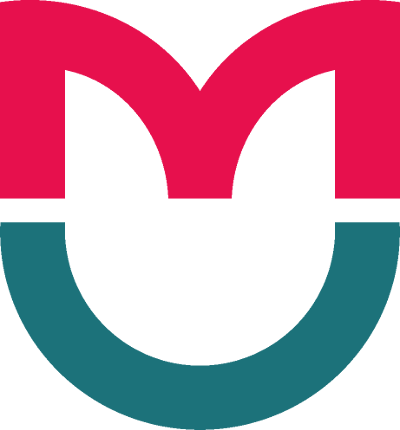
ORIGINAL RESEARCH
Changes in plasma sphingolipid levels against the background of lipid-lowering therapy in patients with premature atherosclerosis
1 Central State Medical Academy of the Department of Presidential Affairs of the Russian Federation, Moscow, Russia
2 City Clinical Hospital № 51, Moscow, Russia
3 Institute of Biochemical Physics named after N. M. Emanuel, Moscow, Russia
4 Lomonosov Moscow State University, Moscow, Russia
Correspondence should be addressed: Larisa O. Minushkina
Marshala Timoshenko, 19, str. 1А, Moscow, 121359; ur.liam@anikhsunim
Funding: RFBR grant 19-04-00870А, Sphingolipidome Analysis of Cardiovascular Disease Markers.
Author contribution: Rogozhina AA — sampling, data acquisition; Alessenko AV — project management; Kurochkin IN — data analysis; Minushkina LO — data analysis, manuscript writing; Gutner UA, Shupik MA, Maloshitskaya OA — sample preparation, laboratory tests, data analysis; Lebedev AT, Zateyshchikov DA — study planning, data analysis, manuscript editing.
Compliance with ethical standards: the study was approved by the Ethics Committee of City Clinical Hospital № 51, Moscow (protocol № 02/19 dated February 7, 2019). Informed consent was submitted by all patients.
In connection with increasing disability and mortality due to coronary artery disease (CAD) among young people [1], as well as with emergence of novel diagnostic methods, the in-depth investigation of risk factors, prevention methods, and complications of premature CAD (i. e. with the onset before the age of 55 years in men, and before the age of 60 years in women) [2], becomes more and more urgent.
The most significant risk factor for atherosclerosis is dyslipidemia, in particular, the elevated levels of lowdensity lipoproteins (LDL-C). These are directly associated with increased risk of cardiovascular disease [3]; hypercholesterolemia exceeding 4.9 mmol/L increases the risk of CAD by six times [4].
Due to developing mass spectrometry methods, the new laboratory parameters have been increasingly determined as additional markers of cardiovascular risk in patients with CAD, among them sphingolipids.
Sphingolipids contain the molecules of organic aliphatic amino alcohol sphingosine. It has been found, that sphingolipid catabolism is associated with cholesterol catabolism [5]. Cholesterol content of the membranes strongly correlates with the sphingolipid content (ceramides, sphingosines, and sphingosine-1-phosphate). Sphingomyelins form the myelin sheath cell membranes, and are detected in the atherosclerotic plagues [6]; ceramides contribute to cell proliferation, apoptosis and inflammation associated with atherosclerosis [7]. Currently, there is some evidence that ceramide levels, especially the levels of Cer16:0, correlate with the risk of atherosclerosis complications. These data served as the basis for calculation of the specific ceramide risk score (CRS) [8].
All the patients at high and extremely high risk for atherosclerosis have indications for high-intensity lipid-lowering therapy aimed to correct dyslipidemia and to reduce the risk of atherosclerosis progression.
The study was aimed to assess the dynamic changes in standard lipid indicators and sphingolipids against the background of lipid-lowering therapy in patients with premature CAD, atherosclerosis and hypercholesterolemia.
METHODS
The study was carried out in 2019–2020 at Moscow City Clinical Hospital № 51. A total of 18 patients (4 women and 14 men) aged 53 ± 6.7 (35–65 years) eligible for intensive lipid-lowering therapy were enrolled. Inclusion criteria: clinical manifestations of CAD or peripheral artery disease in men aged under 55, and women aged under 60, or hyperlipidemia (LDL-C levels exceeding 4.9 mmol/L). Exclusion criteria: no submitted informed consent. Fifteen patients had a history of acute myocardial infarction and coronary artery stent placement: two of them had multivessel disease and underwent coronary artery bypass grafting (CABG), others had peripheral artery disease or dyslipidemia. Among patients, 16 individuals (36.4%) had hypertension, three individuals (6.8%) had diabetes mellitus, 11 individuals (25%) reported smoking at the time of enrollment, and four individuals (9.1%) reported the history of smoking.
