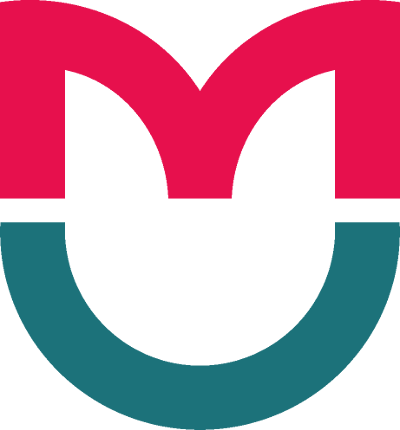
This article is an open access article distributed under the terms and conditions of the Creative Commons Attribution license (CC BY).
ORIGINAL RESEARCH
Tryptophan catabolites and predicted gut flora enzyme-encoding genes
1 Pirogov Russian National Research Medical University, Moscow, Russia
2 Peoples' Friendship University of Russia, Moscow, Russia
3 Center for Digital and Translational Biomedicine, Center for Molecular Health, Moscow, Russia
4 Kazan (Volga Region) Federal University, Kazan, Russia
5 Dmitry Rogachev National Medical Research Center of Pediatric Hematology, Oncology and Immunology, Moscow, Russia
6 Centre for Strategic Planning and Management of Biomedical Health Risks of the Federal Medical Biological Agency, Moscow, Russia
7 National Medical Research Center of Endocrinology, Moscow, Russia
Correspondence should be addressed: Olga P. Shatova
Ostrovityanova, 1, Moscow, 117997, Russia; moc.liamg@po.avotahs
Funding: the study was performed as part of the Assignment № 0373100122119000041 for the project “Creation of a bank of blood serum and fecal samples from healthy donors and patients with obesity, metabolic syndrome, type 2 diabetes mellitus, impaired mucosal barrier of the gastrointestinal tract with the aim of identifying candidate species-specific mediators of the quorum sensing human microbiota systems modulating the endocrine and metabolic function of adipose tissue”.
Author contribution: Shatova OP — primary data acquisition, statistical processing, manuscript writing and preparation of figures; Gaponov AM — manuscript writing; Grigoryeva TV — microbiome assessment; Vasiliev IYu — microbiome assessment and statistical data processing; Stoletova LS — data analysis; Makarov VV, Yudin SM — writing parts of the manuscript; Roumiantsev SA — study concept, manuscript editing; Shestopalov AV — study concept, data analysis, manuscript writing and editing.
Compliance with ethical standards: the study was approved by the Ethics Committee of the Pirogov Russian National Research Medical University (protocol No. 186 of 26 June 2019). All patients submitted the informed consent to the use of biomaterial for scientific purposes.
In the last decade, the research aimed at understanding hologenome has reached a new level due to advances in highthroughput next generation sequencing (NGS) allowing one to accurately identify microbial species and related metabolic pathways. A hologenome is a sum of the host genes and symbiotic/mutualistic microbial genes [1]. There are numerous reports of the studies focused on assessing the taxonomic gut microbiota composition associated with various physiological states and disorders. Many researchers pay close attention to studying the role of dysbiosis in obesity and diabetes mellitus [2], non-specific ulcerative colitis [3] and other gastrointestinal tract disorders [4–6]. There is no doubt, that gut microbiota is a major player in metabolic disorders and the so-called “metabolic inflammation” [7]. It is well known that metabolites produced by microbiota act as signaling molecules [8]. It has been found that indole-3-ethanol, indole-3-pyruvate, and indole-3-aldehyde regulate the apical junctional complex integrity and ensure normal intestinal barrier permeability via the aryl hydrocarbon receptors (AhR) of enterocytes [9, 10]. Thus, microbial tryptophan catabolites (MICT) modulate both intestinal barrier function and resistance to intestinal pathogens [11]. However, there is still little data on regulation of gut microbiota enzymes by tryptophan derivatives. That is why one of the objectives of our study was to explore the relationship between the predicted gut microbiome enzymeencoding gene abundance and the levels of tryptophan catabolites.
