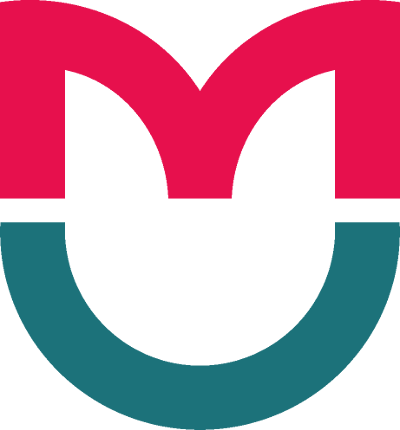
ORIGINAL RESEARCH
Specifics of gut microbiota in women with idiopathic recurrent miscarriage
Medical Academy named after S. I. Georgievsky of Vernadsky Crimean Federal University, Simferopol, Russia
Correspondence should be addressed: Lesya N. Gumenyuk
Bul'var Lenina, 5/7, 295006, Simferopol, Republic of Crimea; ur.liam@kuynemyg_aysel
Author contribution: Gumenyuk LN — study idea and design; Bordyugov MD, Sarchuk EV — data collection, analysis and interpretation; Knyazeva SV, Zastavsky VA — statistical data processing; Kritskaya DV, Saytibragimova SE, Kurtvelieva AI — article authoring.
Compliance with ethical standards: the study was approved by the Ethics Committee of the Medical Academy named after S. I. Georgievsky of Vernadsky Crimean Federal University (Minutes № 10 of October 16, 2021); it was planned and conducted in compliance with the Declaration of Helsinki. All participants of the study signed the voluntary informed consent form.
Recurrent miscarriage (RM) is a heterogeneous condition that implies two or more clinical pregnancy losses before 22nd week of gestation [1]. It is diagnosed in 2-5% of pregnant women [2]. After two miscarriages, the risk of pregnancy termination more than doubles and reaches 36–38% [3].
The discerned etiological factors behind RM are chromosomal abnormalities (2–6%), anatomical changes in the uterus (10–15%), infectious and inflammatory (2–6%), endocrine (17–20%), autoimmune (20%) diseases [4], thrombophilic conditions (10%) [5].
Yet, about 50% of RM cases are idiopathic, i.e., unexplained [6]. Idiopathic recurrent miscarriage (IRM) remains an urgent problem for reproductive medicine worldwide [7].
According to the current concepts, one of the key processes in the pathogenesis of IRM is aberrant production of proinflammatory cytokines accompanied by dysregulation of the immune response [8, 9]. Tumor necrosis factor alpha (TNFα) [10] and interleukin IL17 [11], which offer embryotoxic and antithrophoblastic activity, are of particular interest in this context [12]. There is evidence that women with IRM have significantly higher peripheral blood level of TNFa (40–70% higher than in control group) [13], which is associated with initiation of apoptosis [14] and inhibition of trophoblast invasion [15], activation of thrombokinase [16], deceleration of expression of nucleotide-binding oligomerization domain [17], growth of cytotoxicity of dNK cells [18], all of which, in turn, compromise spiral artery remodeling [16], cause thrombosis, trophoblast infarction and detachment [16], corrupt functions of decidual cells [17], trigger immunological rejection of the fetus [18], and, ultimately, miscarriage. Most researchers believe that women with IRM have elevated serum levels of IL17 [11], so it can be taken as a prognostic factor of the condition [19]. High level of IL17 inversely correlates with the content of Treg cells in peripheral blood and decidual membrane [20], the low level of which potentiates the processes of embryo rejection [21]; it is independently associated with activation of expression of NF-kB (transcription nuclear factor), decelerated expression of progesterone receptors and weakened functional activity thereof, which leads to decidual dysplasia, insufficiency of embryo nutrition, stimulation of myometrial contractility and, in the end, miscarriage [22].
At the same time, the immune system is closely linked to the neuroendocrine system. Teams of researchers actively discuss how dysregulation of the epiphyseal-pituitary-adrenal axis ups the risk of IRM, pointing to the special importance of changes in the secretion of melatonin and cortisol [23, 24]. Analysis of the hormonal profile of blood of women with IRM revealed elevated cortisol and decreased melatonin levels [25, 24]. Moreover, cortisol level fluctuations were associated with important etiological factors of IRM, such as subnormal fibrinolytic activity of the vascular wall, impaired trophoblast invasion and functions [26, 27], induction of apoptosis [28], inhibition of progestrone secretion [29]. In turn, lower blood melatonin level is linked to immunological trophoblast rejection (due to progesterone secretion inhibition) and myometrial contractility stimulation (through reinforced prostaglandin synthesis) [30].
