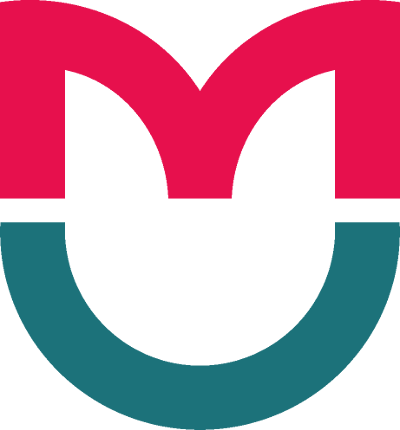
This article is an open access article distributed under the terms and conditions of the Creative Commons Attribution license (CC BY).
ORIGINAL RESEARCH
Amicoumacin-based prodrug development approach
1 Shemyakin and Ovchinnikov Institute of Bioorganic Chemistry of the Russian Academy of Sciences, Moscow, Russia
2 Pirogov Russian National Research Medical University, Moscow, Russia
Correspondence should be addressed: Alexandra Sergeevna Tsarkova
st. Miklukho-Maklaya, 16/10, Moscow, 117997, Russia; moc.liamg@avokrastla
Funding: the work was supported by the grant № 075-15-2021-1049 from the Ministry of Science and Higher Education of the Russian Federation.
Contribution of the authors: Yu. A. Prokopenko — development and isolation of Amikoumacin; V. I. Shmygarev — complete synthesis of an inactivated analog of Amikoumacin A; S. S. Terekhov, M. Yu. Zakharova — in vitro experiments with MPro protease; M. A. Dubinny — NMR spectroscopy and data analysis; A. S. Tsarkova — literature analysis, data processing, article writing; I. V. Yampolsky, I. V. Smirnov - general project management.
Compliance with ethical standards: the work was carried out in compliance with the principles of the Declaration of Helsinki of the World Medical Association.
The 2019 coronavirus disease (COVID-19) has caused catastrophic healthcare and economic crises in many countries around the world [1]. Although several vaccines have been effective in slowing the spread of the SARS-CoV-2 virus, their long-term protection and effectiveness against variants of the virus is still unclear [2–5]. Outbreaks of epidemics caused by coronaviruses in 2002 and 2012 served as a powerful impetus for pharmaceutical and biotechnological research, but only in 2021 did the first drug candidates appear that successfully passed clinical trials [6, 7]. Despite the fact that mass vaccination greatly reduces the spread efficiency and lethality of a viral infection, the remaining high levels of morbidity and mortality [8, 9] require the development of new antiviral drugs for the treatment of COVID-19.
An attractive molecular target for new antiviral drugs is the translational machinery of the infected cell. In this study, the highly potent translational inhibitor Amicoumacin A (Ami), first isolated from Gram-positive bacteria Bacillus pumilus [10], was used to develop a prodrug against SARS-CoV-2. One approach to targeted therapy for coronavirus infection may be proteolytic activation of an Ami-based prodrug. The recognition sequence of the main protease MPro allows for the targeted release of the active Ami molecule and subsequent translation inhibition. The aim of the study was the synthesis of Amicoumacin A inactivated analog, which is a promising tool for the development of drugs aimed at specific translational suppression.
METHODS
NMR spectra (δ, ppm; J, Hz) were recorded on a Bruker Fourier 300 (300 MHz; USA) and Bruker Avance I (700 MHz, USA) instruments at 303 K in CDCl3 and DMSO-d6, internal reference TMS. Mass spectra were recorded on a Waters ACQUITY UPLC H-Class LC/MS System (Waters; USA) by electrospray ionization (ESI). Melting points were determined on an SMP30 apparatus and were not corrected. Analytical and preparative thin layer chromatography was performed on Merck plates (Germany) with a UV-254 fluorescent indicator. Silica gel from Merck (Kieselgel 60, 70–230 mesh) was used for column chromatography. Acros Organics (Thermo Fisher Scientific; Belgium) and SigmaAldrich (Merck; Germany) reagents were used without additional purification. The reactions were carried out using freshly distilled solvents from Khimmed (Russia).
Synthesis of an inactivated derivative of Amicoumacin A
N-tert-butoxycarbonyl-L-glutamic acid dimethyl ester (1)
14.7 g (0.10 mol) of L-glutamic acid were suspended in 75 ml of methanol and 25 ml (0.20 mol) of trimethylsilyl chloride were added dropwise at room temperature. The precipitate gradually dissolved. The reaction mixture was left overnight at room temperature. The next day the solvent was evaporated. To the residue were added 75 ml of methanol, 40 ml (0.3 mol) of triethylamine and 22 g (0.1 mol) of di-tert-butyl carbonate. The reaction mixture was left at room temperature for a day. Control by thin layer chromatography (TLC) ethyl acetate : hexane — 1 : 1 (Rf = 0.36). Then the solvent was evaporated, the mixture was diluted with 200 ml of water and extracted with 3 × 50 ml of ethyl acetate. The organic layer was washed with water, 5% hydrochloric acid, 5% potassium carbonate and again with water. After drying over sodium sulfate, the solvent was evaporated producing 23 g of product 1 in the form of a colorless viscous oil, with 85% yield.
1H NMR (300 MHz, CDCl3) δ 5.09(s, br, 1H), 4.32(s, br, 1H), 3.73(s, 3H), 3.67(s, 3H), 2.47-2.31(m, 2H), 2.24-2.10(m, 1H), 2.03-1.85(m, 1H), 1.43(s, 9H).
