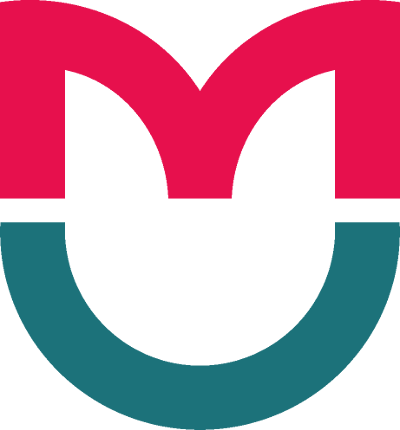
This article is an open access article distributed under the terms and conditions of the Creative Commons Attribution license (CC BY).
REVIEW
Recent advances in diagnostics of neonatal hypoxic ischemic encephalopathy
Kulakov National Medical Research Center for Obstetrics, Gynecology and Perinatology, Moscow, Russia
Correspondence should be addressed: Denis N. Silachev
Akademika Oparina, 4, Moscow, 117997, Russia; ur.4anirapo@vehcalis_d
Funding: the study was supported by the Russian Science Foundation grant number 22-15-00454; https://rscf.ru/project/22-15-00454/
Author contribution: Starodubtseva NL, Eldarov ChM, Kirtbaya AR, Balashova EN, Gryzunova AS, Ionov OV, Zubkov VV and Silachev DN — literature analysis, manuscript writing and editing.
Neonatal hypoxic ischemic encephalopathy
Neonatal hypoxic ischemic encephalopathy (HIE) is one of the leading causes of neonatal mortality and disability. Among premature babies (gestational age < 28 weeks) HIE occurs in about 4–48 cases per 1000 pregnancies, and the incidence strongly correlates with a decrease in gestational age [1–4]. HIE accounts for 6–9% of all neonatal deaths and 21–23% of term infant deaths. According to the literature, up to 10% of neonates with HIE die after birth and 25% develop severe neurological disorders: cerebral palsy, seizures, mental retardation, cognitive impairment and epilepsy [5–9].
Clinical outcomes in infants with this pathology depend on its correct prediction, detection and classification as a basis for the choice of strategy. Among infants with signs of perinatal asphyxia, about 20% are at risk for HIE of varying severity, of which 40–50% will be at risk of disability, including mental retardation, epileptic seizures, visual and hearing impairments and possibly death [10].
Therapeutic hypothermia (TH) is a proven, effective treatment for HIE that improves survival and long-term prognosis [11, 12]. Current recommendations for the use of TH include infants with moderate to severe HIE starting from 37 weeks of gestation and exclude infants with mild HIE. Still, 75% of neonatal centers in UK perform TH in infants with mild HIE, according to a national survey [13]. The prospective research on infants with mild encephalopathy (PRIME) project revealed early emergent electroencephalography (EEG) changes, seizures, pathological signs in brain MRI or neurological symptoms in 52% of infants with mild HIE at discharge [14]; a follow-up examination in 19 months on average revealed disability in 16% and Bayley-III scores below 85 in 40% of the cases. It was noted that in children with mild HIE receiving no TH, cognitive outcomes were similar to those in children with HIE of moderate severity and no TH. A systematic follow-up in infants with mild HIE revealed neurodevelopmental pathological sequelae in 25% of the cases (n = 341) [15–17].
Clinical diagnosis and prognosis of HIE
The early and accurate assessment of HIE severity remains one of the most challenging tasks in neonatal care. Neonatology uses a variety of clinical methods to assess the morphological and functional state of the nervous system in order to predict longterm outcomes, such as neuroimaging and neurophysiological techniques, and their combinations [18–22]. However, the currently available instrumental assessments for the risks of brain damage in neonates have inherent limitations that prevent using them during the first hours of life; hence the uncertainty about the severity of ongoing brain damage and concomitant neurological risks during the early postnatal period [23, 24].
The available clinical assessment tools for HIE include amplitude-integrated EEG, neuroimaging and cerebral blood flow scans [22, 24–27]. A number of studies have shown that about 80% of EEG seizures in neonates are unrelated to clinical status and thus cannot be identified without constant EEG monitoring, even by experienced clinicians. Moreover, there is no straightforward correlation between HIE severity and the incidence of seizure activity revealed clinically or by EEG [28–30].