Many researchers analyze the features of gut microbiota taxonomic composition in healthy individuals and individuals with various disorders [12, 13], such as obesity, or assess fecal levels of tryptophan catabolites that are more likely to be produced by microbiota only [8]. However, all this has yet to be proven, since microbiota composition in obese patients is still poorly understood [12]. Some authors believe that indole3-acetate is the entirely microbiota-derived metabolite [13], however, an enzyme is expressed in humans that is potentially capable of producing this metabolite. This enzyme, aldehyde dehydrogenase (ЕС: 1.2.1.3), converts indole-3-acetaldehyde to indole-3-acetate [14]. That is why the second objective of our study was to estimate concordance between changes in the levels of tryptophan metabolites and enzymes that could potentially be involved in tryptophan metabolite production. Today, it is believed that MICT include indole, tryptamine, skatole, indole-3-pyruvate, indole-3-lactate, indole-3-acrylate, indole-3-propionate, indole-3-acetamide, indole-3-ethanol, indole-3-aldehyde, and indole-3-acetaldehyde [8]. It should be noted that among all MICT the highest intestinal levels have been reported for indole, which represents both quorum sensing (QS) microbiota molecule and signaling molecule in the human body [15]. It is generally accepted that the main MICT also include indole-3-acetate and indole-3-lactate [16]. Tryptophan acquired from food can be metabolized into indole-3-acetate by gut microbiota via the indole-3-acetamide pathway catalyzed by tryptophan monooxygenase (ЕС: 1.13.12.3) and indole-3-acetamide hydrolase (ЕС: 3.5.1.4). Indole-3-lactate is derived from indole-3-pyruvate as a result of the reduction reaction catalyzed by aromatic 2-oxoacid reductase (ЕС: 1.1.1.110), while indole-3-pyruvate is formed due to three enzymes: tryptophan transaminase (ЕС: 2.6.1.27), L-tryptophan-pyruvate aminotransferase (ЕС: 2.6.1.99), and L-amino acid oxidase (ЕС: 1.4.2.2). Indole-3-lactate can be further converted to indole-3-acrylate by 3-(aryl)acryloylCoA:(R)-3-(aryl)lactate CoA-transferase (ЕС: 2.8.3.17) [17]. It should be noted that metabolite derived from indole-3-lactate (indole-3-acrylate) activates indole pyruvate decarboxylase (ЕС: 4.1.1.74) involved in indole-3-pyruvate conversion via the indole-3-acetate pathway [17] (fig. 1).
The studies focused on exploring obesity have revealed elevated blood levels of indole-3-acetate, indole-3-lactate, and indole in affected individuals [8, 18]. At the same time many authors report impoverishment of microbiota taxonomic composition in obese patients [19, 20]. The role of indole-3acetate and indole-3-propionate in suppression of inflammation contributing to adipose tissue remodeling and insulin resistance has been proven [21]. There is no doubt that correction of the diet results in significant changes of the gut microbiota taxonomic composition. However, it is difficult to say whether these changes will be persistent and how long it will take to return to the dysbiosis phenotype typical for obesity. These indicators will often be specific for each particular patient [22].
Thus, this leaves open the question of the composition of certain tryptophan metabolite producers, homeostatic stability of this composition in healthy and obese individuals, as well as the place these metabolites occupy in the overall metabolic profile of the gut microbial community and their impact on the gut microbiome enzymatic landscape. The “enzymatic landscape” (abundance of enzyme-encoding genes) is the presence and content (in arbitrary units) of genes encoding various enzymes, i.e. the possible presence of enzymes corresponding to the abundance of microbial DNAs potentially capable of expressing certain enzymes.
The study was aimed to assess the levels of tryptophan metabolites in fecal extracts and their correlation with the predicted gut microbiome enzyme-encoding gene abundance in obese patients.
METHODS
Trial arm
A total of 223 patients with an average age of 39.9 ± 4.2 years were surveyed. Two clinical groups were formed. Group 1 (n = 109): control group of healthy volunteers with no obesity and/or metabolic syndrome, average body mass index (BMI) of 19.8 [18.5–22.0] kg/m2 and waist circumference (WC) of 73.0 [68.0–74.5] cm. Inclusion criteria for group 1: age 30–50 years, BMI < 25 kg/m2, WC < 80 cm for females and < 94 cm for males; taking no antibiotics, prebiotics or probiotics within three months before enrollment; submitted informed consent to study participation. Exclusion criteria for group 1: pregnancy and lactation, decompensated chronic disease, cancer, history of congenital tryptophan metabolism disorder, acute viral respiratory infection; any gastrointestinal tract disorder (including non-specific ulcerative colitis, Crohn's disease, irritable bowel syndrome); depression; alcoholism, and no obesity and/or metabolic syndrome. Group 2 (n = 114): study group with obesity and/or metabolic syndrome, average BMI of 33.0 [31.0–36.0] kg/m2 and WC of 90.0 [96.0–105.0] cm. Inclusion criteria for group 2: age 30–50 years, BMI 30–34.9 kg/m2, WC > 80 cm for females and > 94 cm for males; taking no antibiotics, prebiotics or probiotics within three months before enrollment; submitted informed consent to study participation. Exclusion criteria for group 2: pregnancy and lactation, decompensated chronic disease, cancer, history of congenital tryptophan metabolism disorder, acute viral respiratory infection; any gastrointestinal tract disorder (including non-specific ulcerative colitis, Crohn's disease, irritable bowel syndrome); depression; alcoholism. Patients of the study groups did not follow any diet. A stool (feces) sample was obtained from each study participant. Individuals living in the same area (Rostov Region and the city of Rostov-on-Don) in summer and autumn were enrolled in order to minimize the effects of climate, diet and ethnic factors on the gut microbiome.
