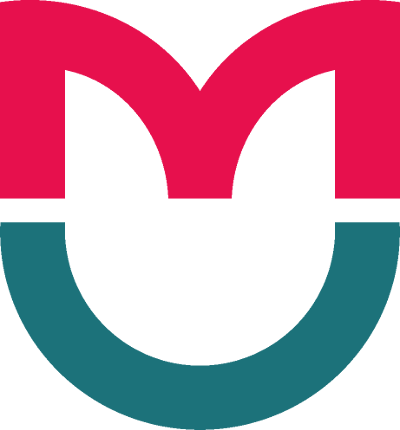
This article is an open access article distributed under the terms and conditions of the Creative Commons Attribution license (CC BY).
ORIGINAL RESEARCH
Comparison of lipid alterations in astrocytomas with increasing grade
1 Skolkovo Institute of Science and Technology, Moscow, Russia
2 Moscow Institute of Physics and Technology, Dolgoprudny, Russia
3 Siberian State Medical University, Tomsk, Russia
4 Semenov Federal Research Center for Chemical Physics, Moscow, Russia
Correspondence should be addressed: Igor A. Popov
Institutskiy per., 9, str. 7, Dolgoprudny, 141701, Russia; ur.tpim@ai.vopop
Funding: the study was performed within the framework of the state assignment of the Ministry of Science and Higher Education of the Russian Federation (agreement № 075-03-2022-107, project № 0714-2020-0006). The study involved the use of equipment of the Semenov Federal Research Center for Chemical Physics RAS.
Author contribution: Pekov SI — concept, data analysis, manuscript writing; Bocharov KV — experimental procedure, resource provision; Bormotov DS — experimental procedure, data analysis; Eliferov VA — experimental procedure, communication with surgeons; Parochkina EV — experimental procedure; Sorokin AA — data analysis, concept; Nikolaev EN — resource provision; Popov IA — search of funding sources, research management.
Compliance with ethical standards: the study was approved by the Ethics Committee of the Burdenko Research Institute of Neurosurgery (protocols № 40 dated 12 April 2016 and № 131 dated 17 July 2018) and conducted in accordance with the principles of the Declaration of Helsinki (2000) and its subsequent revisions. All patients submitted the informed consent to study participation and the use of biomaterial for scientific purposes.
Tumors of the central nervous system are considered to be the most demanding to precision of surgical intervention. The resection completeness and volume are the key parameters affecting the outcome of complex treatment and the long-term overall survival [1–3]. Thus, the tumor margin identification accuracy is the most important parameter controlled during surgery, and the surgeon has to balance between the need to remove the maximum amount of the affected tissue and preservation of adjacent non-affected functional regions of the brain. In this regard, glial tumors characterized by diffuse tumor cell invasion are particularly challenging. Intraoperative computed tomography, magnetic resonance imaging, and rapid histological analysis are widely used as the methods to facilitate the surgeon’s decision-making, however, their substantially time-consuming nature limits the use of the methods for continuous monitoring of the resection area [4, 5]. Ultrasound guidance and fluorescence imaging are convenient and effective, however, the accuracy and specificity of these methods drop to 50% for some tumors [6, 7]. Today, the mass-spectrometry-based molecular profiling methods rapidly transform into a quick universal tool to support neurosurgical procedures [4, 8]. Regardless of the nature of the specific method for the sample collection and ionization, the mass spectrometry methods are based on assessing the features of tumor tissues addressing both changes in the lipid composition of proliferating cells and the set and concentration of specific metabolites, such as N-acetylaspartate, 2-hydroxyglutarate, and glutamic acid [9–11].
The mass spectrometry profiles acquired when developing such methods enable the building of classification and regression models distinguishing cancerous and noncancerous tissue with high sensitivity and specificity [12, 13]. It should be noted that such methods are generally based not on a single marker, but on the combination of multiple peaks in the spectra reflecting metabolic reprogramming occurring during malignant transformation of the tissue [14]. To proliferate, the cancer cells need large amounts of biomass, however, tumor hypovascularization results in the lack of nutrients. Activation of anaerobic glycolysis, even in the presence of oxygen (Warburg effect) [15], also leads to activation of fatty acid synthesis and acceleration of lipogenesis. The synthesis of saturated fatty acids and the lack of dietary unsaturated fatty acids result in significant changes in the lipid composition of cells, including redistribution of fatty acid residues among triglycerides and phospholipids [16]. Despite the fact that the biochemical basis explaining the differences in the lipid composition between glioma cells and healthy glial cells is well known, no thorough tumor lipidome investigation has yet been carried out, since such studies usually involve cell lines or xenografts, which do not fully reflect the tumors’ natural biological variability and physiological environment [17]. Diffuse tumors are still challenging for metabolomic studies due to difficulty in obtaining enough tumor material containing no incorporated cells of other types for assessment.
