear receptors constitutive androstane receptor and pregnane X receptor ameliorate cholestatic liver injury. Proceedings of the National Academy of Sciences. 2005; 102 (6): 2063–8.
- Wagner M, et al. CAR and PXR agonists stimulate hepatic bile acid and bilirubin detoxification and elimination pathways in mice. Hepatology. 2005; 42 (2): 420–30.
- Makishima M. Nuclear receptors as targets for drug development: regulation of cholesterol and bile acid metabolism by nuclear receptors. Journal of pharmacological sciences. 2005; 97 (2): 177–83.
- Boyer JL. Nuclear receptor ligands: rational and effective therapy for chronic cholestatic liver disease? Gastroenterology. 2005; 129 (2): 735–40.
- Keppler D. The roles of MRP2, MRP3, OATP1B1, and OATP1B3 in conjugated hyperbilirubinemia. Drug Metabolism and Disposition. 2014; 42 (4): 561–5.
- Schroeder RJ, et al. Cholesterol and sphingolipid enhance the Triton X-100 insolubility of glycosylphosphatidylinositol-anchored proteins by promoting the formation of detergent-insoluble ordered membrane domains. Journal of Biological Chemistry. 1998; 273 (2): 1150–7.
- Guyot C, Stieger B. Interaction of bile salts with rat canalicular membrane vesicles: evidence for bile salt resistant microdomains. Journal of hepatology. 2011; 55 (6): 1368–76.
- Linton KJ. Lipid flopping in the liver. Biochemical Society Transactions. 2015; 43 (5): 1003–10.
- Groen A, et al. Complementary functions of the flippase ATP8B1 and the floppase ABCB4 in maintaining canalicular membrane integrity. Gastroenterology. 2011; 141 (5): 1927–37.
- Wang H, et al. Endogenous bile acids are ligands for the nuclear receptor FXR/BAR. Molecular cell. 1999; 3 (5): 543–53.
- Parks DJ, et al. Bile acids: natural ligands for an orphan nuclear receptor. Science. 1999; 284 (5418): 1365–8.
- Makishima M, et al. Identification of a nuclear receptor for bile acids. Science. 1999; 284 (5418): 1362–5.
- Modica S, Gadaleta RM, Moschetta A. Deciphering the nuclear bile acid receptor FXR paradigm. Nuclear receptor signaling. 2010; 8 (1): nrs. 08005. Available from: https://journals.sagepub. com/doi/pdf/10.1621/nrs.08005.
- Mezina A, et al. 845 Whole Exome Sequencing Identifies ABCB4 Gene Variants As Modifiers of Biliary Atresia Outcomes. Gastroenterology. 2014; 146 (5): S-928.
- Ramachandran P, et al. RNA-seq reveals outcome-specific gene expression of MMP7 and PCK1 in biliary atresia. Molecular biology reports. 2019; 46 (5): 5123–30.
- Matthews RP, et al. DNA hypomethylation causes bile duct defects in zebrafish and is a distinguishing feature of infantile biliary atresia. Hepatology. 2011; 53 (3): 905–14.
- Dong R, Zhao R, Zheng S. Changes in epigenetic regulation of CD4+ T lymphocytesin biliary atresia. Pediatric research. 2011; 70 (6): 555–9.
- Dong R, et al. Abnormal DNA methylation of ITGAL (CD11a) in CD4+ T cells from infants with biliary atresia. Biochemical and biophysical research communications. 2012; 417 (3): 986–90.
- Udomsinprasert W, et al. Global methylation, oxidative stress, and relative telomere length in biliary atresia patients. Scientific reports. 2016; 6: 26969.
- Li K, et al. Foxp3 promoter methylation impairs suppressive function of regulatory T cells in biliary atresia. American Journal of Physiology-Gastrointestinal and Liver Physiology. 2016; 311 (6): G989–G997.
- Cofer ZC, et al. Methylation microarray studies highlight PDGFA expression as a factor in biliary atresia. PloS one. 2016; 11 (3): e0151521.
- Liu F, et al. PDGFA gene rs9690350 polymorphism increases biliary atresia risk in Chinese children. Bioscience reports. 202; 40 (7). Available from: https://portlandpress.com/bioscirep/ article/40/7/BSR20200068/225782/PDGFA-gene-rs9690350- polymorphism-increases.
- Yang Y, et al. MicroRNA-29b/142-5p contribute to the pathogenesis of biliary atresia by regulating the IFN-γ gene. Cell death & disease. 2018; 9 (5): 1–9.
- Ye Y, et al. Downregulation of microRNA-145 may contribute to liver fibrosis in biliary atresia by targeting ADD3. PloS one. 2017; 12 (9): e0180896.