All patients were divided into three groups based on the fact of receiving lipid-lowering therapy at the time of enrollment (see figure). Dynamic changes in blood lipid levels were assessed prior to therapy and 4–8 weeks after starting treatment. The efficiency of initial statin therapy was analyzed in group 1 (n = 6). All patients in this group have had received no lipid-lowering medications prior to enrollment: at least three months prior to enrollment because of their own decision to discontinue treatment.
All patients in group 2 (n = 6) had a history of myocardial infarction in young age (the average age was 54.5 ± 1.87 years). At the time of enrollment, the patients received lipidlowering drugs prescribed by attending physicians outside the scope of the study, however, LDL-C target levels (<1.4 mmol/L) had not been achieved. Five people received 20–40 mg doses of atorvastatin, and one person received 10 doses of rosuvastatin. Lipid-lowering therapy was adjusted, and the dose was escalated in all patients.
All patients receiving statins were analyzed separately (groups 1 and 2) (n =12).
Group 3 (n = 7) included five men and two women (the average age was 52.29 ± 9.1 years) (one patient moved to group 3 after having completed the assessment in group 2 in accordance with the protocol). Five patients had a history of myocardial infarction. All of them received lipid-lowering drugs (atorvastatin 40–80 mg once daily, rosuvastatin 40 mg once daily in combination with ezetimibe 10 mg once daily or, in case of statin intolerance or contraindications, only ezetimibe 10 mg once daily). Against the background of such therapy, all patients had LDL-C levels exceeding 1.7 mmol/L at the time of enrollment. All patients were prescribed alirocumab 150 mg once daily for two weeks in accordance with the study protocol. Group 3 also included two patients with provisional or established diagnosis of familial hypercholesterolemia made in accordance with the Dutch Lipid Clinical Network Score. One of these patients could not take statins due to concomitant diseases of the hepatobiliary tree (chronic pancreatitis, hepatitis С) and elevated levels of transaminases. The second patient with stable CAD failed to achieve LDL-C target levels when receiving maximum tolerated statin doses.
Blood sampling for biochemical tests and mass spectrometry was performed on the day of enrollment (in the morning after 12-hour fasting) and after 4–8 weeks of therapy. Blood was collected from cubital vein into sterile Vacutainer tubes. Serum was obtained by blood centrifugation at 3000 rpm for 15 min. CLIMA MC-15 biochemistry analyzer (RAL; Spain) was used to determine the parameters of serum. The following reference values were used: total cholesterol 2.0–5.2 mmol/L; LDL-C up to 3.3 mmol/L; HDL-C 0.91–1.56 mmol/L; serum triglycerides 0.50–1.70 mmol/L.
Measuring plasma sphingolipid levels
Sphingolipids were determined in blood plasma of the described above patients. During sample preparation blood was centrifuged at 3000 rpm for 10 min, 1.5 ml of supernatant were collected. Then 1.5 ml of supernatant were centrifuged at 15,000 rpm for 10 min in order to ensure complete sedimentation of cells. Lipids for mass spectrometry were extracted from blood plasma by the Bligh and Dyer method [9].
Mass spectrometric detection of various molecular species of sphingomyelins, ceramides and sphingoid bases (sphingosine and sphinganine) was performed with the TSQ Endura Triple Quadrupole Mass Spectrometer (Thermo Fisher Scientific; Germany) using the multiple reaction monitoring (MRM) technique, the pressure in the collision cell was 2.0 mTorr. Resolution of Q1 and Q3 was 1.2 Da. For ceramides, fragmentation of protonated and dehydrated parent molecules was performed at the energy of 20 eV down to ion with m/z 264.2 Da, the dwell time was 35 ms. For sphingomyelins, fragmentation of protonated parent molecules was performed at the energy of 20 eV down to ion with m/z 184.1 Da, the dwell time was 35 ms.