Contemporary research reports confirm that gut microbiota plays a role in the pathophysiology of IRM, since it is crucial to formation and modulation of neuro-immune-endocrine reactions. Convincing data on qualitative changes of microbial makeup in women with IRM have been presented [31]. In general, they tend to have poorer bacterial diversity, depleted useful commensals and abundant pathobionts [32–33]; however, information about their microbiome's generic composition is fragmentary and contradictory. Besides, scientific literature contains only isolated reports on the association between gut microbiota and inflammatory biochemical markers in women with IRM, and there are no studies that investigated the relationship between intestinal bacteria and concentration of cortisol and melatonin in IRM cases.
Thus, exploration of the association between gut microbiota and IRM condition is a relevant problem. In the context of this study, we aimed to analyze taxonomic changes of gut microbiota and assess, at the level of childbearing, its relationship with plasma levels of cortisol, melatonin, TNFa and IL17 in women with IRM.
METHODS
This prospective comparative cross-sectional study was conducted at the gynecological department of the perinatal center of the Semashko State Medical University (Simferopol). It involved 55 women with primary IRM (median age — 31.6 [26.9; 33.9] years), who made up the treatment group (TG), and 60 women with normal pregnancy (NP) (median age — 30.3 [25.9; 33.2] years) who applied for an abortion and made up the control group (CG).
Criteria for inclusion in the TG: confirmed IRM diagnosis; 35 years or age or younger; correct couple karyotype.
Criteria for exclusion from the TG: underweight or overweight; genetic and anatomical causes of RM; chronic infectious, inflammatory, endocrine, autoimmune, thrombophilic, oncological diseases; irritable bowel syndrome; chronic diseases of digestive and hepatobiliary systems; bacterial, viral and fungal infectious diseases; mental pathology; tobacco abuse; stool changes (diarrhea/constipation) and intake of drugs that affect stool within 30 days before the study; vaccination 60 days before the study; intake of antibacterial, probiotic, prebiotic, antiviral, symbiotic, or acid-suppressing drugs within 90 days before the study.
Criteria for inclusion in the CG: 35 years or age or younger; normal pregnancy; uncomplicated gynecological and obstetric anamnesis; one or more successful pregnancies; no chronic extragenital pathology and allergic reactions in the anamnesis; no mental pathology in the anamnesis; no more than 3 respiratory infections a year; no infectious and acute diseases, changes in stool (diarrhea/constipation); no intake of drugs affecting stool within 60 days before the study; no intake of antibacterial, probiotic, prebiotic, antiviral, symbiotic, or acidsuppressing drugs within 90 days before the study.
Criteria for exclusion from the CG: pregravid underweight or overweight; ART pregnancy; high risk of miscarriage; body temperature above 36.9 °C.
Recurrent miscarriage was diagnosed under the codification criteria of the European Society of Human Reproduction and Embryology (ESHRE) [34].
We analyzed taxonomic composition of the gut microbiota of all study participants. Stool samples were collected when the women were admitted to the hospital, in the morning (8.00–11.00); after collection, they were frozen and stored in plastic containers at –80 °C up to the day of metagenomic analysis. Total DNA were extracted with the help of phenols. DNA libraries of 16S rRNA gene fragments were prepared under the standard protocol recommended by the manufacturer of MiSeq SOLiD 5500 Wildfire sequencer (AppliedBiosystems; USA), using primers for V3 and V4 variable regions of the 16S rRNA gene [35]. To filter quality of readings and classify their taxonomy, we used QIIME software (v.1.9.1) [36]. Identification of the taxonomic affiliation of readings was done in two stages: first, we selected a reference set of operational taxonomic units (OTU) of bacteria by comparing our readings of the 16S rRNA gene with data from the GreenGenes database version 13.5 [37]; secondly, we identified taxonomic affiliation of the OTE using the RDP algorithm and referring to HITdb, a specialized database of human gut microbiota [38].
The identified species, genera and phylum of the microorganisms were the basis for assessment of the qualitative and quantitative composition of gut microbiota. To evaluate α-diversity, we calculated the Chao1 index, number of the detected taxa (Sobs), indicator reflecting the real number of taxa (ACE). For this operation, we used Mothur software (v.1.22.0) (https://www.mothur.org).
Serum concentrations of cortisol, melatonin, TNFa, and IL17 were established with the help of solid-phase enzyme immunoassay, using test systems from Vector-Best (Russia) and Immuno Biological Laboratories (Germany). Fasting blood samples were taken from cubital vein in the morning (7.00–10.00), after 15 or more minutes of rest.