13С NMR (75 MHz, CDCl3) δ 173.2, 172.6, 155.3, 80.0, 52.9, 52.4, 51.8, 30.0, 28.3, 27.8
MS (EI): m/z, 276.2 [M+H+].
Dimethyl(2S,4R)-2-[(tert-butoxycarbonyl)-amino]- (cyanomethyl)pentanedioate (2)
To a solution of 7.5 g (27.3 mmol) of N-tert-butoxycarbonyl-L-glutamic acid dimethyl ester (1) in 50 ml of dry tetrahydrofuran (THF) 60 ml (60 mmol) of 1M solution of LiHMDS in THF was added at –80 °C. The reaction mixture was kept at this temperature for 1 h, and then a solution of 1.9 ml (27.3 mol) of bromoacetonitrile in 10 ml of dry THF was slowly added so that the temperature did not rise above –70 °C. TLC control ethyl acetate : hexane — 1 : 1 (Rf = 0.48). After 40 min, a 1M HCl solution was added to the mixture at –70 °C until the pH was neutral, then the organic layer was evaporated. The residue was dissolved in 100 ml of a water-benzene mixture (1 : 1). The organic layer was evaporated and the residue was chromatographed on silica gel, eluent petroleum ether : ethyl acetate (3 : 1), producing 5 g of product 2 with 58% yield.
1H NMR (300 MHz, CDCl3) δ 5.10(s, br, 1H), 4.38(s, br, 1H), 3.75(s, 3H), 3.74(s, 3H), 2.9-2.7(m, 3H), 2.25-2.05(m, 2H), 1.43(s, 9H).
13С NMR (75 MHz, CDCl3) δ 172.4, 172.0, 155.5, 117.1, 80.6, 52.7, 52.6, 51.0, 38.3, 33.9, 28.3, 19.0
MS (EI): m/z, 287.2 [M+H+].
Methyl (2S)-2-[(tert-butoxycarbonyl)amino]-3-[(3S)-2- hydroxypyrrolidin-3-yl]propanoate (3)
2 g (6.4 mmol) of cyanomethyl derivative 2 and 0.83 g (6.4 mmol) of cobalt chloride were dissolved in 40 ml of methanol. Upon cooling to –10 °C, 2.2 g (57 mmol) of dry sodium borohydride was added portionwise. After each portion, the mixture turned black and then brightened again. After 24 hours at room temperature the reaction was complete, according to LC/MS mass spectrometry data. The organic solvent was evaporated, the residue was suspended in ethyl acetate and passed through a thin layer of silica gel, the solvent was evaporated, and the residue was chromatographed on silica gel, eluent ethyl acetate (Rf = 0.16), producing 1.0 g of crystalline substance 3 with 55% yield; melt. point 114 °C
1H NMR (300 MHz, CDCl3) δ 6.42(s, br, 1H), 5.54(d, 8.4 Hz, 1H), 4.30(m, 1H), 3.72(s, 3H), 3.37-3.27(m, 2H) , 2.50-2.35(m, 2H), 2.15-2.05(m, 1H), 1.90-1.75(m, 2H), 1.42(s, 9H).
13C NMR (75 MHz, CDCl3) δ 179.7, 172.9, 155.7, 79.9, 52.4, 52.3, 40.3, 38.1, 34.1, 28.3, 28.1
MS (EI): m/z, 315.3 [M+H+].
Methyl (2S)-2-{[2-(benzyloxycarbonylamino)-4- methylpentanoyl]amino}-3-[(3S)-2-hydroxypyrrolidin-3-yl] propanoate (4)
30 mg (0.104 mmol) of ester 3 was dissolved in 0.3 ml of methylene chloride and 0.2 ml of trifluoroacetic acid was added. After 30 min at room temperature, the starting material disappeared according to TLC data. Trifluoroacetic acid was evaporated on a rotary evaporator. The obtained trifluoroacetate salt was dissolved in 1 ml of methylene chloride, 33 mg (0.13 mmol) of Cbz-L-leucine, 40 mg (0.13 mmol) of TBTU, 15 mg (0.13 mmol) of N-hydroxybenzotriazole were added and cooled to 0 °C. 0.11 ml (0.62 mmol) of diisopropylethylamine was added to the reaction mixture. The mixture was kept in the refrigerator overnight. The reaction mixture was treated with a mixture of potassium carbonate solution and ethyl acetate, the organic layer was washed with water and evaporated. The residue was chromatographed on silica gel, eluent chloroform:methanol 95/5 (Rf = 0.14). 30 mg of product 4 was obtained as a yellowish viscous oil with 66% yield.
1H NMR (300 MHz, CDCl3) δ 7.96 (d, 7.0 Hz, 1H), 7.40-7.21(m, 5H), 6.64 (s, 1H), 5.56(d, 9.0, 1H), 5.07(s,2H) , 4.54-4.30(m, 2H), 3.70(s, 3H), 3.36-3.18(m, 2H), 2.47-2.27(m, 2H), 2.26-2.11(m, 1H), 1.82-1.59(m, 4H), 1.57-1.43(m,1H), 0.95(d,6.1Hz,6H)
13C NMR (75 MHz, CDCl3) δ 179.9, 173.0, 172.2, 156.1, 136.3, 128.5, 128.1, 127.9, 66.8, 53.3, 52.4, 51.4, 42.4, 40.5, 398.3, 32.9, 2.2.1
MS (EI): m/z, 434.4 [M+H+].