For sphingosine and sphingosine deuterated standard (d7, Avanti; USA), fragmentation of protonated parent molecules was performed at the energy of 12.5 eV down to ions with m/z 259.3 and 252.3 Da respectively, the dwell time was 35 ms.
For sphinganine, fragmentation of protonated parent molecule was performed at the energy of 12.5 eV down to ion with m/z 266.3 Da, the dwell time was 35 ms. The following parameters of the ionization source were used: heater temperature set to 300 °С, capillary temperature 340 °С, sheath gas flow rate 45 arb (arbitrary units), auxiliary gas flow rate 13 arb, sweep gas flow rate 1 arb. Sphingosine d7, sphinganine, sphingomyelin d18:1/16:0, sphingomyelin d18:1/18:0, ceramide d18:1/16:0, ceramide d18:1/18:1, ceramide d18:1/18:0, ceramide d18:1/24:1, ceramide d18:1/24:0 (Avanti; USA) were used as standards. Chromatographic separation was carried out using the Ultimate 3000 system (Thermo Fisher Scientific; Germany) and the Eclipse Plus C8 column 3.0 × 150 mm (Agilent; USA), the particle size was 3.5 µm. Temperature was set to 50 °С, and flow rate was set to 400 µL/min. When determining sphingosine, ceramides and sphingomyelin, the following mobile phases were used: phase А, 0.1% formic acid (v/v) in water, phase B, 0.1% formic acid (v/v) in methanol (55% of phase B at 0.7 min, 100% of phase B at 7 min, 100% of phase B at 12 min, 55% of phase B from13 to 17 min, 55% of phase B at 13 min). When determining sphinganine, the following mobile phases were used: phase А, 0.1% formic acid (v/v) in water, phase B, 50% methanol + 50% acetonitrile + 0.1% formic acid (v/v) (20% of phase B at 1.5 min, 100% of phase B at 2 min, 100% of phase B at 7 min, 20% of phase B at 7 min, 20 of phase B at 10 min). The relative ceramide value was assessed using external calibration (method of standard addition). The Ceramide Porcine Brain 860052P ceramide mixture (Avanti; USA) containing 50% of d18:1/18:0 50% and 20% of d18:1/24:1 was used as a standard. Calculations were performed based on the peak areas for MRM transitions MH+·→ m/z 264.4 Da and (М+Н-Н2О)+·→ m/z 264.4 Da. The content of sphingosine d18:1 was determined by internal calibration (internal standard method, the standard was D-erythro-sphingosine d7, Sigma; USA) based on the sum of peak areas for MRM transitions (m/z 300+·→ m/z 252.3 Da for non-deuterated and m/z 307+·→ m/z 259.3 for deuterated sphingosine). The content of sphingosine d18:0 was defined using external calibration (the standard was DL-erythro-dihidrosphingosine, Sigma; USA) based on the peak areas for MRM transitions m/z 302+·→ m/z 266.3 Da.
CRS, described as a predictor for coronary artery disease mortality, was assessed using the previously reported scale involving the use of certain ceramide molecular species [8]. In order to access the ceramide risk, the following ratios were calculated: Cer16:0/Cer24:0, Cer18:0/Cer24:0, Cer24:1/ Cer24:0. The levels of Cer16:0, Cer18:0, Cer24:1, and the values of these ceramides’ ratios with Cer24:0, were divided into quartiles. The patients falling within the third quartile were assigned score 1 for each of six parameters, and the patients falling within the fourth quartile were assigned score 2. The total score was considered the ceramide risk score.
Statistical analysis
Statistical processing of the results was performed using the SPSS ver. 21.0 software package (IBM; USA). Quantitative variables were presented as mean and standard deviation (M ± SD). All quantitative variables were tested for normality using the Shapiro–Wilk test. Distribution of all the quantitative variables was other than normal. The Wilcoxon signed-rank test was used to compare related samples. Significance of differences between two independent samples was determined using the Mann–Whitney test. Significance of correlations was assessed using the Spearman’s rank correlation coefficient. The differences were considered significant when р < 0.05 in all types of analysis.
RESULTS
Changes in cholesterol and sphingolipid levels were assessed by groups of patients at the time of lipid-lowering therapy initiation and adjustment (statins or alirocumab) (tab. 1).