Chromatographic analysis
Stool samples were obtained from all study participants in accordance with the study protocol. The samples were transported and stored in accordance with the cold chain protocol at a temperature not exceeding –40 °С.
The tryptophan metabolites were quantified in the feces by high-performance liquid chromatography-mass spectrometry (HPLC-MS/MS). Analysis was performed using the Agilent 1290 LC system (Agilent Inc.; USA) equipped with autosampler, thermostatted column compartment, and degasser. During fecal sample preparation, the sample was lyophilized to dryness, then a portion with the weight of about 5 mg was subjected to extraction in 50% aqueous methanol solution supplemented with internal standard and ascorbic acid. After centrifugation the sample was analyzed by HPLCMS/MS.
Chromatographic separation was performed using the Discovery PFP HS F5 column (2.1 × 150 mm; 3 µm, Supelco Inc, St. Louis, Missouri, USA). Detection was carried out in the Agilent 6470 triple quadrupole mass spectrometer (Agilent Inc.; USA) coupled to MRM and electrospray ionisation. The parent and daughter ions that were characteristic of each compound in the MRM mode, as well as ionization and dissociation parameters were optimized using standards for the studied metabolites. The signal received was processed using the Masshunter software (Agilent Inc.; USA).
The column temperature and flow rate were set at 40 °С and 0.4 mL/min, respectively. The mobile phases consisted of 0.1% aqueous formic acid solution (phase А) and acetonitrile (phase В). The gradient program was as follows: 0 min — 1% В; 4 min — 10% В; 9 min — 90% В; 10 min — 90% В; 10.1 — 1% В; 12 min — 1% B. The positive ion electrospray ionization was used. The main MS parameters were as following: gas temperature — 300 °С; gas consumption — 8 L/min; nebulizer gas — 20 pounds per square inch; inert gas heater — 300; inert gas consumption — 10 L/min; capillary voltage — 3500 kV.
Metabolite concentrations were calculated by the internal standard (2-hydroxynicotinic acid) method. The standards for analytes were prepared using artificial matrix containing bovine serum albumin and sodium chloride. The studied metabolites were added to the matrix, and preparation was performed in accordance with the assessment method.
The method was validated based on selectivity, linearity, accuracy, reproducibility, matrix effect, and analyte stability. Validation was performed in accordance with the FDA guidelines on validation of bioanalytical techniques. Fecal samples were lyophilized to dryness, them the sample with the weight of about 5 mg was subjected to extraction in 50% aqueous methanol solution supplemented with internal standard and ascorbic acid. Sample preparation was performed as follows: 100 µL of methanolic fecal extracts (calibrators or QC) were mixed with the internal standard solution, 10 µL of the source solution, 10 µg/mL of the 2-hydroxynicotinic acid solution, and 400 µL of acetonitrile. Then the mixture was shaken, centrifuged for 10 min at 13,000 rpm, and evaporated to dryness in the vacuum centrifugal evaporator at 37 °С. After that the residue was retrieved with 100 µL of 0.02% ascorbic acid solution in 10% methanol, centrifuged, and transferred to the tube for LC-MS. The extract (5 µL) was introduced into the liquid chromatography system for further analysis by HPLC-MS/MS.
16S rRNA gene V3-V4 region sequencing
Bacterial DNA was extracted from fecal samples using the QIAamp Fast DNA Stool Mini Kit (QIAGEN GmbH; Germany). The resulting microbial DNA was amplified using primers specific for the 16S rRNA gene V3-V4 variable region. After the AMPure XP (Beckman Coulter; USA) paramagnetic beads purification of the mixture, the PCR products were indexed using the Nextera XT Index Kit (Illumina, Inc.; USA). The mixture was purified using paramagnetic beads again, and the resulting libraries were sequenced using the MiSeq plarform (Illumina, Inc.; USA) in accordance with the manufacturer’s protocol.