Benzyl (1-(((S)-1-(((3S,4S,5S)-1-amino-4,5-dihydroxy-6-(((S)-1- ((S)-8-hydroxy -1-hydroxychroman-3-yl)-3-methylbutyl)amino)- 1,6-dioxohexan-3-yl)amino)-1-oxo-3-((S)-2-oxopyrrolidin-3-yl) propane -2-yl)amino)-4-methyl-1-oxopentan-2-yl)carbamate (5)
4 mg (9 μmol) of dipeptide 4 ester was dissolved in 0.1 ml of methanol and 0.02 ml (20 μmol) of 1M LiOH solution in water was added. After 1 h at room temperature, according to LCMS data, the reaction was complete. The mixture was neutralized with 0.02 ml of a 1M hydrochloric acid solution, the solvents were evaporated on a rotary evaporator. The resulting acid was dissolved in 0.1 ml of DMF, 3 mg (6 μmol) of Amicoumacin A trifluoroacetate, 3.8 mg (11.7 μmol) of TBTU, 6.2 mg (6 μmol) of N-hydroxybenzotriazole were added and cooled to 0 °C. 0.01 ml (54 μmol) of diisopropylethylamine was added to the reaction mixture. 24 hours later, the reaction mixture was diluted with water and extracted with ethyl acetate, after evaporation of the organic solvent, the product was isolated by preparative TLC on silica gel, eluent ethyl acetate: methanol 85/15 (Rf = 0.3). 0.5 mg of product 5 was obtained as a mixture of diastereomers (3 : 1) with 10% yield.
1H and 13C NMR DMSO-d6: see table.
MS (EI): m/z, 825.6 [M+H+].
Amicumacin A Production and Purification
The study used the strain Bacillus pumilus VKM B-3464D (Patent RU2737856C1) [11], alternative name Bacillus pumilus strain 124, GenBank: QENN00000000.1, isolated in 2017 [12]. B. pumilus (GenBank: QENN00000000.1) producing Ami was cultured at 28°C in SYC medium containing 40 g/L sucrose, 5 g/L yeast extract, 4 g/L CaCO3, 1.5 g/L K2HPO4, 2 g/l glucose, 2 g/l NaCl, 1.5 g/l MgSO4, 2 g/l (NH4)2SO4, 0.01 g/l FeSO4, 0.01 g/l MnCl2. B. pumilus was inoculated from an overnight culture (using a dilution of 1 : 100) and cultured in 750 ml flasks in 100 ml of medium on a thermostatically controlled shaker with a shaking intensity of 250 rpm. Cells were centrifuged at 10,000 g for 10 min, and the supernatant was filtered using a Millistak + HC Pod Depth Filter (Millipore, Billerica; USA).
The extraction of Ami involved three chromatographic steps. At the first stage, the supernatant was purified by solid-phase extraction with the LPS-500 sorbent (Technosorbent; Russia) on an XK 26 column (GE Healthcare Life Sciences; USA) using buffer A (10 mM NH4OAc, pH 5.0, 5% ACN), buffer B (10 mM NH4OAc, pH 5.0, 80% ACN), flow rate 6 ml/min, step gradient 0–10 min (0% B), 10–20 min (20% B), 20–36 min (40% B) and 36–45 min (100% B). Fractions containing Ami (40% B) were lyophilized, dissolved in dimethyl sulfoxide (DMSO), and fractionated twice on a RP-HPLC Zorbax ODS 62 × 250 mm column (Agilent; USA) using buffer A and B, flow rate 5 ml/min, gradient 0–10 min (0% B), 10–24 min (0–70% B), 24–25 min (70–100% B). Finally, Ami was purified on a Symmetry C18 5 μm 4.6 × 150 mm (Waters; USA) RP-HPLC column using buffer A and B, flow rate 1 ml/min, gradient 0–5 min (0% B), 5–20 min (0–100% B). Amicumacin A was monitored by absorbance at 315 nm. Ami concentration was measured using εMeOH315nm = 4380 M–1cm–1.
Protease Production and Purification
The codon-optimized sequence of the gene encoding the full-length MPro of SARS-CoV-2 fused to the 6xHis sequence at the C-terminus and to the GST protein at the N-terminus in the pGEX6p plasmid [13] was kindly provided by Prof. Rolf Hilgenfeld. The MPro-encoding gene was flanked by sequences of two protease sites for subsequent processing of full-length MPro: an MPro recognition site for autoprocessing (at the N-terminus of the sequence) and a PreScission™ protease site just before 6×His for its removal, as described previously [13]. The full-length MPro protein was obtained in E. coli
BL21(DE3) cells and purified according to the procedure [13]. The GST-MPro protein was self-processing during expression. MPro-His was purified by metal chelate chromatography using TALON sorbent (Clontech; USA) and treated with PreScission™ Pro (MPro to PreScission ratio as 100:1) for 48 h at 4°C to remove 6×His and obtain MPro with intact C-terminus. The MPro/ PreScission™ Pro mixture was purified using GST-Sepharose (Amersham Biosciences, Germany) and TALON (Clontech; USA) IMAC sorbents. Western blot analysis using antibodies to 6xHis showed that the 6xHis sequence was completely removed. MPro was concentrated to 10 mg/ml in 50 mM Tris-HCl pH 7.5.