In group 1, a significant decrease in the levels of shortchain ceramides Cer 14:1 was observed (p = 0.046). Longchain sphingomyelins SM 22:1, SM 22:0, SM 24:0 significantly decreased against the background of lipid-lowering therapy initiation in all patients of this group (p = 0.028).
In group 2, the levels of total cholesterol (p = 0.028), LDL-C (p = 0.043), long-chain sphingomyelins SM18:1, SM 24:1 and SM 26:1, ceramide Cer 16:1 (p = 0.028), and SM 22:1 (p = 0.046) significantly decreased against the background of the escalated statin doses. Cer 22:1 levels significantly increased (p = 0.028).
After the lipid-lowering therapy adjustment, the levels of cholesterol decreased by 9.5% (Δ cholesterol = –0.45 ± 0.40), and the levels of LDL-C decreased by 16.2% (ΔLDL-C = –0.50 ± 0.44) in all patients. LDL-C target levels were achieved in only one patient, in other patients, advice was provided on lifestyle correction, the lipid-lowering drug dose was escalated, or the second drug was prescribed (ezetimibe 10 mg once daily). One female patient maintained LDL-C levels ≥ 2.6 mmol/L against the background of dual lipid-lowering therapy (maximum tolerated doses of statins: atorvastatin 80 mg, ezetimibe 10 mg). She was prescribed alirocumab.
Analysis of data obtained in all patients receiving statins revealed significantly decreased levels of cholesterol (p = 0.028), LDL-C (p = 0.021), and long-chain sphingomyelins SM 24:1 (p = 0.012), SM 24:0 (p = 0.028), SM 26:1 (p = 0.010), SM 22:1 (p = 0.004).
In group 3, the levels of total cholesterol decreased by 36.2% (ΔTC = –2.72 ± 4.48), and the levels of LDL-C (p = 0.018) decreased by 60.1% compared to baseline (ΔLDL-C = –2.67 ± 3.12) against the background of alirocumab therapy.
Of all the defined ceramide ratios (Cer 16:0/24:0, 18:0/24:0, 24:1/24:0), only the Cer 16:0/24:0 ratio significantly decreased in the group receiving alirocumab (p = 0.043). No significant changes in ceramide risk score were observed in any of the groups.
Comparison of dynamic changes in sphingolipid levels was performed in patients, who had started therapy for the first time, and against the background of escalated statin doses (groups 1 and 2), as well as in each group of patients receiving alirocumab (group 3) (tab. 2).
When comparing dynamic changes in sphingolipid levels in each group, it was observed that in group 1 the level of ceramide Cer 16:1 increased slightly (Δ Cer 16:1 = 4.1 ± 7.17), and in group 2 it decreased (Δ Cer 16:1 = –1.70 ± 1.32). The differences were considered significant (p = 0.015).
Against the background of alirocumab therapy, the increase in the levels of Cer 14:1 (Δ Cer 14:1 = 0.10 ± 0.35) was observed (p = 0.022), however, the levels of this ceramide decreased (Δ Cer 14:1 = –0.26 ± 0.259) in patients, who had started taking statins. When comparing dynamic changes in groups 1 and 3, the significant differences were observed in the levels of SM 22:0 (Δ SM 22:0 = –10310.6 vs 8114.48) (p = 0.035) and SM 24:0 (Δ SM 24:0 = –11342.31 vs 4807.69) (p = 0.022). In patients receiving statins and patients receiving alirocumab the dynamic changes of SM 22:1 (Δ SM 22:1 = –4793.04 vs 3930.79) (p = 0.045) and SM 26:1 (ΔSM 26:1 = –207.00 vs 150.46) (p = 0.005) were different in groups 2 and 3 (ΔSM 26:1= –191.58 vs 150.46).
When conducting correlation analysis in group 1, a significant strong positive correlation was observed between the dynamic changes of Cer 14:1 and the changes in the levels of LDL-C (ρ = 0.829; p = 0.042); there was а significant strong negative correlation with baseline LDL-C level (ρ = –0.829; p = 0.042) (tab. 3). In the same group, a positive correlation was observed between the dynamic changes of long-chain sphingomyelins, and the changes in the levels of LDL — Δ SM 24:1 with Δ LDL (ρ = 0.943; p = 0.005), Δ SM 26:1 with Δ LDL (ρ = 0.829; p = 0.042), together with negative correlation with baseline LDL-C level — with Δ SM 24:1 (ρ = –0.943; p = 0.005), with Δ SM 26:1 (ρ = –0.829; p = 0.042). In group 2, only changes in SM 18:0 positively correlated with baseline LDL-C level (ρ = 0.812; p = 0.05).