Technical processing of sequencing results
Quality control of the resulting reads was performed using the fastQC tool in accordance with the following criteria: 1) base quality distribution — at least 90% with the quality > 25; 2) base length distribution — at least 90% of reads reach a length of 300 nucleotides; 3) maximum percentage of undefined bases — 1.
The gene sequences obtained were assessed using the QIIME v.1.9.1 tool and the Greengenes v.13.8 reference database with the 97% similarity threshold. The data on the bacterial taxa abundance in the overall pool of reads were obtained as proportions (0–1) calculated based on the number of mapped reads per taxon. The total number of observed operational taxonomic units (Observed OTUs) was calculated in order to characterize the gut microbiome alpha diversity. An operational taxonomic unit is a surrogate taxonomic level, the result of bacterial 16s RNA gene sequencing data clustering.
Bioinformatics analysis
Primary processing of the sequencing results and compilation of the list of OTUs was performed in QIIME v.1.9.1 [23]. The second phase involved analysis of the microbiota members’ probable metabolic role by reconstructing unobservable states with PICRUSt [24].
The predicted enzyme-encoding gene abundance was assessed using bioinformatics analysis involving matching of metagenomic sequencing data with the KEGG Enzyme database using PICRUSt [24]. The resulting predicted enzymeencoding gene abundance data specified in relative units could be compared between samples and cohorts of the studied groups within a project.
Statistical analysis of the study results was performed using the STATISTICA 12.0 software package (StatSoft Inc; USA). All the data sets obtained were tested for normality using the Shapiro–Wilk test. The distribution was normal, and the data were presented as mean and standard deviation.
Spearman's rank correlation coefficients are provided in tables. The correlation analysis involved assessment of the correlation coefficient significance. The differences were considered significant at p < 0.05.
RESULTS
Fecal levels of tryptophan catabolites
Assessment of the tryptophan catabolite fecal levels has shown that indole and indole-3-lactate are dominant MICT (tab. 1).
The fecal levels of eight tryptophan metabolites were significantly lower in adult obese patients than in healthy donors: indole-3-lactate, quinolinic acid, 3-hydroxyindoleacetoacetic acid, anthranilic acid, xanthurenic acid, indole-3carboxaldehyde, indole-3-acrylate, and indole-3-propionate. It should be noted that obese patients have dramatically reduced fecal levels of indole-3-lactate: the average level of this tryptophan metabolite is 78% lower compared to normal (tab. 1).
Correlation of the fecal tryptophan catabolite levels with the predicted abundance of the gut microbiome enzyme-encoding genes
We used PICRUSt analysis of the microbiome taxonomic diversity to quantify the predicted abundance of various enzyme-encoding genes based on the abundance of DNA of various intestinal microorganisms in healthy and obese individuals, then we performed the correlation analysis of the predicted gut microbiome enzyme abundance and the fecal levels of tryptophan catabolites.
It was found that obese individuals had a stronger link between the abundance of the gut microbiota enzyme-encoding genes and the tryptophan metabolites. Thus, we have found 251 significant correlations (significance level = 3, р ≤ 0.001) in healthy subjects, while among obese individuals there are 479 significant correlations with the same significance level (fig. 2).
It was found that there were no significant changes in the fecal indole levels of obese individuals (tab. 1), while the predicted gut microbiome enzyme-encoding gene abundance correlated to the indole levels increased tenfold (fig. 2).
A significant decrease in fecal levels associated with obesity was found for the second dominant intestinal tryptophan catabolite, indole-3-lactate (tab. 1). However, the number of significant correlation pairs for indole-3-lactate and the predicted abundance of genes encoding various gut microbiome enzymes increased from five pairs we had found in healthy individuals to 214 pairs in obese individuals (fig. 2).
In was found that in obese patients the potential key signaling tryptophan metabolites correlated to the predicted gut microbiome enzyme-encoding gene abundance were as follows: indole, indole-3-lactate, kynurenic and quinolinic acids. In non-obese individuals, these key signaling molecules included indole-3-acrylate and anthranilic acid (fig. 2).
Further analysis revealed enzymes, the predicted abundance of genes encoding which was more strongly correlated (Spearman's rank correlation coefficient 0.407–0.340) to the fecal levels of indole-3-lactate (tab. 2).