Analysis of Cbz-Leu-CG-Ami prodrug activation by MPro protease
The specific activity of MPro was evaluated using the FRET substrate (FRET-S), Dabcyl-KTSAVLQ↓SGFRKM-E(Edans)- NH2 (BPS Bioscience, USA) and corresponded to the pure MPro preparation [14]. Proteolysis of the prodrug was carried out in the presence of 0.1–1 μM MPro in reaction buffer (20 mM Tris-HCl pH 7.3, 100 mM NaCl, 1.0 mM EDTA, and 1.0 mM DTT) using 10 μM Cbz-Leu-CG-Ami at 30°С. The reaction products were analyzed using reverse phase HPLC according to the previously described procedure [15]. Amicoumacin A and its derivatives were detected by absorbance at 315 nm. The concentration was evaluated using the Ami standard, εMeOH315nm = 4380 M–1cm–1.
Cytotoxicity measurement
Cytotoxicity was assessed using the MTT assay using the HEK- 293 cell line (ATCC; USA). A549 cells were seeded at 2 × 105 cells/well in a 96-well microplate in DMEM (Gibco, Invitrogen; USA) with 2 mM L-glutamine (Invitrogen; USA), 10% fetal bovine serum (Gibco; USA), and incubated at 37°C and 5% CO2 for 24 h. After incubation, the medium was removed. Cells were then treated with fresh medium containing varying concentrations of Amicoumacin A and Cbz-Leu-CG-Ami analog in the range of 0.01–50 μg/ml for 48 h. After this procedure, the medium was removed and the cells were treated for 2 h with MTT reagent (concentration 2.5 mg/ml). Live cells converted MTT to formazan, which turns blue-violet when dissolved in DMSO. The solution was removed and 200 μl of DMSO was added to dissolve the formazan. Optical absorbance of treated cells and control (untreated) cells was measured at 570 nm using a Varioskan Flash plate reader (Thermo Fischer Scientific; USA). Cell viability was evaluated as the ratio of optical density of treated cells to control measured in each experiment.
RESULTS
To obtain a peptide that mimics the recognition site of the MPro protease [16], a glutamine analog (cycloglutamine) 3 was synthesized in four steps with a total yield of 27% from glutamic acid [17, 18]. Alkylation of glutamic acid diester 1 proceeds stereoselectively, however, incomplete conversion of the starting diester is observed (fig. 1). Reaction product 2 was purified by normal-phase column chromatography. Nitrile reduction was carried out with sodium borohydride in methanol at –10 °C. In the process of reduction, as a result of a competing reaction, dimerization of compound 2 occurs with the formation of a secondary amine (~15–20%), which is easily separated during chromatographic purification.
After removal of the Boc protecting group from 3, subsequent acylation with Cbz-leucine in the presence of the condensing agent TBTU [16], and ester hydrolysis, the dipeptide 4 containing a methyl protecting group on the carboxyl fragment was obtained (fig. 1).
Amicoumacin A is a particularly potent translation inhibitor in both pro- and eukaryotes [19, 20]. In nature, Ami is produced by probiotic strains of Bacillus pumilus [10, 12], which mediate their antimicrobial activity. For this study, Amicumacin A was obtained by production in B. pumilus bacteria according to the method described previously [21].
At the final stage of the synthesis, after quantitative cleavage of the methyl protecting group with an alkali solution in water, dipeptide 4 was condensed with Amicoumacin A in the presence of TBTU without additional purification (fig. 1). The target product Cbz-Leu-CG-Ami 5 purified by preparative TLC was obtained as a mixture of diastereomers (3 : 1). For target compound 5 1D 1Н, 2D multiplicity-edited 13C-HSQC and 2D TOCSY NMR spectra (Bruker Avance I 700 MHz) were recorded. An analysis of the NMR spectra made it possible to isolate the spin systems and, after comparison with the spectra of the two initial fragments (Amicumacin A and dipeptide 4), unambiguously assign the chemical shifts of protons and 13C atoms attached to them through one chemical bond (table and fig. 2). Modification of Amicoumacin A at 10'-NH2 led to a change in the chemical shifts of the carbon atom 10' (–2.56 ppm) and neighboring atoms 9' and 11' (+1.52 and +1.57 ppm, respectively).
As a result, an inactivated analog of Ami 5 was synthesized and used in the subsequent testing for possible activation by SARS-CoV-2 protease MPro. In contrast to the original Amicoumacin A, which has a highly effective antiproliferative effect (IC50 = 0.06 ± 0.02 μg/ml) [19, 22], the modified Cbz- Leu-CG-Ami 5 derivative did not inhibit cell growth up to an increase in the concentration of 50 μg/ml. Despite the fact that the sequence of the Cbz-Leu-CG peptide allows highly efficient targeting of MPro [16], the Cbz-Leu-CG-Ami molecule was stable in the presence of the MPro protease. It has been shown that less than 3% of free Amicoumacin A can be released in the presence of 1 μM MPro ([S]/[E] = 10-100) within 4 hours.
DISCUSSION
The emergence of COVID-19 in 2019 posed challenges for healthcare professionals to quickly diagnose and provide medical care to patients. Currently, most of the drugs used to treat COVID-19 are non-specific, have a large number of serious side effects, and are applicable only in emergency cases [23]. Only two SARS-CoV-2-specific drug candidates have successfully passed clinical trials [6, 7], one of which has now found application in the drug nirmatrelvir [23]. Currently, an intensive study of the clinical and epidemiological features of the coronavirus disease, as well as the development of new means of its prevention and treatment continues.