The amplitude-integrated background EEG patterns in HIE strongly correlate with neurological outcome [31]. However, their prognostic value depends on the duration of background pattern changes with regard to HIE, whereas time limit for the prevention of adverse outcomes in infants receiving TH constitutes 48–72 h of life [27]. Qualitative assessment of brain damage by MRI during the first 48 hours of life is prone to underestimation [32–34].
Various risk factors have been shown to undermine the benefits of TH in newborns with asphyxia, including HIE severity, delayed commencement of the treatment, hypo- and hyperglycemia, seizures, hyperoxia and hypocapnia during the therapy, as well as certain comorbidities. A well-known risk factor for poor neurological outcome in neonates is perinatal sepsis; besides, TH has a negligible neuroprotective effect in the inflammation-sensitized hypoxic-ischemic encephalopathy [35–39].
HIE is rarely caused by metabolic imbalances [40]. Congenital metabolic disorders manifest in neonatal period after normal delivery with no signs of perinatal asphyxia. The newborns develop neurological symptoms and signs of multiple organ failure, which may clinically resemble HIE. A detailed family history to establish risk factors such as parental consanguinity or infant death in the anamnesis is essential, as most congenital metabolic disorders have an autosomal recessive pattern of inheritance. All neonates at risk for congenital metabolic disorders should be examined accordingly, as these patients may decisively benefit from specific therapies rather than hypothermia.
Another category of genetically determined neurological disorders mimicking HIE are neonatal myopathies and encephalopathies. Neonates with congenital centronuclear myopathy present with muscular hypotension associated with respiratory failure and early lethal outcome, which may also mimic HIE [41]. The widespread use of whole-genome sequencing methods has revealed a number of new pathological variants, e.g. in GNAO1, associated with functional defects of the central nervous system and clinical phenotypes similar to HIE [42, 43]. Thus, TH has been effective only in some patients with clinical signs of HIE. The early differential diagnosis of HIE in neonates can be facilitated by analysis of blood levels for a variety of metabolites using advanced analytical techniques, notably chromatography–mass spectrometry. This method allows identification of hundreds of metabolites within a few hours, which fits the time window for a decision on TH.
New approaches in HIE diagnostics: proteomic studies
Recent years have been marked by a constantly growing interest in systems biology — a science that integrates several levels of information to achieve a more comprehensive knowledge of a biological system. Genomics, transcriptomics, proteomics and metabolomics are the pillars of systems biology, forming a group of the so-called 'omics'-sciences. The ongoing boom in 'omics'-sciences is a consequence of recent breakthroughs in instrumental technologies, particularly in the fields of chromatography, mass spectrometry and sequencing. Technologies used to create the 'omics'-platforms are analytical approaches for obtaining information about molecules of various order that make up a biological sample: nucleic acids, proteins and metabolites. These methods allow simultaneous recording of quantitative and qualitative content for thousands of compounds, thus creating a unique 'fingerprint' of the current state of the body. The 'omics'-sciences are mutually complementing: genomics reveals the potential, transcriptomic shows the intention, proteomics tells what is going on and metabolomics reflects the influence of external and internal environments on the genetically determined biochemical landscape. As genes and proteins provide the basis for cellular activities, big shifts in physiological status mostly occur at the level of metabolites. In the applied perspective, metabolome is probably the best sensor for pathophysiological status of cells, tissues, organs and the body [44].
Research on biomarkers of various diseases, including those with high social impact, constitutes a booming field at the intersection of genomics, proteomics, metabolomics and instrumental analytics including nuclear magnetic resonance (NMR), mass spectrometry (MS), high performance liquid chromatography coupled with mass spectrometry (HPLC-MS/MS) and enzyme immunoassay (ELISA). This is reflected by a soaring number of publications, symposia and special sections at industry conferences focused on this issue.
It should be noted that opportunities of clinical investigation during the neonatal period are tightly limited. Among neonatal biological samples suitable for the study of molecular marker dynamics those collected non-invasively (urine, stools) or lowinvasively (blood, dried blood spots) are highly preferable for medical reasons. Leftovers from routine clinical tests can be used straightforwardly for advanced analysis without additional contacts with the patient.