In group 1, there was a significant strong correlation between the dynamic changes in Δ SM 26:1 and the baseline HDL-C level (ρ = –0.829; p = 0.042); no significant correlations were observed in patients of group 2. Significant correlations with baseline HDL-C level in all patients receiving statins were observed for Δ SM 14:1 (ρ = –0.602; p = 0.038), Δ SM 14:0 (ρ = –0.676; p = 0.016), Δ SM 16:1 (ρ = –0.630; p = 0.028), Δ SM 24:0 (ρ = –0.581; p = 0.047, Δ SM 26:1(ρ = –0.595; p = 0.041). In group 3, there was a significant strong negative correlation between changes in SM 20:1 (ρ = –0.929; p = 0.003) and SM 20:0 (ρ = –0.929; p = 0.003) and baseline HDL-C level.
DISCUSSION
urrently, the major classes of lipid-lowering drugs are inhibitors of HMG-CoA reductase (statins) inhibiting hepatic cholesterol biosynthesis, cholesterol absorption inhibitors (ezetimibe), and proprotein convertase subtilisin/kexin type 9 (PCSK9) inhibitors acting through inhibiting of PCSK9, binding to LDL receptor, and the increase in the number of such receptors on the surface of hepatocytes. When applying lipid-lowering therapy, the main clinical reference is the decrease in the LDL-C levels to target levels [2]. The differences in the mechanism of action may also contribute to differences in effect on the levels of other lipid fractions, such as sphingomyelins and ceramides. It is known, that ceramide levels may be considered as an additional risk factor for complications of atherosclerosis (myocardial infarction and stroke). This has been shown in the cohort study Rochester Epidemiology Project (REP) when observing 1131 healthy individuals aged over 45. The ratios Cer 16:0/Cer 24:0, Cer 18:0/Cer 24:0, Cer 24:1/Cer 24:0, and the ceramide risk score were the main predictors of adverse outcomes [10]. The same ceramides and their ratios were found to be independent predictors of cardiovascular death in the cohort of 1704 patients with CAD [11]. In the course of our study, we also assessed the dynamic changes of these ratios and the ceramide risk score against the background of lipid-lowering therapy.
Elevated sphingomyelin levels can be also considered as an independent predictor of adverse outcomes. This has been shown in analysis of cohorts of patients with familial lipid disorders using the methods of big data analysis and machine learning [12]. Prognostic value of certain sphingomyelins has been also shown for cerebrovascular disease [13].
There is little literature data on the effect of therapy with statins, PCSK9 inhibitors, and ezetimibe on the sphingolipid levels. The randomized trial showed that the combination of atorvastatin and fibrates ensured reduced levels of acylglycerols and the majority of ceramides, as well as the elevated levels of sphingomyelins. Atorvastatin dose escalation modestly decreased the levels of lysophosphatidylcholine [14]. The randomized trial aimed to compare the effect of 80 mg simvastatin and 10 mg simvastatin/ezetimibe combination showed the differences in the effects of these regimens on the levels of lipid and sphingolipid fractions. High-dose statin therapy resulted in more prominent changes in sphingolipid levels. Meanwhile, the dynamic changes in the cholesterol and LDL-C levels were comparable. The high-dose statin reduced the levels of sphingomyelins and ceramides much more; it also affected the levels of phosphotidylcholine, which could partially explain the pleiotropic effect of statins [15].
In the course of randomized trial involving the use of different rosuvastatin doses (10 and 40 mg) in patients with metablic syndrome, both low and high statin doses significantly decreased the levels of ceramides and sphingolipids. The significant dose-dependent differences were shown for dynamic changes of phosphatidylcholine, lysophosphatidylcholine,
alkylphosphatidylcholine, alkenylphosphatidylcholine (plasmalogen), and phosphatidylinositol. The dose-dependent effect was also shown for sphingolipids after being normalized to phosphatidylcholine level [16].