The results of the evaluation of the cellular cytotoxicity of the Cbz-Leu-CG-Ami 5 conjugate obtained in this study on the HEK-293 cell line show a decrease in the IC50 of the inactivated Amicoumacin by several orders of magnitude compared to the initial Ami, which indicates that, despite the high efficiency of the natural Amicoumacin A in the inhibition of eukaryotic translation, inactivated analogs can be created on its basis, the subsequent specific activation of which will allow for targeted therapeutic effects. However, the rate of proteolysis of the synthetic derivative Cbz-Leu-CG-Ami is more than four orders of magnitude lower than the rate of proteolysis characteristic of natural substrates, which indicates the need for further optimization of the resulting molecule. The introduction of substituents at the P1' position is critical for the functioning of many proteases, which, apparently, is also characteristic of MPro and requires additional introduction of adapter sequences.
CONCLUSIONS
The development of drugs directed toward specific cellular targets and mechanisms underlies the successful treatment of viral diseases. The present work proposes the development of a new inactivated analog of the natural protein biosynthesis inhibitor Amicoumacin A, modified with the Cbz-Leu-CG peptide sequence recognized by the main MPro protease of the SARS-CoV-2 virus. The study of the ability of the synthetic analog of Ami to be activated by the main SARS-CoV-2 protease revealed the fundamental possibility of creating a prodrug with the indicated principle of action, however, the observed low level of hydrolysis indicates the need for further modification of the resulting molecule. Optimization of the structure and increase in activity of new analogs of Amicoumacin A represent, in our opinion, a promising approach for the development of drug candidates for the treatment of COVID-19.
An attractive molecular target for new antiviral drugs is the translational machinery of the infected cell. In this study, the highly potent translational inhibitor Amicoumacin A (Ami), first isolated from Gram-positive bacteria Bacillus pumilus [10], was used to develop a prodrug against SARS-CoV-2. One approach to targeted therapy for coronavirus infection may be proteolytic activation of an Ami-based prodrug. The recognition sequence of the main protease MPro allows for the targeted release of the active Ami molecule and subsequent translation inhibition. The aim of the study was the synthesis of Amicoumacin A inactivated analog, which is a promising tool for the development of drugs aimed at specific translational suppression.
METHODS
NMR spectra (δ, ppm; J, Hz) were recorded on a Bruker Fourier 300 (300 MHz; USA) and Bruker Avance I (700 MHz, USA) instruments at 303 K in CDCl3 and DMSO-d6, internal reference TMS. Mass spectra were recorded on a Waters ACQUITY UPLC H-Class LC/MS System (Waters; USA) by electrospray ionization (ESI). Melting points were determined on an SMP30 apparatus and were not corrected. Analytical and preparative thin layer chromatography was performed on Merck plates (Germany) with a UV-254 fluorescent indicator. Silica gel from Merck (Kieselgel 60, 70‒230 mesh) was used for column chromatography. Acros Organics (Thermo Fisher Scientific; Belgium) and SigmaAldrich (Merck; Germany) reagents were used without additional purification. The reactions were carried out using freshly distilled solvents from Khimmed (Russia).
Synthesis of an inactivated derivative of Amicoumacin A
N-tert-butoxycarbonyl-L-glutamic acid dimethyl ester (1)
14.7 g (0.10 mol) of L-glutamic acid were suspended in 75 ml of methanol and 25 ml (0.20 mol) of trimethylsilyl chloride were added dropwise at room temperature. The precipitate gradually dissolved. The reaction mixture was left overnight at room temperature. The next day the solvent was evaporated. To the residue were added 75 ml of methanol, 40 ml (0.3 mol) of triethylamine and 22 g (0.1 mol) of di-tert-butyl carbonate. The reaction mixture was left at room temperature for a day. Control by thin layer chromatography (TLC) ethyl acetate : hexane ― 1 : 1 (Rf = 0.36). Then the solvent was evaporated, the mixture was diluted with 200 ml of water and extracted with 3 × 50 ml of ethyl acetate. The organic layer was washed with water, 5% hydrochloric acid, 5% potassium carbonate and again with water. After drying over sodium sulfate, the solvent was evaporated producing 23 g of product 1 in the form of a colorless viscous oil, with 85% yield.
1H NMR (300 MHz, CDCl3) δ 5.09(s, br, 1H), 4.32(s, br, 1H), 3.73(s, 3H), 3.67(s, 3H), 2.47-2.31(m, 2H), 2.24-2.10(m, 1H), 2.03-1.85(m, 1H), 1.43(9H, s).
13С NMR (75 MHz, CDCl3) δ 173.2, 172.6, 155.3, 80.0, 52.9, 52.4, 51.8, 30.0, 28.3, 27.8
MS (EI): m/z, 276.2 [M+H+].
Dimethyl(2S,4R)-2-[(tert-butoxycarbonyl)-amino]-(cyanomethyl)pentanedioate (2)
To a solution of 7.5 g (27.3 mmol) of N-tert-butoxycarbonyl-L-glutamic acid dimethyl ester (1) in 50 ml of dry tetrahydrofuran (THF) 60 ml (60 mmol) of 1M solution of LiHMDS in THF was added at –80 °C. The reaction mixture was kept at this temperature for 1 h, and then a solution of 1.9 ml (27.3 mol) of bromoacetonitrile in 10 ml of dry THF was slowly added so that the temperature did not rise above –70 °C. TLC control ethyl acetate : hexane ― 1 : 1 (Rf = 0.48). After 40 min, a 1M HCl solution was added to the mixture at –70 °C until the pH was neutral, then the organic layer was evaporated. The residue was dissolved in 100 ml of a water-benzene mixture (1 : 1). The organic layer was evaporated and the residue was chromatographed on silica gel, eluent petroleum ether : ethyl acetate (3 : 1), producing 5 g of product 2 with 58% yield.