Several studies featuring quests for biomarkers in neonatal blood, urine and cerebrospinal fluid (CSF) samples have been published, representing various pathologies including HIE [45]. It should be noted that CSF collection is highly invasive. True enough, CSF may contain neural markers including glial fibrillary acidic protein (GFAP) and S100B, but not during the first critical 6 h after birth, although these markers can be of significant value for the prognosis of already developed HIE [46]. The use of urine has been considered as well, notably for the measurements of lactate-creatinine ratio in suspected HIE; however, the infants with congenital neonatal asphyxia usually present with delayed onset of urination because of the concomitant kidney damage, which makes urine a hardly suitable substrate for clinical diagnosis of HIE on a routine basis [47].
Accordingly, the major efforts are focused on the use of biomarkers circulating with the blood, mostly of peptide nature, as well as neuronal exosomes and inflammatory cytokines and metabolites. The latest advances in metabolomics, proteomics and transcriptomics shift the focus towards complex molecular patterns instead of individual biological compounds. The much sought-for patterns should clearly distinguish HIE against the background of concomitant damage to kidney, myocardium, etc., inevitable in perinatal asphyxia. So far, the available 'omics' data for the initial stage of brain injury in HIE (hypoxic phase during the first 6 h after birth) have been clinically irrelevant.
The search for 'omics' patterns is complicated, firstly, by the nature of pathological process in HIE. The hypoxic conditions switch brain cells to anaerobic metabolism, to which they are not fit (especially neurons). Under conditions of stress, excitotoxicity and metabolic waste accumulation, the energy resources quickly become depleted and the cells die massively by necrosis thereby seeding the inflammatory reaction. The ischemic/hypoxic phase is followed by reperfusion/reoxygenation phase marked by reactive oxygen species production, initiation of apoptosis cascades and inflammation. The final and permanent phase of chronic inflammation aggravates the excitotoxicity, oxidative metabolism impairment and protease and caspase activation, eventually leading to organic brain injury and impaired functionalities [48]. The associated dynamic changes in molecular and biochemical cascades are mirrored by corresponding profiles in blood plasma; noteworthy, many relevant candidate markers (compounds with characteristic disease-related dynamics) 'miss' the time window of the emergency decision making (within 6 h after birth). For instance, blood levels of the above-mentioned GFAP and S100B, as well as neuron-specific enolase and myelin basic protein, rise 12–24 h after birth i.e. clearly beyond the 6 h limit [49–51]. Clinically, HIE is difficult to differentiate from neonatal sepsis or congenital metabolic disorders; moreover, sepsis may accompany HIE, modifying its clinical presentation. It is important to emphasize that erroneous administration of HT due to diagnostic inaccuracy can be harmful to patients with non-ischemic encephalopathies [52]. This additional constraint underscores the demand for reliable markers of neonatal brain damage associated specifically with the ischemic/hypoxic genesis of the condition.
Another obstacle in molecular diagnostics of HIE is marker specificity with regard to neuronal damage. By assumption, such markers should be mostly proteins essential for structural integrity of nervous tissue; accordingly, upon violation of the blood brain barrier such markers penetrate to CSF first and then to the blood [53]. Very often, though, the cues are blurred by collateral processes, e.g. inflammatory, triggered by asphyxia. For instance, in cord blood of full-term newborns with severe HIE (n = 25) the brain-type creatine phosphokinase (BT-CC) levels were increased several-fold compared with matching healthy controls over the first 6 h and 24 h of life [54]. Of note, BT-CC is not a brain damage-specific enzyme, but is expressed in most tissues and its elevated levels may result from damage to other organs in asphyxia [55]. Another candidate molecule, ubiquitin C-terminal hydrolase L1, is a neuron-specific cytoplasmic marker of apoptosis. Blood tests for this protein carried out in 50 newborns (37 weeks of gestation on average) revealed significant increase within 24 h of life specifically in moderate and severe HIE, despite a negligible correlation of its levels with subsequent MRI changes recorded on day 5 and in 1 year follow-up [56]. Yet another study focused on neurofilament light chain protein (NFL) showing increased blood levels in newborns with moderate and severe HIE receiving HT. The initial measurement was made at 18 h, when the temperature inside HT chamber reached its target level. Despite strong correlation of NFL blood dynamics with MRI scans revealed in the study, no data for the first 6 h of life were available, and apparently NFL should be regarded as a late prognostic marker non-applicable for the early diagnosis required for the emergency treatment [57].
The violation of the blood-brain barrier integrity promotes endothelial cell activation with induced secretion of non-specific angiogenic markers, notably VEGF. Apart from endothelial cells, VEGF can also be produced by astrocytes and microglia. However, the evidence on its increased plasma levels in HIE is contradictory [58] and the molecule is not specific for brain tissues [59]. A similar situation is observed for adrenomodulin and secretoneurin, produced by the brain but also by the endocrine and neuroendocrine systems, with the expression significantly enhanced by hypoxia [60, 61].