During treatment with rosuvastatin, the dynamic changes in blood ceramide levels negatively correlated with the dynamic changes in the levels of ApoB100 very low-density lipoproteins (VLDL). No association between the dynamic changes in the levels of ceramides and sphingomyelins, and the dynamic changes in the levels of total cholesterol, LDL-C, triglycerides, and АроА1 LDL was observed. The discovered association between the dynamic changes in АроВ VLDL and the dynamic changes in ceramides against the background of rosuvastatin therapy demonstrates the potential features of the mechanism of action for this statin [17].
In our study, the initial treatment with statins resulted in significantly decreased levels of Cer 14:1 and reduced levels of sphingomyelins SM 22:1, SM 22:0, and SM 24:0. Reduced levels of Cer 14:1 and long-chain sphingomyelins correlated with baseline levels of LDL-C and dynamic changes in LDL. With the lipid-lowering treatment intensification (escalation of statin dose and addition of ezetimibe in some patients), the overall dynamic changes in sphingomyelins were more prominent, which correlated with the results of the studies cited. No significant impact on the levels of ceramides and ceramide risk was observed.
Association between PCSK9 level and blood ceramide and sphingolopid levels was investigated in the course of the study, involoving 31 patients with liver diseases of different etiology. In patients with high PCSK9 level, LDL-C levels were higher than expected. However, the levels of total cholesterol, sphingolopids, and ceramides showed no correlation with PCSK9 level. After the patients were divided into groups with high and low PCSK9 levels, it turned out that the patients with plasma PCSK9 levels above the median had significantly lower levels of certain sphingolipids and ceramides: CE 16:0, CE 20:5, CE 20:4, CE 22:6, CE 22:4, SM 18:0, SM 20:1, SM 24:2, SM 24:1. To date, the mechanism, underlying such association, is unclear [18].
The EQUATOR trial, involving the use of monoclonal antibody against PCSK9 RG7652, showed the predominant decline in the levels of ceramides CE 24:1, CE 24:0, and СE 26:0, contained mainly in LDL [19]. The other study, involving PCSK9 inhibitor prescription against the background of treatment at the maximum tolerated statin dose, also showed both reduced ceramide levels and reduced ceramide risk score, the integral parameter, which correlated with the risk of adverse outcome in patients with dyslipidemia [20]. Earlier, the Ludwigshafen Risk and Cardiovascular Health (LURIC) trial showed that homozygous state for the rare mutant allele R46L (PCSK9), characterized by low enzyme activity, was associated with 30% decrease in the ceramide risk score compared to other allelic variants [21]. This trial also demonstrated 13% decrease in the ceramide risk score against the background of simvastatin therapy, and lack of ceramide dynamic changes against the background of taking ezetimibe.
During our study, alirocumab added to therapy resulted in significantly decreased LDL-C, however, no significant changes in the levels of sphingomyelins and ceramides were observed. Only significant increase in Cer 22:1 and decreased ratio Cer 16:0/24:0, being the marker of ceramide risk, were observed. In the course of our study, alirocumab was added to therapy in patients, who had previously received maximum tolerated statin doses in accordance with indications registered for appropriate drug [2, 22]. However, the baseline sphingolipid levels were relatively low; these were significantly lower compared to groups 1 and 2 against the background of four-week statin therapy. Against the background of low baseline sphingomyelin levels, PCSK9 inhibitor had no significant effect of the levels of sphingomyelins and ceramides. It was probably due to the drug mechanism of action, different from the statin mechanism of action.
This study had the following limitations: the sample of patients was small, the study was conducted with the use of background therapy with various statins.
CONCLUSION
Sphingomyelin levels decrease against the background of statin therapy; when applying starting doses of statins, these correlate with reduced levels of LDL-C. When escalating lipidlowering therapy, the correlation between dynamic changes in sphingomyelins and LDL becomes less prominent. Treatment with PCSK9 inhibitors results in significantly reduced LDL-C levels, however, it does not affect the levels of sphingomyelins. No significant dynamic changes in ceramides and ceramide risk are observed against the background of statin therapy. However, PCSK9 inhibitor added to therapy reduces the Cer 16:0/24:0 ratio, which can be considered a marker of decreased cardiovascular risk.