1H NMR (300 MHz, CDCl3) δ 5.10(s, br, 1H), 4.38(s, br, 1H), 3.75(s, 3H), 3.74(s, 3H), 2.9-2.7(m, 3H), 2.25-2.05(m, 2H), 1.43(9H, s).
13С NMR (75 MHz, CDCl3) δ 172.4, 172.0, 155.5, 117.1, 80.6, 52.7, 52.6, 51.0, 38.3, 33.9, 28.3, 19.0
MS (EI): m/z, 287.2 [M+H+].
Methyl (2S)-2-[(tert-butoxycarbonyl)amino]-3-[(3S)-2-hydroxypyrrolidin-3-yl]propanoate (3)
2 g (6.4 mmol) of cyanomethyl derivative 2 and 0.83 g (6.4 mmol) of cobalt chloride were dissolved in 40 ml of methanol. Upon cooling to –10 °C, 2.2 g (57 mmol) of dry sodium borohydride was added portionwise. After each portion, the mixture turned black and then brightened again. After 24 hours at room temperature the reaction was complete, according to LC/MS mass spectrometry data. The organic solvent was evaporated, the residue was suspended in ethyl acetate and passed through a thin layer of silica gel, the solvent was evaporated, and the residue was chromatographed on silica gel, eluent ethyl acetate (Rf = 0.16), producing 1.0 g of crystalline substance 3 with 55% yield; melt. point 114 °C
1H NMR (300 MHz, CDCl3) δ 6.42(s, br, 1H), 5.54(d, 8.4 Hz, 1H), 4.30(m, 1H), 3.72(s, 3H), 3.37-3.27(m, 2H) , 2.50-2.35(m, 2H), 2.15-2.05(m, 1H), 1.90-1.75(m, 2H), 1.42(9H, s).
13C NMR (75 MHz, CDCl3) δ 179.7, 172.9, 155.7, 79.9, 52.4, 52.3, 40.3, 38.1, 34.1, 28.3, 28.1
MS (EI): m/z, 315.3 [M+H+].
Methyl (2S)-2-{[2-(benzyloxycarbonylamino)-4-methylpentanoyl]amino}-3-[(3S)-2-hydroxypyrrolidin-3-yl]propanoate (4)
30 mg (0.104 mmol) of ester 3 was dissolved in 0.3 ml of methylene chloride and 0.2 ml of trifluoroacetic acid was added. After 30 min at room temperature, the starting material disappeared according to TLC data. Trifluoroacetic acid was evaporated on a rotary evaporator. The obtained trifluoroacetate salt was dissolved in 1 ml of methylene chloride, 33 mg (0.13 mmol) of Cbz-L-leucine, 40 mg (0.13 mmol) of TBTU, 15 mg (0.13 mmol) of N-hydroxybenzotriazole were added and cooled to 0 °C. 0.11 ml (0.62 mmol) of diisopropylethylamine was added to the reaction mixture. The mixture was kept in the refrigerator overnight. The reaction mixture was treated with a mixture of potassium carbonate solution and ethyl acetate, the organic layer was washed with water and evaporated. The residue was chromatographed on silica gel, eluent chloroform:methanol 95/5 (Rf = 0.14). 30 mg of product 4 was obtained as a yellowish viscous oil with 66% yield.
1H NMR (300 MHz, CDCl3) δ 7.96 (d, 7.0 Hz, 1H), 7.40-7.21(m, 5H), 6.64 (s, 1H), 5.56(d, 9.0, 1H), 5.07(s,2H) , 4.54-4.30(m, 2H), 3.70(s, 3H), 3.36-3.18(m, 2H), 2.47-2.27(m, 2H), 2.26-2.11(m, 1H), 1.82-1.59(m, 4H), 1.57-1.43(m,1H), 0.95(d,6.1Hz,6H)
13C NMR (75 MHz, CDCl3) δ 179.9, 173.0, 172.2, 156.1, 136.3, 128.5, 128.1, 127.9, 66.8, 53.3, 52.4, 51.4, 42.4, 40.5, 398.3, 32.9, 2.2.1
MS (EI): m/z, 434.4 [M+H+].
Benzyl (1-(((S)-1-(((3S,4S,5S)-1-amino-4,5-dihydroxy-6-(((S)-1-((S)-8-hydroxy -1-hydroxychroman-3-yl)-3-methylbutyl)amino)-1,6-dioxohexan-3-yl)amino)-1-oxo-3-((S)-2-oxopyrrolidin-3-yl)propane -2-yl)amino)-4-methyl-1-oxopentan-2-yl)carbamate (5)
4 mg (9 µmol) of dipeptide 4 ester was dissolved in 0.1 ml of methanol and 0.02 ml (20 µmol) of 1M LiOH solution in water was added. After 1 h at room temperature, according to LCMS data, the reaction was complete. The mixture was neutralized with 0.02 ml of a 1M hydrochloric acid solution, the solvents were evaporated on a rotary evaporator. The resulting acid was dissolved in 0.1 ml of DMF, 3 mg (6 µmol) of Amicoumacin A trifluoroacetate, 3.8 mg (11.7 µmol) of TBTU, 6.2 mg (6 µmol) of N-hydroxybenzotriazole were added and cooled to 0 °C. 0.01 ml (54 μmol) of diisopropylethylamine was added to the reaction mixture. 24 hours later, the reaction mixture was diluted with water and extracted with ethyl acetate, after evaporation of the organic solvent, the product was isolated by preparative TLC on silica gel, eluent ethyl acetate: methanol 85/15 (Rf = 0.3). 0.5 mg of product 5 was obtained as a mixture of diastereomers (3 : 1) with 10% yield.