Inflammation markers can also have diagnostic significance, as different types of immune cells and inflammatory factors participate in primary and secondary reactions to ischemic damage, including regeneration. A number of cytokines (TNFα, interleukins IL1β, IL6, IL8, IL10, etc.) show significantly increased serum levels in both term and preterm babies with neonatal asphyxia [62]. In a study enrolling 20 newborns with asphyxia, 36 weeks of gestation on average, plasma levels of pro-inflammatory cytokines (notably IL6 and IL8) were significantly increased within 6–24 h of life and correlated with HIE severity [63]. At the same time, it should be remembered that hypoxiaischemia-associated inflammatory processes affect all systems of the body, especially in preterm babies. In fact, the inflammatory reaction can be triggered by the labor itself and subsequently amplified under conditions of HIE [64]. A study enrolling newborns at 35 weeks of gestation with HIE, receiving HT (33 °С for 72 h, commenced within 6 h of life) or not, revealed an important role of IL6, IL1β, IL8, TNFα and IL10 in HIE and correlation of their levels with the outcome; importantly, these levels cannot be attributed specifically to brain damage. In cases of successful HT, the IL6, IL8 and IL10 levels decreased significantly by 36 h of life [65]. Yet another study assessed the cytokine profiles in newborns (36 weeks of gestation) with pronounced HIE, receiving HT (33.5 °С for 72 h) starting from within 6 h of life. Blood samples collected 24 h and 72 h after birth revealed sustainably elevated cytokine levels for IL6 only [51].
Overall, the above-mentioned studies indicate low prognostic capacity and questionable feasibility of using individual molecular and biochemical markers for clinical purposes in HIE. Hopefully, the use of 'omics' technologies to reveal complex disease-specific patterns of biomolecules will be more helpful; in this regard mass spectrometry holds great promise as a universal tool for highthroughput analysis of various biological compounds in one sample, including proteins, lipids and metabolites.
Studies considering proteomic analysis of biological fluids collected from neonates with HIE are still rare [66, 67]. One of them (2020) enrolled newborns with HIE (gestational age 37 weeks; n = 12 including four pts of each grade — mild, moderate and severe) and matching controls (n = 16). Blood samples (3 mL) were collected before HT, within 5–7 h of life. The blood was tested by quantitative mass spectrometry using an isobaric label for relative quantification (iTRAQ). Subsequent bioinformatic analysis showed that blood levels of certain proteins differed between HIE patients and controls in a severitydependent manner; a set of 133 unique candidate proteins were identified, 14 of them showing elevated blood levels. Most of the differentially represented proteins were involved in cell damage responses or acute/chronic inflammation, or incorporated in the membranes of extracellular organelles and exosomes. However, only two candidate markers, haptoglobin and S100A8, were subsequently confirmed by Western blot and PCR as differentially represented. The S100A8 protein is involved in several important pathways, including inflammation and the regulation of calcium homeostasis, through interactions with S100A9 [68]. The S100A8/A9 complex inhibits pro-inflammatory cytokine production thus suppressing excessive inflammation [69], whereas haptoglobin is an antioxidant protein which binds free hemoglobin thereby protecting the brain from reactive oxygen species upon hemorrhage; haptoglobin is also expressed in the liver [70]. So far, this is the only published proteomic analysis of blood samples collected from neonates with HIE [67].
Yet another published attempt of proteomic/peptidomic analysis (2020) involved CSF samples collected from newborns with HIE (n = 4; 38–39 weeks of gestation at birth, Apgar score < 5) within 24 h of. The iTRAQ mass spectrometry revealed differential representation of 34 peptides belonging to a total of 25 proteins compared with the control. Bioinformatic analysis implicated many of these proteins in chromatin compaction processes within the nucleus. The most prominent reduction was shown by a heat shock protein 90α peptide involved in cell death program through formation of pores in the membrane, as confirmed by functional in vitro assay. The experiments on PC12 cell line demonstrated that the studied heat shock protein 90α peptide indeed protects the cells from pyroptosis induced by a 6 h glucose-oxygen deprivation [66]. These results illustrate the utility of animal and cell experimental models in the search for brain damage-associated specific patterns for clinical translation. The approach enables differentiation of the clinical picture of brain tissue damage from other complications and dysfunctionalities associated with asphyxia.