Among microbiome enzymes, the predicted abundance of genes which is correlated to the intestinal levels of indole-3lactate in obese patients, the enzymes involved in metabolism of carbohydrates, amino acids, polyamines, nucleotides and sulfosaccharides were identified (tab. 2). It was also found that fecal levels of indole-3-lactate correlated with the predicted abundance of genes encoding enzymes involved in metabolism of amino acids: arginine, glutamate and glutamine.
Correlation of the gut microorganism taxonomic abundance with the fecal levels of indole-3-lactate
Analysis of the bacterial and archaeal abundance at the family (f), genus (g) and species (s) levels in healthy subjects showed that there was a significant correlation between the fecal levels of indole-3-lactate and the abundance of the following families of microorganisms: Ruminococcaceae, Lachnospiraceae, Akkermansiaceae, Barnesiellaceae (Appendix 1).
At the same time it was found that in obese patients other members of gut microbiota, such as Enterobacteriaceae and Pseudomonadaceae, were significantly correlated to fecal levels of indole-3-lactate (Appendix 2). It is important to note that in obese individuals microorganisms, the content of which was correlated to intestinal levels of indole-3-lactate, were different from normal. Whereas in healthy individuals a total of 54 significant correlations between various microbial species in the intestine and indole-3-lactate were found, there were three times more significant correlations in obese patients (154 pairs).
Our analysis revealed no significant correlations between indole-3-lactate and the abundance of Klebsiella, Pseudomonas, Escherichia-Shigella in the intestine of individuals with normal weight. It is obvious that in obese individuals indole-3-lactate is produced mainly by the family Enterobacteriaceae. It is important to note that, according to the literature, obesity results in the gut microbiota taxonomic composition impoverishment [19]. However, our study has shown that obesity instead results in enrichment of taxonomic abundance of the gut microbiota being a potential producer of indole-3-lactate.
DISCUSSION
It is interesting to note, that obese individuals show a decrease in the fecal levels of tryptophan catabolites, and the maximum decrease has been determined for indole-3-lactate. Meanwhile, this specific metabolite is a dominant in both healthy and obese individuals. However, we have found that indole-3lactate plays a key role as a potential regulator of the predicted gut microbiome enzyme-encoding gene abundance only in obese individuals. We have identified 214 various enzymes, the predicted abundance of which is potentially dependent on indole-3-lactate.
We believe that the predicted abundances we have determined for the enzymes involved in polyamine metabolism constitute an important target of indole-3-lactate in the pathogenesis of obesity. It is well known that gut microbiota synthesizes polyamines from arginine and its product, ornithine [20]. Polyamine metabolism plays a pivotal role in regulation of the systemic and mucosal adaptive immunity. Arginine, for its part, is an important modulator of the macrophage and T cell metabolism capable of affecting the effector functions of these cells. Furthermore, polyamines inhibit production of proinflammatory cytokines and possess antioxidant effect [25]. In the intestine, polyamines can reduce the cytokine release, thereby promoting reparation of damaged epithelium and restoration of normal barrier function. Spermine (polyamine) inhibits activation of inflammasome, which is a protein complex expressed by epithelial cells that is capable of regulating the IL18 secretion [25]. It has been also shown that the presence of the Bifidobacterium animalis probiotic strain can induce resistance to oxidative stress and contribute to the increase in life expectancy depending on the increased microbial synthesis of polyamines [26]. According to the literature, the elevated polyamine levels in white adipose tissue, liver or skeletal muscles can stimulate energy metabolism and protection from alimentary obesity [27]. It is also known that polyamine metabolites are involved in adipogenesis [28]. It has been shown that treatment with exogenic spermine effectively reduces body weight and fasting glucose levels, it also improves glucose tolerance in mice with obesity caused by diet [29]. Furthermore, spermine affects insulin receptors and insulin sensitivity [30]. Thus, immunological and metabolic effects of polyamines are largely congruent with the effects of the indole AhR signaling. The correlations we have identified may be indicative of the involvement of both indole-3-lactate and polyamines in the mechanisms underlying tolerogenicity (or their impairment) in obese patients; a simultaneous decrease in these two tolerogenicity mechanisms associated with obesity might cause the increase in the intestinal barrier permeability.