STATISTICA 8.0 software package (StatSoft Inc.; USA) was used to statistically process the data obtained. For normal distribution cases, quantitative indicators are given as means and standard deviations, in abnormal distribution situations — as medians (Me) and 25th and 75th percentiles. Qualitative attributes were described with the help of the absolute number of values and percentages. Quantitative group-wise comparison of the data relied on the Student t-test and Mann–Whitney U-test, qualitative comparison — on the chi-squared test. To establish correlation between the values, we calculated the Spearman's rank correlation coefficient. The differences were considered statistically significant at p < 0.05.
RESULTS
Table tab. 1 describes the participants. The groups were comparable in age (p = 0.122) and body mass index (p = 0.087).
Intergroup comparison of gut microbiota revealed a significantly decreased α-diversity in the treatment group (Chao1 p = 0.014), as well as a downward trend of the ACE and Sobs curves (p = 0.053 and p = 0.051, respectively) (fig. 1).
Compared to the CG, women of the TG had significantly decreased abundance of Bifidobacterium (p < 0.001), Lachnospira (p = 0.032), Roseburia (p = 0.003), Coprococcus (p = 0.012) and increased abundance of Ruminococcus (p < 0.001) and Klebsiella (p = 0.002) (fig. 2).
In women with IRM, compared to women with normal pregnancy, levels of plasma cortisol, TNF and IL17 were significantly higher, and melatonin — significantly lower (tab. 2).
We have established statistically significant correlations of cortisol levels with abundance of Lachnospira (r = –0.51, p = 0.002), relationship between melatonin level and abundance of Coprococcus (r = –0.49, p = 0.012), correlations between TNF/IL17 concentrations and Chao1 (r = –0.51, p = 0.002; r = –0.54, p = 0.001, respectively), found TNF concentration to cross-correlate with abundance of Ruminococcus (r = 0.51; p = 0.002), and IL17 concentration to negatively correlate with Bifidobacterium abundance (r = –0.52, p = 0.001).
DISCUSSION
In this study, we refined the data on the changes in taxonomic composition of gut microbiota in women with IRM at the level of childbearing, and established the links between the said changes and plasma concentrations of cortisol, melatonin, TNF and IL17.
A number of previous studies reported gut microbiota alterations in women with IRM [31–33], and in this work, we have also shown that to be significantly different from gut microbiota of women with normal pregnancy. According to our data, women with IRM, compared to women with normal pregnancy, have lower bacterial α-diversity, which is confirmed by a significantly lower Chao1 value. These findings are consistent with the results of earlier studies [33, 39]. In addition, dysbiosis in women with IRM decreased the abundance of bacteria capable of immunomodulation, namely, Bifidobacterium, Lachnospira, Roseburia, Coprococcus, and Prevotella, which are known to produce butyrate and propionate short-chain fatty acids (SCFAs). The respective deficit translated into activation of histone deacetylase and inhibition of G-protein receptors GPR41, GPR43 and GPR109A, and, as a result, development of a chronic inflammation [40]. At the same time, we registered increased abundance of potential pathobionts, namely, bacteria of genera Ruminococcus and Klebsiella. In this respect, out data is only partially consistent with the findings of other researchers. One study reported women with IRM to have low abundance of Prevotella, Roseburia, Lachnospira, and high abundance of Ruminococcus and Klebsiella bacteria [32]. Other studies reported diminishing numbers of Lachnospira, Roseburia, Prevotella [33], and decreasing abundance of Prevotella [31]. These inconsistencies may largely be explained by differences in geographical locations and participant inclusion methodologies. In our study, to prevent distortion of the results, we refrained from enlisting women with a complicated gynecological history and comorbid extragenital pathology, as well as those who took probiotics, prebiotics, and symbiotic drugs within 3 months before the study. Another important factor is the small sample size in the mentioned studies [32, 33].