Experimental models for brain damage marker identification
In vitro and in vivo experimental models help to identify specific proteomic and metabolomic patterns in HIE by means of reducing the system's complexity. The newly identified molecular patterns of brain damage can be used in clinical data interpretation and analysis. One study modeled hypoxic ischemia in mice on postnatal day 7; the experiments involved ligation of carotid artery and 92% N2 / 8% O2 chamber for 30 min, followed by mass spectrometry analysis of brain tissue 24 h post-intervention. The results indicate significantly reduced expression of collapsin response mediator proteins known to be involved in axon pathfinding and neuronal outgrowth in general [71]. Another team conducted metabolomics studies in newborn pigs. The animals underwent ligation of the left jugular vein and right carotid artery and were placed under hypoxic conditions to model asphyxia (12–36 h of life). Blood samples collected at three time points (before the procedure, immediately after hypoxia and 120 min after hypoxia during the reoxygenation phase) were analyzed by liquid chromatography–time-of-flight mass spectrometry. The results indicate substantial increase in biochemical markers of anaerobic metabolic pathways including cytidine and uridine derivatives, free fatty acids and choline. The authors suggest using these compounds as early diagnostic markers of HIE in neonates [72]. Several published in vitro studies used cell cultures as model systems to identify candidate markers of brain cell damage. In one study, murine hippocampal cell line HT22 underwent 17.5 h of hypoxia in the presence of 0.69 mM glucose. Proteomic analysis by HPLC–tandem mass spectrometry revealed a set of 105 unique proteins expressed differentially by treated cells compared with the control (normoxia).
As shown by subsequent processing, the majority of these proteins were targets of HIF-1α induced by hypoxia; although the factor per se escaped proteomic detection, its increased expression was revealed by western blot analysis. The induction of HIF-1α targets triggered glycolysis and antioxidant systems while blocking inflammatory reactions [73]. Another study modeled the acidosisinduced cell damage in neuronal cultures by 24 h incubation at pH = 6.2. Subsequent proteomic analysis revealed a set of 69 unique proteins related to cell death, impaired synaptic plasticity and oxidative stress [74]. Such studies demonstrate the suitability of cell models for identification of specific proteomic markers of neuronal damage for the purposes of differential diagnostics.
Metabolomic approaches in HIE diagnosis and prognosis
Metabolomic profiling of the blood can be a highly sensitive diagnostic and prognostic tool for neonatal conditions including congenital metabolic disorders, sepsis and HIE. Neonatal sepsis is invariably accompanied by changes in glucose and lactate metabolism, reflecting a shift from the mitochondrial oxidative phosphorylation to glycolysis and pentose phosphate pathway, as well as oxidative stress and fatty acid oxidation [75]. Several candidate metabolites have been also proposed as markers for the early diagnosis of HIE.
It should be emphasized that HIE severity and dynamics depend on gestational age at birth. Accordingly, metabolomic and proteomic profiles of the disease are likely to be gestational agedependent both qualitatively and quantitatively [76, 77]. In term and preterm babies, metabolomic profiles behave differently. For instance, markers of tyrosine metabolism, tryptophan/phenylalanine biosynthesis, urea cycle and arginine/proline metabolism in the urine significantly correlate with gestational age [78].
Contemporary advances in MS and NMR have enabled metabolomics analysis for HIE with simultaneous recording of spectra for hundreds of metabolites, which is an absolute prerequisite given the dynamic character and complexity of the pathogenic process in HIE. In accordance with the nature of pathology, the metabolomic tests are focused on the signs of hypoxia and metabolic impairments. The strategies can be roughly defined as targeted (i.e. analysis of a small number of particular metabolites), semi-targeted (analysis of a large panel of metabolites), and non-targeted (whole-metabolome). About a dozen of studies describing urine and blood metabolome dynamics in neonates with HIE have been published so far; the urine samples were studied by MS and NMR.
