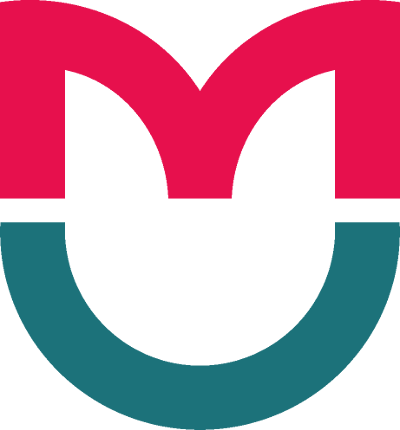
ORIGINAL RESEARCH
Emerging prediction of preeclampsia based on the expression of exosomal SUMO proteins
Kulakov National Medical Research Center for Obstetrics, Gynecology and Perinatology, Moscow, Russia
Correspondence should be addressed: Vladoslava A. Gusar
Akademika Oparina, 4, Moscow, 117997, Russia; ur.liam@rasug_v
Funding: the study was supported by the RSF grant [22-15-00363 “Epigenetic and biochemical aspects of abnormal pregnancy in disturbances of the trophoblast invasive properties: from early diagnosis to prevention of maternal and perinatal morbidity”].
Author contribution: Gusar VA, Timofeeva AV — study concept; Fedorov IS — statistical analysis, graphic design; Gusar VA, Tarasova AM — research procedure (western blotting); Sukhova YuV, Ivanets TYu — providing the clinical basis, assessment of hormones; Gusar VA — data analysis/interpretation, manuscript writing; Timofeeva AV — editing.
Compliance with ethical standards: the study was approved by the Ethics Committee of the Kulakov National Medical Research Center for Obstetrics, Gynecology and Perinatology (protocol № 13 dated 12 October 2015); the informed consent was obtained from all the patients enrolled.
- Ilekis JV, Tsilou E, Fisher S, et al. Placental origins of adverse pregnancy outcomes: potential molecular targets: an Executive Workshop Summary of the Eunice Kennedy Shriver National Institute of Child Health and Human Development. American Journal of Obstetrics and Gynecology. 2016; 215 (1): S1–S46.
- Rana S, Lemoine E, Granger JP, Karumanchi SA. Preeclampsia: pathophysiology, сhallenges, and perspectives. 2019; Circ Res. 124 (7): 1094–1112.
- Staff AC. The two-stage placental model of preeclampsia: An update. Journal of Reproductive Immunology. 2019; 134–135: 1–10.
- Tenório MB, Ferreira RC, Moura FA, Bueno NB, de Oliveira ACM, Goulart MOF. Cross-Talk between Oxidative Stress and Inflammation in Preeclampsia. Oxidative Medicine and Cellular Longevity. 2019; 2019: 1–26.
- Melchiorre K, Giorgione V, Thilaganathan B. The placenta and preeclampsia: villain or victim? American Journal of Obstetrics and Gynecology. 2022; 226 (2): S954–S962.
- Chen CW, Jaffe IZ, Karumanchi SA. Pre-eclampsia and cardiovascular disease. Cardiovascular Research. 2014; 101 (4): 579–86.
- Ritz E, Amann K, Koleganova N, Benz K. Prenatal programming— effects on blood pressure and renal function. Nat Rev Nephrol. 2011; 7 (3): 137–44.
- Poon LC, Shennan A, Hyett JA, et al. The International Federation of Gynecology and Obstetrics ( FIGO ) initiative on preeclampsia: a pragmatic guide for first‐trimester screening and prevention. Int J Gynecol Obstet. 2019; 145 (S1): 1–33.
- ACOG. Obstetrics & Gynecology. 2019; 133 (1): 1–1.
- Yagel S, Cohen SM, Goldman-Wohl D. An integrated model of preeclampsia: a multifaceted syndrome of the maternal cardiovascular-placental-fetal array. American Journal of Obstetrics and Gynecology. 2022; 226 (2): S963–S972.
- Gobble RM, Groesch KA, Chang M, Torry RJ, Torry DS. Differential regulation of human PlGF gene expression in trophoblast and nontrophoblast cells by oxygen tension. Placenta. 2009; 30 (10): 869–75.
- Chang M, Mukherjea D, Gobble RM, Groesch KA, Torry RJ, Torry DS. Glial cell missing regulates Placental Growth Factor (PGF) gene transcription in human trophoblast. Biology of Reproduction. 2008; 78 (5): 841–51.
- Baczyk D, Kibschull M, Mellstrom B, et al. DREAM mediated regulation of GCM1 in the human placental trophoblast. PLoS ONE. 2013; 8 (1): e51837.
- Chou C-C, Chang C, Liu J-H, Chen L-F, Hsiao C-D, Chen H. Small ubiquitin-like modifier modification regulates the DNA binding activity of glial cell missing Drosophila Homolog a. Journal of Biological Chemistry. 2007; 282 (37): 27239–49.