The relationship between the fecal levels of indole-3lactate and the predicted abundance of genes encoding the gut microbiome enzymes involved in sulfoglycolysis is poorly understood. The sulfoquinose (SQ) sulfosaccharide is produced by almost all photosynthetic organisms on the Earth and is metabolized by bacteria via sulfoglycolysis. The Embden– Meyerhof–Parnas sulfoglycolytic pathway metabolizes SQ to produce dihydroxyacetone phosphate and sulpholactate aldehyde, it is similar to conventional glycolysis pathway [31, 32]. The literature reports studies focused on identification of microorganisms involved in sulfoglycolysis [33]. However, there are no studies aimed at assessing the relationship between the sulfoglycolysis metabolites and the levels of indole tryptophan metabolites. We have found significant correlations between the predicted abundance of genes encoding the gut microbiome enzymes involved in sulfoglycolysis and the fecal levels of indole-3-lactate in obese individuals (fig. 3). It should be noted that sulfosaccharides represent a reservoir of sulfate that can potentially be used for synthesis of glycosaminoglycans and remodeling of extracellular matrix, which is important for both development of chronic sluggish inflammation associated with obesity and formation of pro-tumorigenic phenotype typical for obese individuals [21].
It is important to note that we have found no significant correlations between the fecal levels of tryptophan catabolites and the abundance of genes encoding the gut microbiome enzymes involved in their production.
Obesity is associated with alteration of the gut microbiota genotype and possibly phenotype: the number of taxa that might supply indole-3-lactate increases three times. Meanwhile, a significant increase in fecal levels of indole-3-lactate can be indicative of severe bacterial colonization of the intestine and increased uptake of this tryptophan catabolite by appropriate microbiota, the taxa typical for obese patients. It is fecal levels of indole-3-lactate for which the number of significant correlations with the predicted abundance of genes encoding the enzymes involved in carbohydrate, lipid, nucleotide and amino acid metabolism, as well as sulfoglycolysis, is increased. Microbiota, including gut microbiota, is believed to be stable and evolutionarily selected at the population level. However, commensal microbiota can be qualitatively and quantitatively modulated [19] by the diet, various signaling molecules and cytokines. Perhaps, enrichment of the indole-3-lactateproducing taxa is of a compensatory nature and occurs to suppress production of pro-inflammatory cytokines that is usually found in obese patients. Thus, it is well known that indole3-lactate has a profound anti-inflammatory effect: it is involved in induction of immunoregulatory T cells and suppression of inflammatory T cells [34]. The recent research has shown that indole-3-lactate is a dominant tryptophan catabolite for Bifidobacterium (B. longum subsp. infantis). Furthermore, rather high levels of indole-3-lactate are found in human breast milk [34]. We have shown that fecal levels of indole-3-lactate in obese patients might depend on the family Enterobacteriaceae taxonomic abundance. Reduction of the share of this family in gut microbiota is correlated to the decrease in indole-3-lactate levels of obese individuals. However, taking into account the increase in serum levels of indole-3-lactate in obese individuals we have revealed earlier [18], it is likely that production of this MICT in the intestine is stable or even increased, while transport of indole-3-lactate from the intestine into blood in obese patients can be intensified. The answer remains to be seen, since not only gut microbiota can be the source of indole-3- lactate, but also microbiota occupying other ecological niches in the human body.
CONCLUSIONS
Indole and indole-3-lactate are dominant tryptophan catabolites in both healthy and obese individuals. Obese patients show a significant decrease in fecal levels of indole-3-lactate, quinolinic acid, 3-hydroxyindole-acetoacetic acid, anthranilic acid, xanthurenic acid, indole-3-carboxaldehyde, indole-3-acrylate, and indole-3-propionate. The maximum decrease is reported specifically for indole-3-lactate. Obese individuals show a stronger link between the predicted gut microbiome enzymeencoding gene abundance and MICT. In the group of healthy subjects, the abundance of gut microbiome enzyme-encoding genes is correlated to the levels of indole-3-acrylate and anthranilic acid, while in obese individuals other MICT (indole and indole-3-lactate) together with quinolinic and kynurenic acids define the gut microbiome “enzymatic landscape”. The more close correlations between the predicted gut microbiome enzyme-encoding gene abundance and MICT have been revealed specifically for indole-3-lactate. It has been found that the fecal levels of indole-3-lactate are correlated to the predicted abundance of genes encoding the gut microbiome enzymes, including those involved in metabolism of carbohydrates, nucleotides, amino acids, polyamines, and sulfosaccharides. No significant correlations between the levels of tryptophan catabolites and the predicted abundance of genes encoding the enzymes involved in tryptophan catabolite production have been revealed. Obese patients of the studied population show the increase in taxonomic diversity of potential indole-3-lactate producers. Potential adjustment of the gut microbiota metabolic activity with tryptophan catabolites can be of preventive and therapeutic significance for obese patients.