One study (2018) analyzed urine samples from newborns with HIE (n = 10; 38 weeks of gestation at birth, Apgar score 5–7). The urine was collected 6 h, 48 h (during HT), 72 h (after HT) and 1 month after birth. A NMR study revealed significantly increased urine levels of lactate, myoinositol and betaine along with significantly decreased urine levels of the Krebs cycle intermediates (citrate, α-ketoglutarate, succinate), acetone, dimethylamine, glutamine, pyruvate, N-acetyl groups, arginine and acetate in lethal cases compared with matching healthy controls [79]. Another study (2017) used HPLC-MS/MS for metabolomic analysis of urine samples from newborns with HIE (n = 13; 36 weeks of gestation at birth on average, Apgar score 2–3). The urine was collected 12 h and on days 3 and 9 after birth. The results indicate significantly decreased levels of certain amino acids (the branched leucine and isoleucine and aromatic phenylalanine, tyrosine and tryptophan), kynurenine and hippuric acid along with increased acylcarnitine levels in HIE compared with matching healthy controls [80].
Metabolomic studies on neonatal blood samples collected from patients with HIE have been attempted as well. One study applied non-targeted metabolomic analysis of cord blood collected from newborns (gestational age 36 weeks, Apgar score 6) diagnosed with neonatal asphyxia with or without signs of HIE. The samples were collected within 20 min after delivery of the placenta during labor. The HIE and non-developed HIE subgroups revealed statistically significant differences in cord blood levels of melatonin, leucine, kynurenine and 3-hydroxydodecanoic acid. HIE severity markers were also identified, including D-erythrose phosphate, acetone, 3-oxotetradecanoic acid and methylglutaryl carnitine. Bioinformatic analysis has shown that HIE disrupts about 50 and 75% of tryptophan and pyrimidine metabolic pathways, respectively [81]. A recent study (2021) applied gas chromatography with time-of-flight mass spectrometry in a similar setting. The study enrolled newborns with moderate and severe HIE (n = 24; gestational age 35 weeks, Apgar score < 7) and matching healthy controls (n = 24). The samples (2 mL of blood from femoral artery) were collected immediately after birth in order to obtain plasma for metabolomic analysis. A set of 52 metabolites detected in the blood of newborns with HIE clearly differentiated the patients from the control group. These metabolites have been affiliated to pathways of amino acid metabolism, energy metabolism, neurotransmitter biosynthesis, pyrimidine metabolism, HIF regulation by oxygen and G proteincoupled receptor (GPCR) signaling. Fourteen compounds showed high predictive value for HIE, including alanine, glutamic acid, glutamine, L-malic acid, succinic acid, pyruvic acid and taurine. In addition, α-ketoglutaric acid and hydroxylamine revealed high predictive value between moderate and severe forms of HIE, with the area under ROC curve being 0.729 [82].
Metabolomic patterns revealed in these studies are consistent with the impaired energy metabolism and mitochondrial damage initiated in HIE. The most typically observed changes involved energy metabolites indicating a shift towards anaerobic metabolism (elevated lactate levels) and Krebs cycle disruption. The accumulation of lactate and Krebs cycle intermediates (citrate, alpha-keto-glutarate, succinate and fumarate) are explained by ATP depletion and respiratory chain dysfunction. In newborns with pronounced (moderate to severe) HIE, elevated succinate levels may also correlate with severity [83]. We should also note that a number of studies have repeatedly emphasized that even after 48 h of intensive care with HT, newborns with successfully treated HIE have altered metabolic features distinguishing them from healthy peers [84]. The altered glutamine levels reflect its being a precursor for glutamate, the main excitatory mediator during perinatal brain injury [85]. Hypoxanthine, which promotes formation of the detrimental reactive oxygen species upon interaction with xanthine oxidase, is often mentioned in such studies; a characteristic increase in hypoxanthine levels is considered the main clinical biochemical feature of asphyxia [86].
Metabolomic analysis revealed an important role of lipids in perinatal asphyxia. Choline revealed the most prominent HIE-related changes (elevated levels) among lipid metabolites [83]. Choline and its derivatives are essential for the structural integrity of cell membranes and signaling (phospholipids), neurotransmission (acetylcholine synthesis), lipid transport (lipoproteins) and methyl group metabolism (homocysteine reduction) [87]. Blood levels of inositol, the main precursor in the synthesis of phosphorylated signaling compounds, increase significantly in newborns after perinatal asphyxia, but these changes are not specific for nervous system damage [46, 77]. Overall, the search for markers of neuronal damage by metabolomic analysis can be assumed the most effective and appropriate vector for the field of early diagnosis in HIE.
