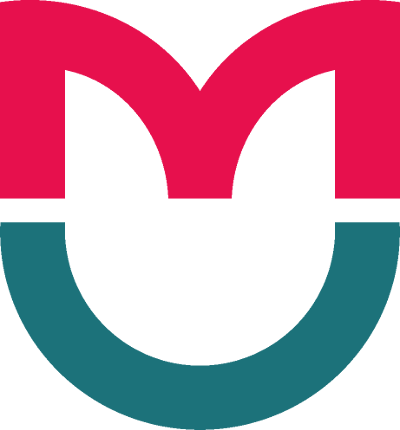
Статья размещена в открытом доступе и распространяется на условиях лицензии Creative Commons Attribution (CC BY).
ОРИГИНАЛЬНОЕ ИССЛЕДОВАНИЕ
Влияние нетранслируемых последовательностей мРНК на иммуногенность мРНК-вакцин против M. tuberculosis у мышей
1 Центральный научно-исследовательский институт туберкулеза, Москва, Россия
2 Автономная некоммерческая образовательная организация высшего образования «Научно-технологический университет «Сириус», Сириус
3 Институт цитологии и генетики Сибирского отделения Российской академии наук, Новосибирск, Россия
Для корреспонденции: Галина Сергеевна Шепелькова
Яузская аллея, д. 2, 107564, г. Москва, Россия; ur.irtc@avoklepehs.g
Финансирование: исследование выполнено при поддержке Министерства науки и высшего образования Российской Федерации (соглашение № 07510-2021-113, уникальный идентификатор проекта РФ----193021X0001).
Благодарности: авторы выражают благодарность сотрудникам АНО ВО «Университет «Сириус» И. М. Теренину за постановку транскрипции in vitro, О. В. Заборовой за формуляцию мРНК в липидные наночастицы.
Вклад авторов: Г. С. Шепелькова — планирование и постановка экспериментов (in vivo и ex vivo), анализ результатов, написание рукописи; В. В. Решетников — клонирование, подготовка мРНК вакцины, написание рукописи; В. Г. Авдиенко — постановка экспериментов (in vivo и ex vivo), анализ результатов; Д. В. Шевырев — подготовка мРНК вакцины, анализ результатов; В. В. Еремеев — дизайн исследования, анализ результатов, написание рукописи; Р. А. Иванов — дизайн исследования, написание рукописи.
Соблюдение этических стандартов: исследование одобрено этическим комитетом ФГБНУ «ЦНИИТ» (протокол № 3/2 от 11 мая 2023 г.), проведено в соответствии с Приказом Минздрава № 755 и Руководством Управления по охране лабораторных животных А5502-01.
- Global tuberculosis report, 2023.
- Lai R, Ogunsola AF, Rakib T, Behar SM. Key advances in vaccine development for tuberculosis-success and challenges. NPJ vaccines. 2023; 8: 158. DOI: 10.1038/s41541-023-00750-7.
- Self WH, Tenforde MW, Rhoads JP, Gaglani M, Ginde AA, Douin DJ, et al. Comparative Effectiveness of Moderna, PfizerBioNTech, and Janssen (Johnson & Johnson) Vaccines in Preventing COVID-19 Hospitalizations Among Adults Without Immunocompromising Conditions — United States, MarchAugust 2021. MMWR. Morbidity and mortality weekly report. 2021; 70: 1337–43, DOI: 10.15585/mmwr.mm7038e1.
- Melo A, de Macedo LS, Invencao M, de Moura IA, da Gama M, de Melo CML, et al. Third-generation vaccines: features of nucleic acid vaccines and strategies to improve their efficiency. Genes. 2022; 13. DOI:10.3390/genes13122287.
- Martinon F, Krishnan S, Lenzen G, Magne R, Gomard E, Guillet JG, et al. Induction of virus-specific cytotoxic T lymphocytes in vivo by liposome-entrapped mRNA. European journal of immunology. 1993; 23: 1719–22, DOI: 10.1002/eji.1830230749.
- Conry RM, LoBuglio AF, Wright M, Sumerel L, Pike MJ, Johanning F, et al. Characterization of a messenger RNA polynucleotide vaccine vector. Cancer research. 1995; 55: 1397–400.
- Anderson BR, Muramatsu H, Jha BK, Silverman RH, Weissman D, Kariko K. Nucleoside modifications in RNA limit activation of 2'–5'-oligoadenylate synthetase and increase resistance to cleavage by RNase L. Nucleic acids research. 2011; 39: 9329– 38. DOI: 10.1093/nar/gkr586.