A substantial amount of data obtained by mass spectrometry-based molecular profiling enables investigation of metabolic alterations even in heterogeneous samples, since the use of advanced mathematical approaches makes it possible to identify the characteristic features of molecular profiles distinguishing the tissues, by separating these features from natural biological variability and the contributions of other cells present in the sample [16, 18]. The study was aimed to identify the differences in the lipid composition of grade 2 and 3 astrocytomas in order to confirm the suspected metabolic alterations associated with malignant transformation of tissues.
METHODS
All the samples included in the study were collected by the neurosurgeon, who monitored the progress of surgical procedure using the neuronavigation systems or visually. Composition of the sample that had to consist mostly of tumor tissue was the main selection criterion. The areas showing signs of necrosis and comprising a large number of blood vessels were not selected for the study. The tissue samples were washed with sterile saline to remove the remaining blood and divided into two visually similar parts. The first part was frozen and stored at –80 °C prior to the study. The second part was used by a professional pathologist to conduct histological assessment using the routine protocol. The samples containing less than 75% tumor cells and the samples showing signs of necrosis were excluded from the study based on the pathology report. Thus, a total of 28 anaplastic astrocytoma tissue samples (grade 3; collected from 23 patients) and 15 diffuse astrocytoma tissue samples (grade 2; collected from 12 patients) were selected.
All the collected samples were analyzed at random. Furthermore, different samples obtained from the same patient were not analyzed on the same day in order to minimize possible bias. Each tissue sample was thawed just before the analysis and divided into 2–4 parts depending on the size. These parts were immediately subjected for mass spectrometry-based profiling. A total of 81 mass spectrometry profiles of anaplastic astrocytoma samples and 46 mass spectrometry profiles of diffuse astrocytoma samples were acquired.
All the samples were analyzed using Inline Cartridge Extraction (ICE) [19]. The tissue sample of about 1 mm3 was placed in a disposable cartridge, which was a stainless steel tube having a fused silica electrospray emitter on one end and sealed with an input polyether ether ketone (PEEK) capillary on the other end. The fused silica emitter was sealed against entry of tissue particles with a glass microfiber filter. The sample in the cartridge was washed with 100 µL of the solution containing isopropanol, methanol, acetonitrile, and water (3 : 3 : 3 : 1 v/v) supplemented with 0.1% formic acid (v/v), then the cartridge was mounted into the ion source of a mass spectrometer. Extraction was performed by continuously supplying the above solvent with the flow rate of 3 µL/min. Mass spectra were acquired using the LTQ XL Orbitrap ETD mass spectrometer (Thermo Fisher Scientific; San Jose, USA) with the 30,000 resolution (at m/z 400) within the range of m/z 500–1000, in both polarities consequently.
Peaks with the signal-to-noise ratio less than 2 were removed from mass spectra, then the peaks found in less than 25% of individual scans in each group were removed. Then, only peaks with intensity values exceeding the median were selected for further analysis. Extraction of the peaks characterizing each group of samples was performed by shrinkage discriminant analysis (SDA) [20]. Significance of each extracted peak was assessed using the lfdr criterion (local false discovery rate) showing the probability that an individual peak would not have an impact on the sample classification. The lfdr threshold value of 33% was selected.
Two tissue samples with the maximum volume in each group were also used for identification of lipids. For that approximately 10 mg of the tissue were homogenized in 400 µL of the above solution on ice. The homogenate was processed in the ultrasonic bath for 5 min and centrifuged at 21,000 g for 15 min at a temperature of 4 °C. Supernatant was transferred to the chromatography vial and subjected to vacuum evaporation. Lipids were redissolved in 20 µL of the solution containing n-butanol, isopropanol, and water (8 : 21 : 69 v/v) supplemented with 5 mМ phosphoric acid. The analysis of lipids was performed by the reverse phase nanoHPLC–HRMS/ MS using the above mass spectrometer. Lipids were identified using the LipiDex software.