1H and 13C NMR DMSO-d6: see table.
MS (EI): m/z, 825.6 [M+H+].
Amicumacin A Production and Purification
The study used the strain Bacillus pumilus VKM B-3464D (Patent RU2737856C1) [11], alternative name Bacillus pumilus strain 124, GenBank: QENN00000000.1, isolated in 2017 [12]. B. pumilus (GenBank: QENN00000000.1) producing Ami was cultured at 28°C in SYC medium containing 40 g/L sucrose, 5 g/L yeast extract, 4 g/L CaCO3, 1.5 g/L K2HPO4, 2 g/l glucose, 2 g/l NaCl, 1.5 g/l MgSO4, 2 g/l (NH4)2SO4, 0.01 g/l FeSO4, 0.01 g/l MnCl2. B. pumilus was inoculated from an overnight culture (using a dilution of 1 : 100) and cultured in 750 ml flasks in 100 ml of medium on a thermostatically controlled shaker with a shaking intensity of 250 rpm. Cells were centrifuged at 10,000 g for 10 min, and the supernatant was filtered using a Millistak + HC Pod Depth Filter (Millipore, Billerica; USA).
The extraction of Ami involved three chromatographic steps. At the first stage, the supernatant was purified by solid-phase extraction with the LPS-500 sorbent (Technosorbent; Russia) on an XK 26 column (GE Healthcare Life Sciences; USA) using buffer A (10 mM NH4OAc, pH 5.0, 5% ACN), buffer B (10 mM NH4OAc, pH 5.0, 80% ACN), flow rate 6 ml/min, step gradient 0–10 min (0% B), 10–20 min (20% B), 20–36 min (40% B) and 36–45 min (100% B). Fractions containing Ami (40% B) were lyophilized, dissolved in dimethyl sulfoxide (DMSO), and fractionated twice on a RP-HPLC Zorbax ODS 62 × 250 mm column (Agilent; USA) using buffer A and B, flow rate 5 ml/min , gradient 0–10 min (0% B), 10–24 min (0–70% B), 24–25 min (70–100% B). Finally, Ami was purified on a Symmetry C18 5 µm 4.6 × 150 mm (Waters; USA) RP-HPLC column using buffer A and B, flow rate 1 ml/min, gradient 0–5 min (0% B), 5–20 min (0–100% B). Amicumacin A was monitored by absorbance at 315 nm. Ami concentration was measured using εMeOH315nm = 4380 M-1cm-1.
Protease Production and Purification
The codon-optimized sequence of the gene encoding the full-length MPro of SARS-CoV-2 fused to the 6xHis sequence at the C-terminus and to the GST protein at the N-terminus in the pGEX6p plasmid [13] was kindly provided by Prof. Rolf Hilgenfeld. The MPro-encoding gene was flanked by sequences of two protease sites for subsequent processing of full-length MPro: an MPro recognition site for autoprocessing (at the N-terminus of the sequence) and a PreScission™ protease site just before 6×His for its removal, as described previously [13]. The full-length MPro protein was obtained in E. coli BL21(DE3) cells and purified according to the procedure [13]. The GST-MPro protein was self-processing during expression. MPro-His was purified by metal chelate chromatography using TALON sorbent (Clontech; USA) and treated with PreScission™ Pro (MPro to PreScission ratio as 100:1) for 48 h at 4°C to remove 6×His and obtain MPro with intact C-terminus. The MPro/PreScission™ Pro mixture was purified using GST-Sepharose (Amersham Biosciences, Germany) and TALON (Clontech; USA) IMAC sorbents. Western blot analysis using antibodies to 6xHis showed that the 6xHis sequence was completely removed. MPro was concentrated to 10 mg/ml in 50 mM Tris-HCl pH 7.5.
Analysis of Cbz-Leu-CG-Ami prodrug activation by MPro protease
The specific activity of MPro was evaluated using the FRET substrate (FRET-S), Dabcyl-KTSAVLQ↓SGFRKM-E(Edans)-NH2 (BPS Bioscience, USA) and corresponded to the pure MPro preparation [14]. Proteolysis of the prodrug was carried out in the presence of 0.1‒1 µM MPro in reaction buffer (20 mM Tris-HCl pH 7.3, 100 mM NaCl, 1.0 mM EDTA, and 1.0 mM DTT) using 10 µM Cbz-Leu-CG-Ami at 30°С. The reaction products were analyzed using reverse phase HPLC according to the previously described procedure [15]. Amicoumacin A and its derivatives were detected by absorbance at 315 nm. The concentration was evaluated using the Ami standard, εMeOH315nm = 4380 M-1cm-1.
Cytotoxicity measurement
Cytotoxicity was assessed using the MTT assay using the HEK-293 cell line (ATCC; USA). A549 cells were seeded at 2 × 105 cells/well in a 96-well microplate in DMEM (Gibco, Invitrogen; USA) with 2 mM L-glutamine (Invitrogen; USA), 10% fetal bovine serum (Gibco; USA), and incubated at 37°C and 5% CO2 for 24 h. After incubation, the medium was removed. Cells were then treated with fresh medium containing varying concentrations of Amicoumacin A and Cbz-Leu-CG-Ami analog in the range of 0.01–50 µg/ml for 48 h. After this procedure, the medium was removed and the cells were treated for 2 h with MTT reagent (concentration 2.5 mg/ml). Live cells converted MTT to formazan, which turns blue-violet when dissolved in DMSO. The solution was removed and 200 μl of DMSO was added to dissolve the formazan. Optical absorbance of treated cells and control (untreated) cells was measured at 570 nm using a Varioskan Flash plate reader (Thermo Fischer Scientific; USA). Cell viability was evaluated as the ratio of optical density of treated cells to control measured in each experiment.