- Enserink JM. Sumo and the cellular stress response. Cell Div. 2015; 10 (1): 4.
- He J, Cheng J, Wang T. SUMOylation-Mediated Response to Mitochondrial Stress. IJMS. 2020; 21 (16): 5657.
- Kunz K, Wagner K, Mendler L, Hölper S, Dehne N, Müller S. SUMO signaling by hypoxic inactivation of SUMO-specific isopeptidases. Cell Reports. 2016; 16 (11): 3075–86.
- Karhausen J, Ulloa L, Yang W. SUMOylation connects cell stress responses and inflammatory control: lessons from the gut as a model organ. Front Immunol. 2021; 12: 646633.
- Chang H-M, Yeh ETH. SUMO: from bench to bedside. Physiological Reviews. 2020; 100 (4): 1599–619.
- Baczyk D, Drewlo S, Kingdom JCP. Emerging role of SUMOylation in placental pathology. Placenta. 2013; 34 (7): 606–12.
- Kondoh K, Akahori H, Muto Y, Terada T. Identification of key genes and pathways associated with preeclampsia by a WGCNA and an evolutionary approach. Genes. 2022; 13 (11): 2134.
- Gurung S, Perocheau D, Touramanidou L, Baruteau J. The exosome journey: from biogenesis to uptake and intracellular signalling. Cell Commun Signal. 2021; 19 (1): 47.
- Robbins PD, Morelli AE. Regulation of immune responses by extracellular vesicles. Nat Rev Immunol. 2014; 14 (3): 195–208.
- Salomon C, Kobayashi M, Ashman K, Sobrevia L, Mitchell MD, Rice GE. Hypoxia-induced changes in the bioactivity of cytotrophoblast-derived exosomes. PLoS ONE. 2013; 8 (11): e79636.
- Tannetta DS, Dragovic RA, Gardiner C, Redman CW, Sargent IL. Characterisation of syncytiotrophoblast vesicles in normal pregnancy and pre-eclampsia: expression of Flt-1 and Endoglin. PLoS ONE. 2013; 8 (2): e56754.
- Salomon C, Guanzon D, Scholz-Romero K, et al. Placental exosomes as early biomarker of preeclampsia: potential role of exosomal microRNAs across gestation. The Journal of Clinical Endocrinology & Metabolism. 2017; 102 (9): 3182–94.
- Villarroya-Beltri C, Gutiérrez-Vázquez C, Sánchez-Cabo F, et al. Sumoylated hnRNPA2B1 controls the sorting of miRNAs into exosomes through binding to specific motifs. Nat Commun. 2013; 4 (1): 2980.
- Gusar VA, Timofeeva AV, Chagovets VV, Kan NE, Ivanets TYu, Sukhikh GT. Regulation of the placental growth factor mediated by sumoylation and expression of miR-652-3p in pregnant women with early-onset preeclampsia. Bull Exp Biol Med. 2022; 174 (1): 174–8.
- Gusar V, Timofeeva A, Chagovets V, et al. Diagnostic potential of exosomal hypoxamiRs in the context of hypoxia–sumoylation– hypoxamiRs in early onset preeclampsia at the preclinical stage. Life. 2022; 12 (1): 101.
- Chiarello DI, Salsoso R, Toledo F, Mate A, Vázquez CM, Sobrevia L. Foetoplacental communication via extracellular vesicles in normal pregnancy and preeclampsia. Molecular Aspects of Medicine. 2018; 60: 69–80.
- Salomon C, Torres MJ, Kobayashi M, et al. A Gestational Ppofile of placental exosomes in maternal plasma and their effects on endothelial cell migration. PLoS ONE. 2014; 9 (6): e98667.
- Tannetta D, Collett G, Vatish M, Redman C, Sargent I. Syncytiotrophoblast extracellular vesicles — Circulating biopsies reflecting placental health. Placenta. 2017; 52: 134–8.
- Hendriks IA, D’Souza RCJ, Yang B, Verlaan-de Vries M, Mann M, Vertegaal ACO. Uncovering global SUMOylation signaling networks in a site-specific manner. Nat Struct Mol Biol. 2014; 21 (10): 927–36.
- Hay RT. SUMO. Molecular Cell. 2005; 18 (1): 1–12.
- Baczyk D, Audette MC, Drewlo S, Levytska K, Kingdom JC. SUMO-4: a novel functional candidate in the human placental protein SUMOylation machinery. PLoS ONE. 2017; 12 (5): e0178056.
- Anderson DB, Wilkinson KA, Henley JM. Protein SUMOylation in neuropathological conditions. Drug News & Perspectives. 2009; 22 (5): 255.
- Bawa-Khalfe T, Yeh ETH. SUMO losing balance: SUMO proteases disrupt SUMO homeostasis to facilitate cancer development and Progression. Genes & Cancer. 2010; 1 (7): 748–52.