RESULTS
The limited dynamic range of mass analyzers does not allow us to draw firm quantitative conclusions about the levels of molecules in the original biological sample. However, relative peak intensities change in proportion to changes in the levels of appropriate molecules against the background of other compounds of the selected class. The mixture of solvents used in the study effectively dissolves lipids, especially polar lipids of the cell membranes, which are easy to ionize and detect in the selected mass range. Thus, the molecular profiles acquired during this study correspond mainly to the lipid component of the cell. The use of SDA [20], the subtype of linear discriminant analysis considering internal correlations caused by natural distribution of stable isotopes in biological molecules (mainly 13C for lipids), makes it possible to identify ions, the intensities of which vary between samples of the assessed groups. The CAT score (correlation-adjusted t-score) calculated during this procedure represents an analogue of Student’s t-test considering both internal correlations together with the higher dimension of raw data and the peak intensity distribution deviation from normal. The CAT score demonstrates the individual peak intensity deviation from the average towards appropriate group. As a result, a total of 13 ions of lipids were identified, the levels of which were higher in the diffuse astrocytoma samples than in the anaplastic astrocytoma samples, along with three ions of lipids, the levels of which were elevated based on spectra corresponding to the anaplastic astrocytoma samples (Table). Chemical identification of the ions was performed by chromatography– tandem mass spectrometry based on the lipid extraction from the largest tissue samples, which was likely to be impossible for the majority of samples due to small amounts of available biomaterial. Thus, the problem of preserving natural biological variability of the assessed tumors and interpatient variability recording was solved through mass spectrometry-based tissue profiling without sample preparation.
All the extracted peaks are not among the most intense in the mass spectra; these are considered the second and third quartiles of the intensity distribution. This is due to the fact that the most and the least intense peaks strongly correlate with the natural variability and can vary considerably between patients, which makes their intragroup variability comparable with the intergroup variability in the sample of this size. However, the results obtained demonstrate the decrease in the diversity of lipid composition with increasing grade, which is in line with the overall pattern reported earlier when comparing grade 4 glial tumors with the non-cancerous control samples [16].
DISCUSSION
The reported differences in lipid composition between grade 2 and 3 astrocytomas demonstrate the decrease in the levels of phospholipids containing polyunsaturated fatty acids, which suggests the increase in the share of fatty acids de novo synthesized in the glioma cell membranes with increasing glioma grade. A decrease in the levels of phospholipids with fully saturated fatty acid residues is observed, because, according to the previous studies, the most aggressive glioma cells accumulate fatty acids in the lipid droplets to use them to maintain metabolic activity in the later stages of the disease, when the nutrient availability is reduced even more [15, 21].
It should be noted that the relative content of phosphatidylcholines PC(32:1), PC(34:1), and PC(34:2) is not fully compliant with the overall pattern of astrocytoma growth. Thus, it has been shown that these lipids largely characterize the high-grade cancerous, but not non-cancerous, brain tissue [16], while comparison of diffuse and anaplastic astrocytomas has shown that these lipids are typical for the less malignant form. This observation suggests irregular pattern of the decrease in the levels of various classes of lipids with increasing grade, which results in the increase in the observed share of the above lecithins in diffuse astrocytomas and demonstrates the restriction imposed by the ambient ionization mass spectrometry-based profiling on the possibility of assessing lipid composition without applying more informative chromatography–mass spectrometry methods. The analysis of the overall fatty acid composition of certain lipids that can be performed by ambient inonization mass spectrometry does not enable distinguishing isomeric lipids. Thus, for example, the PC(34:1) phospholipid can consist of palmitoleic and stearic acid residues or palmitic and oleic acid residues. Among fatty acids, it is palmitic acid that is the most accessible for incorporation into lipids with increasing grade, since it is the main product released due to functioning of the NADPH-dependent fatty acid synthase [14]. It should be noted that the pentose phosphate pathway activated due to Warburg effect results in the increased NADPH generation by the tumor cell [22]. Thus, the increasing grade can be associated with both spending the pool of phospholipids containing 32–34 carbon atoms in their fatty acid chains [21, 23] and active replacement of those with the isomeric lipids containing fatty acid chains synthesized de novo in cases of astrocytoma progression to grade 4.
CONCLUSIONS
The use of molecular profiling methods makes it possible to study changes in the lipid composition of cancerous tissues of various grades considering their natural biological variability. The trend towards reducing the diversity of polar lipids contained in the cell membranes is observed during the astrocytoma progression to a higher grade. The length and degree of unsaturation of the fatty acid residues constituting these lipids are also reduced. Such an effect is associated with the fatty acid synthesis activation due to metabolic reprogramming of glial cells during their malignant transformation; it is also associated with activation of fatty acid beta-oxidation required to maintain the cells’ vital activity in cases of high-grade glial tumors.