As already noted, women with IRM had high blood concentrations of cortisol, TNF, IL17, and low concentrations of melatonin; all these hormones and proteins have been confirmed to play a part in the pathogenesis of IRM [13, 11, 23, 24]. Our results are consistent with the findings reported earlier: as a condition, IRM, compared to normal pregnancy, is associated with significantly different plasma concentrations of cortisol, melatonin, TNF, and IL17. A fundamental aspect is that in women with IRM, some gut microbiota species correlate with with plasma concentrations of studied biomarkers; this fact may indicate a relationship between composition, abundance of the microbiota, and IRM. Plasma concentration of cortisol was shown to negatively correlate with the number of Lachnospira bacteria, which points to their possible role in the disruption of operation of hypothalamic-pituitary-adrenal axis (HPAA) in IRM cases. We failed to find studies investigating the relationship between gut microbiota and cortisol in women with IRM. One paper reported patients with Cushing's syndrome to have Lachnospira negatively correlating with cortisol expression [41]. A similar pattern was shown in another study: decreasing abundance of Lachnospira was closely associated with higher cortisol concentrations in healthy children aged 8–16 years [42]. We have found a probable interpretation of this correlation in the literature. Lachnospira is known to be one of the main butyrate-producing species. Since SCFAs can penetrate the blood-brain barrier (through circumventricular organs), it is reasonable to discuss how they act directly on the secretory tone in pituitary neurons of the medial paraventrular nucleus, this modulating activity of HPAA [43]. There are experimental and clinical studies that have shown the ability of SCFAs to affect cortisol expression. In one of such studies, mice were administered SCFAs (67.5 mM acetate + 25 mM propionate + 25 mM butyrate) for seven days, which resulted in inhibition of stress-potentiated corticosterone secretion [44]. Another study reported that seven-day administration of physiological doses of SCFAs directly into the colon of healthy individuals increased their concentration in the systemic bloodstream and attenuated intensity of cortisol response to acute psycho-social stress [45].
There was established a correlation of the level of melatonin with the abundance of Coprococcus, which may be mediated by blocked signals in the system of p-CREB-binding protein, arylalkylamine-N-acetyltransferase, due to the inhibition of tryptophan [46], a precursor of serotonin, from which melatonin is subsequently synthesized. Research papers cover similar associations in patients with juvenile idiopathic arthritis [47] and type 2 diabetes mellitus [48], confirmed by direct close correlations of Coprococcus abundance with the levels of tryptophan metabolites [48] and plasma melatonin [47, 48].
Discussing our results regarding the relationship between gut microbiota and blood levels of TNF and IL17 in the TG, it is important to note that only some of them are consistent with data found in the previously published study reports. Interconnections of gut microbiota and proinflammatory cytokines have been evaluated in an earlier work [33], which found women with IRM to exhibit lower bacterial diversity in association with increasing blood concentrations of TNF and IL17. Our findings are consistent with these results. This fact may indicate that in the patients with IRM, microbiome's proinflammatory effects are probably caused by a holistic dysbiosis. In addition, we have registered direct relationship between TNF blood levels and abundance of Ruminococcus. Ruminococcus bacteria are known to synthesize glucoramnan, an inflammatory lipopolysaccharide, which, by activating TLR4mediated reactions, induces production of proinflammatory cytokines (including TNF) by bone marrow dendritic cells [49]. Currently, a sufficient number of studies indicate that Bifidobacterium can influence the severity of inflammatory reactions [50–54]. Thus, lower abundance of Bifidobacterium is associated with development of preeclampsia [51], shorter life of a heart allograft [50], increased risk of autoimmune conditions [52], and inflammatory bowel diseases [53]. Moreover, low numbers of Bifidobacterium is known to be linked to activation of histone acetylation and suppression of DNA methylation, which, in turn, leads to boosted activation of NF-kB-mediated transcription and intensification of the IL17 expression [50]. The negative correlation between blood concentration of IL17 and abundance of Bifidobacterium that we have registered is an additional evidence of this association. At the same time, our results conflict with findings of the study [33], which established a negative relationship between blood concentration of IL17 and abundance of Prevotella in women with IRM. This inconsistency, as pointed out above, is most likely a consequence of differences in designs of the studies: we did not enlist women with IRM that had gynecological pathologies and metabolic disorders, while the authors of study [33] did not regard polycystic ovary syndrome and insulin resistance as exclusion criteria. This fact could have influenced the differences between TNF and IL17 associations with the gut microbiota species in women with IRM as they are reported in the literature. Nevertheless, the data published in study [33] and the results of our work suggest a significant role played by the gut microbiota in the immunogenesis of IRM. Apparently, the causal relationships between gut microbiota and blood levels of proinflammatory cytokines in IRM cases require further, more scrupulous investigations.
CONCLUSIONS
Women with IRM were found to have pronounced abnormalities in abundance and taxonomic composition of gut microbiota. The statistically significant correlations found between some species in microbiota and hormonal and inflammatory markers confirm the postulate about connections between gut microbiota abundance/diversity and IRM. Modulation of gut microbiota may have preventive and therapeutic effects in women with IRM.