- Muslimov A, Tereshchenko V, Shevyrev D, Rogova A, Lepik K, Reshetnikov V, et al. The dual role of the innate immune system in the effectiveness of mRNA therapeutics. International journal of molecular sciences. 2023; 24. DOI: 10.3390/ijms241914820.
- Weng Y, Li C, Yang T, Hu B, Zhang M, Guo S, et al. The challenge and prospect of mRNA therapeutics landscape. Biotechnology advances. 2020; 40: 107534. DOI: 10.1016/j.biotechadv.2020.107534.
- Orlandini von Niessen AG, Poleganov MA, Rechner C, Plaschke A, Kranz LM, Fesser S, et al. Improving mRNA-based therapeutic gene delivery by expression-augmenting 3' UTRs identified by cellular library screening. Molecular therapy: the journal of the American Society of Gene Therapy. 2019; 27: 824–36. DOI: 10.1016/j.ymthe.2018.12.011.
- Cao J, Novoa EM, Zhang Z, Chen WCW, Liu D, Choi GCG, et al. High-throughput 5' UTR engineering for enhanced protein production in non-viral gene therapies. Nature communications. 2021; 12: 4138. DOI: 10.1038/s41467-021-24436-7.
- Vasileva O, Tereschenko TV, Krapivin B, Muslimov A, Kukushkin I, Pateev I, et al. Immunogenicity of full-length and multi-epitope mRNA vaccines for M. Tuberculosis as demonstrated by the intensity of T-cell response: a comparative study in mice. Bulletin of RSMU. 2023; 03: 42–48. DOI: 10.24075/brsmu.2023.021.
- Kirshina AS, Kazakova AA, Kolosova ES, Imasheva EA, Vasileva OO, Zaborova OV, et al. Effects of various mRNA-LNP vaccine doses on neuroinflammation in BALB/c mice. Bulletin of RSMU. 2022; 6. DOI: 10.24075/brsmu.2022.068.
- Avdienko VG, Babaian SS, Guseva AN, Kondratiuk NA, Rusakova LI, Averbakh MM, et al. Quantitative, spectral, and serodiagnostic characteristics of antimycobacterial IgG, IgM, and IgA antibodies in patients with pulmonary tuberculosis. Problemy tuberkuleza i boleznei legkikh. 2006; 47–55.
- Nikonenko BV, Apt AS, Mezhlumova MB, Avdienko VG, Yeremeev VV, Moroz AM. Influence of the mouse Bcg, Tbc-1 and xid genes on resistance and immune responses to tuberculosis infection and efficacy of bacille Calmette-Guerin (BCG) vaccination. Clinical and experimental immunology. 1996; 104: 37–43, DOI: 10.1046/j.1365-2249.1996.d01-643.x.
- Kozlova IV, Avdienko VG, Babayan SS, Andrievskaya IYu, Gergert VY. Diagnosis of Bactec samples by immunoglobulins of mouse hyperimmune sera obtained against modified antigens of the cell wall of Mycobacterium tuberculosis. Tuberculosis and Lung Diseases. 2019; 97: 25–30.
- Rijnink WF, Ottenhoff THM, Joosten SA. B-Cells and Antibodies as Contributors to Effector Immune Responses in Tuberculosis. Frontiers in immunology. 2021; 12: 640168. DOI: 10.3389/fimmu.2021.640168.
- Logan J, Shenk T. Adenovirus tripartite leader sequence enhances translation of mRNAs late after infection. Proceedings of the National Academy of Sciences of the United States of America. 1984; 81: 3655–9. DOI: 10.1073/pnas.81.12.3655.
- Kaufman RJ. Identification of the components necessary for adenovirus translational control and their utilization in cDNA expression vectors. Proceedings of the National Academy of Sciences of the United States of America. 1985; 82: 689–93. DOI: 10.1073/pnas.82.3.689.
- Sample PJ, Wang B, Reid DW, Presnyak V, McFadyen IJ, Morris DR, et al. Human 5' UTR design and variant effect prediction from a massively parallel translation assay. Nature biotechnology. 2019; 37: 803–9. DOI: 10.1038/s41587-019-0164-5.
- Kozak M. Features in the 5' non-coding sequences of rabbit alpha and beta-globin mRNAs that affect translational efficiency. Journal of molecular biology. 1994; 235: 95–110. DOI: 10.1016/s0022-2836(05)80019-1.
- Kumari S, Bugaut A, Huppert JL, Balasubramanian S. An RNA G-quadruplex in the 5' UTR of the NRAS proto-oncogene modulates translation. Nature chemical biology. 2007; 3: 218–21. DOI: 10.1038/nchembio864.