- Baczyk D, Audette MC, Coyaud E, Raught B, Kingdom JC. Spatiotemporal distribution of small ubiquitin-like modifiers during human placental development and in response to oxidative and inflammatory stress: Placental distribution of small ubiquitin-like modifiers. J Physiol. 2018; 596 (9): 1587–600.
- Saitoh H, Hinchey J. Functional heterogeneity of small ubiquitinrelated protein modifiers SUMO-1 versus SUMO-2/3. Journal of Biological Chemistry. 2000; 275 (9): 6252–8.
- Hasegawa Y, Yoshida D, Nakamura Y, Sakakibara S. Spatiotemporal distribution of SUMOylation components during mouse brain development: Sumo-ylation during brain development. J Comp Neurol. 2014; 522 (13): 3020–36.
- Chalkiadaki A, Talianidis I. SUMO-dependent compartmentalization in promyelocytic leukemia protein nuclear bodies prevents the access of LRH-1 to chromatin. Molecular and Cellular Biology. 2005; 25 (12): 5095–105.
- Snider NT, Omary MB. Post-translational modifications of intermediate filament proteins: mechanisms and functions. Nat Rev Mol Cell Biol. 2014; 15 (3): 163–77.
- Vassileva MT, Matunis MJ. SUMO modification of heterogeneous nuclear ribonucleoproteins. Molecular and Cellular Biology. 2004; 24 (9): 3623–32.
- Vertegaal ACO, Ogg SC, Jaffray E, et al. A Proteomic study of SUMO-2 target proteins. Journal of Biological Chemistry. 2004; 279 (32): 33791–8.
- Bhattacharjee J, Alahari S, Sallais J, Tagliaferro A, Post M, Caniggia I. Dynamic regulation of HIF1Α stability by SUMO2/3 and SENP3 in the human placenta. Placenta. 2016; 40: 8–17.
- Xu Y, Zuo Y, Zhang H, et al. Induction of SENP1 in endothelial cells contributes to hypoxia-driven VEGF expression and angiogenesis. Journal of Biological Chemistry. 2010; 285 (47): 36682–8.
- Zhou HJ, Xu Z, Wang Z, et al. SUMOylation of VEGFR2 regulates its intracellular trafficking and pathological angiogenesis. Nat Commun. 2018; 9 (1): 3303.
- McCaig D, Lyall F. Hypoxia upregulates GCM1 in human placenta explants. hypertension in pregnancy. 2009; 28 (4): 457–72.
- Kohli S, Hoffmann J, Lochmann F, et al. p45 NF-E2 regulates syncytiotrophoblast differentiation by post-translational GCM1 modifications in human intrauterine growth restriction. Cell Death Dis. 2017; 8 (4): e2730–e2730.
- Chang C-W, Chang G-D, Chen H. A novel cyclic AMP/Epac1/ CaMKI signaling cascade promotes GCM1 desumoylation and placental cell fusion. Molecular and Cellular Biology. 2011; 31 (18): 3820–31.
- Luo J, Ashikaga E, Rubin PP, et al. Receptor trafficking and the regulation of synaptic plasticity by SUMO. Neuromol Med. 2013; 15 (4): 692–706.
- Maynard SE, Min J-Y, Merchan J, et al. Excess placental soluble fms-like tyrosine kinase 1 (sFlt1) may contribute to endothelial dysfunction, hypertension, and proteinuria in preeclampsia. J Clin Invest. 2003; 111 (5): 649–58.
- Redman CWG, Staff AC. Preeclampsia, biomarkers, syncytiotrophoblast stress, and placental capacity. American Journal of Obstetrics and Gynecology. 2015; 213 (4): S9.e1-S9.e4.
- Dymara-Konopka W, Laskowska M, Grywalska E, Hymos A, Błażewicz A, Leszczyńska-Gorzelak B. Similar pro- and antiangiogenic profiles close to delivery in different clinical presentations of two pregnancy syndromes: preeclampsia and fetal growth restriction. IJMS. 2023; 24 (2): 972.
- Keikkala E, Vuorela P, Laivuori H, Romppanen J, Heinonen S, Stenman U-H. First trimester hyperglycosylated human chorionic gonadotrophin in serum — a marker of early-onset preeclampsia. Placenta. 2013; 34 (11): 1059–65.
- Morris RK, Bilagi A, Devani P, Kilby MD. Association of serum PAPP-A levels in first trimester with small for gestational age and adverse pregnancy outcomes: systematic review and metaanalysis: Systematic review association serum PAPP-A and adverse pregnancy outcome. Prenat Diagn. 2017; 37 (3): 253–65.
- Krantz D, Goetzl L, Simpson JL, et al. Association of extreme first-trimester free human chorionic gonadotropin-β, pregnancyassociated plasma protein A, and nuchal translucency with intrauterine growth restriction and other adverse pregnancy outcomes. American Journal of Obstetrics and Gynecology. 2004; 191 (4): 1452–8.