RESULTS
To obtain a peptide that mimics the recognition site of the MPro protease [16], a glutamine analog (cycloglutamine) 3 was synthesized in four steps with a total yield of 27% from glutamic acid [17, 18]. Alkylation of glutamic acid diester 1 proceeds stereoselectively, however, incomplete conversion of the starting diester is observed (fig. 1). Reaction product 2 was purified by normal-phase column chromatography. Nitrile reduction was carried out with sodium borohydride in methanol at –10 °C. In the process of reduction, as a result of a competing reaction, dimerization of compound 2 occurs with the formation of a secondary amine (~15–20%), which is easily separated during chromatographic purification.
After removal of the Boc protecting group from 3, subsequent acylation with Cbz-leucine in the presence of the condensing agent TBTU [16], and ester hydrolysis, the dipeptide 4 containing a methyl protecting group on the carboxyl fragment was obtained (fig. 1).
Amicoumacin A is a particularly potent translation inhibitor in both pro- and eukaryotes [19, 20]. In nature, Ami is produced by probiotic strains of Bacillus pumilus [10, 12], which mediate their antimicrobial activity. For this study, Amicumacin A was obtained by production in B. pumilus bacteria according to the method described previously [21].
At the final stage of the synthesis, after quantitative cleavage of the methyl protecting group with an alkali solution in water, dipeptide 4 was condensed with Amicoumacin A in the presence of TBTU without additional purification (fig. 1). The target product Cbz-Leu-CG-Ami 5 purified by preparative TLC was obtained as a mixture of diastereomers (3 : 1). For target compound 5 1D 1Н, 2D multiplicity-edited 13C-HSQC and 2D TOCSY NMR spectra (Bruker Avance I 700 MHz) were recorded. An analysis of the NMR spectra made it possible to isolate the spin systems and, after comparison with the spectra of the two initial fragments (Amicumacin A and dipeptide 4), unambiguously assign the chemical shifts of protons and 13C atoms attached to them through one chemical bond (table and fig. 2). Modification of Amicoumacin A at 10'-NH2 led to a change in the chemical shifts of the carbon atom 10' (–2.56 ppm) and neighboring atoms 9' and 11' (+1.52 and +1.57 ppm, respectively).
As a result, an inactivated analog of Ami 5 was synthesized and used in the subsequent testing for possible activation by SARS-CoV-2 protease MPro. In contrast to the original Amicoumacin A, which has a highly effective antiproliferative effect (IC50 = 0.06 ± 0.02 μg/ml) [19, 22], the modified Cbz-Leu-CG-Ami 5 derivative did not inhibit cell growth up to an increase in the concentration of 50 μg/ml. Despite the fact that the sequence of the Cbz-Leu-CG peptide allows highly efficient targeting of MPro [16], the Cbz-Leu-CG-Ami molecule was stable in the presence of the MPro protease. It has been shown that less than 3% of free Amicoumacin A can be released in the presence of 1 μM MPro ([S]/[E] = 10-100) within 4 hours.
DISCUSSION
The emergence of COVID-19 in 2019 posed challenges for healthcare professionals to quickly diagnose and provide medical care to patients. Currently, most of the drugs used to treat COVID-19 are non-specific, have a large number of serious side effects, and are applicable only in emergency cases [23]. Only two SARS-CoV-2-specific drug candidates have successfully passed clinical trials [6, 7], one of which has now found application in the drug nirmatrelvir [23]. Currently, an intensive study of the clinical and epidemiological features of the coronavirus disease, as well as the development of new means of its prevention and treatment continues.
The results of the evaluation of the cellular cytotoxicity of the Cbz-Leu-CG-Ami 5 conjugate obtained in this study on the HEK-293 cell line show a decrease in the IC50 of the inactivated Amicoumacin by several orders of magnitude compared to the initial Ami, which indicates that, despite the high efficiency of the natural Amicoumacin A in the inhibition of eukaryotic translation, inactivated analogs can be created on its basis, the subsequent specific activation of which will allow for targeted therapeutic effects. However, the rate of proteolysis of the synthetic derivative Cbz-Leu-CG-Ami is more than four orders of magnitude lower than the rate of proteolysis characteristic of natural substrates, which indicates the need for further optimization of the resulting molecule. The introduction of substituents at the P1' position is critical for the functioning of many proteases, which, apparently, is also characteristic of MPro and requires additional introduction of adapter sequences.
CONCLUSIONS
The development of drugs directed toward specific cellular targets and mechanisms underlies the successful treatment of viral diseases. The present work proposes the development of a new inactivated analog of the natural protein biosynthesis inhibitor Amicoumacin A, modified with the Cbz-Leu-CG peptide sequence recognized by the main MPro protease of the SARS-CoV-2 virus. The study of the ability of the synthetic analog of Ami to be activated by the main SARS-CoV-2 protease revealed the fundamental possibility of creating a prodrug with the indicated principle of action, however, the observed low level of hydrolysis indicates the need for further modification of the resulting molecule. Optimization of the structure and increase in activity of new analogs of Amicoumacin A represent, in our opinion, a promising approach for the development of drug candidates for the treatment of COVID-19.