Extracellular vesicles in HIE diagnostics
The targeted analysis of extracellular vesicles produced by neurons in HIE should be mentioned as a promising alternative. Extracellular vesicles (EVs) are specialized 'containers' of cellular origin consisting of cytoplasm enclosed in phospholipid membrane [88]. EVs have been shown to participate in a variety of biological processes including immune regulation, inflammation modulation, cell-to-cell communication, angiogenesis, etc. EVs released by neurons play important roles in their functional maintenance and development [89]. Neuronal EVs have been shown to increase in number under conditions of traumatic brain injury or stroke [90, 91]. EVs hold promise as diagnostic markers in many fields of medicine; their cell type-specific transmembrane proteins can be used as tags for EV sorting and subsequent analysis of particular classes. Neuronal EVs exhibit adhesion molecules, as well as receptors and ligands for specific recognition by target cells, and are probably involved in synaptogenesis. Their cytoplasmic content is protected from degradation, which makes them storable and generally adds to their value as a diagnostic/ prognostic substrate [92–94].
Few clinical studies published so far describe the use of neuronal EVs as brain damage markers in HIE. One study (2015) enrolled 14 newborns (36 weeks of gestation, Apgar score 2–5) diagnosed with HIE and receiving HT starting from within 6 h of life. Blood samples (500 µL) were collected 8, 10 and 14 h after commencement of the therapy. The study used an original protocol for neuronal EV isolation: 100 µL of blood plasma were treated with commercial reagent for exosome precipitation (EXOQ; System Biosciences, Inc.; Mountainview, CA) and the total yield of exosomes was incubated with biotinylated antibodies to contactin-2 — a neuron-specific adhesion molecule. The exosome-Ab complexes were subsequently precipitated with streptavidin to yield a pure fraction of neuronal EVs. Although EV concentrations in the isolates are not specified in the article, they should have been low, providing for measurements of four markers only: synaptopodin, synaptophysin, neuronal enolase and mitochondrial cytochrome-C-oxidase. Of those, only synaptopodin proved to be of diagnostic value; at that, its dynamics reflects secondary damage arising after 6 h of life [95]. It should be noted that such modification of EVs renders them unsuitable for MS-based metabolomic assay. Moreover, very low yields of EVs will hardly afford reliable measurements above the noise thereshold. Another study by the same team (2021) enrolled 26 newborns with HIE (39 weeks of gestation, Apgar score 5); the venous blood samples were collected at 0–6, 12, 24, 48 and 96 h of life. Neuronal EVs were isolated by the same method and analyzed by enzyme immunoassay for a different set of neuronal markers including synaptopodin, neutrophil gelatinase-associated lipocalin (NGAL) and neuropentraxin-2 [96]. NGAL levels have been shown to correlate with severity and outcomes in hemorrhagic stroke, while also being a marker of kidney damage [97, 98]. Neuropentraxin-2 binds α-amino-3hydroxy-5-methyl-4-isoxazolepropionic acid (AMPA) glutamate receptors at excitatory synaptic terminals [99]. Similarly with the previous study, synaptopodin revealed significant prognostic value and correlated with MRI data in cases of mild damage, whereas in more severe cases its prognostic value was nonsignificant and the sensitivity dropped from 40% to 18% within 0–12 h window. Prognostic value of NGAL (with regard to MRI data) was significant in cases of moderate and high severity, but within a 12–48 h window, and no inverse correlation between synaptopodin and NGAL levels was identified [96]. The utility of this approach for clinical diagnostic purposes remains questionable due to the extremely low yields of neuronal EVs.
Thus, proteomic and metabolomic approaches can be applied in differential diagnosis of HIE at early stages of pathogenesis. However, the clinical utility of corresponding brain damage-specific molecular patterns is undermined by the thick background of concomitant damage to other organs during systemic exposure to hypoxia.
CONCLUSION
The continued high rates of neonatal HIE morbidity and mortality necessitate the use of advanced methods to verify the etiology and distinguish it from clinically similar conditions, e.g. early neonatal sepsis and certain congenital metabolic disorders for a timely decision-making on therapeutic hypothermia. The moment of therapy initiation directly affects the long-term neurological outcome: the earlier the commencement, the better the prognosis. The early differential diagnosis of HIE in neonates may involve detection of changes in the metabolite composition of the blood by means of chromatography– mass spectrometry focusing on candidate compounds with the highest diagnostic potential in HIE. The much anticipated translation of 'omics'-based tests, tissue culture models and extracellular vesicle assays into practice will enhance the differentiation of HIE from clinically similar neonatal conditions.