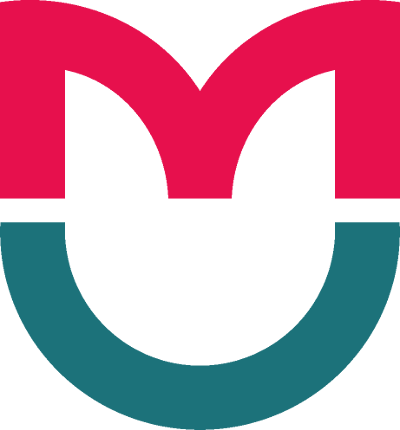
This article is an open access article distributed under the terms and conditions of the Creative Commons Attribution license (CC BY).
ORIGINAL RESEARCH
Effects of biocompatible piezoelectric membranes on the development of fibrosis associated with the oral mucosal wound regeneration
1 Siberian State Medical University, Tomsk, Russia
2 Tomsk Polytechnic University, Tomsk, Russia
3 Montana State University, Bozeman, MT, USA
Correspondence should be addressed: Anastasiia D. Koniaeva
Moskovskij trakt, 2, Tomsk, 634034, Russia; moc.liamg@59aynokaysa
Funding: the study was supported by the RSF (research project No. 23-25-00346)
Author contribution: Koniaeva AD, Varakuta EYu, Bolbasov EN, Stankevich KS — study concept and design; Koniaeva AD, Leiman AE, Kormashov GM, Fedosova MV — data acquisition and processing; Koniaeva AD, Varakuta EYu — manuscript writing; Koniaeva AD, Varakuta EYu, Bolbasov EN, Stankevich KS — manuscript editing.
Compliance with ethical standards: the study was approved by the IACUC of the Siberian State Medical University (protocol No. 11-1 dated 12 July 2022). Rats were handled in accordance with the Directive 2010/63/EU of the European Parliament and of the Council on the protection of animals used for scientific purposes dated 22 September 2010.
Fibrosis is one option for the oral mucosal wound defect repair [1]. However, the presence of fibrosis results in the soft tissue volume reduction, adversely affects the damaged area architectonics and further dental treatment [2].
A number of predictors, the analysis of the severity of which can predict the risk of fibrosis, can be distinguished at the early stages of wound defect regeneration. It is noted that the fibroblast–macrophage reciprocal interactions change depending on the wound healing stage and probability of the risk of fibrosis [3].
The transforming growth factor — (TGFβ) release by cell populations at the wound defect site also indicates the development of fibrosis. TGFβ is released by fibroblasts, macrophages, and other cells of the wound bed; granulation tissue formation, collagen synthesis, and angiogenesis are stimulated against the background of the TGFβ expression increase [4]. However, the TGFβ expression levels and effects on the development of dense fibrous connective tissue depend heavily on microenvironment and cellular context. TGFβ either stimulates, or inhibits cell proliferation. It acts as a chemoattractant for monocytes and fibroblasts; high TGFβ expression levels during the first phase of wound regeneration contribute to granulation tissue development. However, the increased TGFβ expression is accompanied by severe cell infiltration and reduced collagenolysis in the final phase of repair [5]. CD68, the macrophage activity marker, can be also assessed in order to predict the wound healing variant [6].
Efficacy of the use of various biocompatible materials for wound defect closure can be assessed based on the analysis of the above indicators.
There are no data on assessment of the efficacy of using wound coverings with similar physical and chemical properties for oral mucosal wound defect regeneration in the literature.
The study was aimed to perform morphological assessment of the effects of biocompatible piezoelectric membranes on the fibrous tissue formation during regeneration of the oral mucosal wounds.
METHODS
The experiment represented a simple comparative study of two approaches to treatment of the oral mucosal wound defects (involving/not involving closure of the defect with a biocompatible covering).
A polymeric piezoelectric membrane assessed in our study represents the nonwoven fabric made of vinylidene fluoride/tetrafluoroethylene copolymer by electrospinning. The membrane consists of two layers: hydrophilic one facing the wound defect and hydrophobic external one. The membrane is not biodegradable: it is removed from the surface of the healed wound defect after the end of repair. The earlier studies have shown that the fact of piezoelectric properties in the membranes compared to dielectric membranes contributes to the accelerated formation of loose fibrous connective tissue with the smaller specific area of dense fibrous connective tissue. Coverings were also tested for biocompatibility and cytotoxicity using the 3T3L1 fibroblasts at the laboratory of biopolymers and biotechnology of the Tomsk State University [7].
The study involved 45 Wistar rats (4-month-old males with the body weight of 350 ± 30 g), 15 animals per group. Group 1 (n = 15) included animals with open wound defects, group 2 (n = 15) included animals with the wound defects closed with polymeric piezoelectric membranes, group 3 (n = 15) included animals with intact mucosa. Animals were divided into groups by block randomization. Rats were provided by the Goldberg Research Institute of Pharmacology and Regenerative Medicine (Tomsk, Russia). The experiment was conducted after the two-week acclimatization of animals. All rats were kept under standard conditions: in the separate labeled cages, five animals per cage, with ad libitum access to food and water starting from day 2 of the study, at the temperature of +12 °C to +18 °C [8].
This animal species was selected as a research object due to a number of factors: high degree of the experimental modeling data translation relative to humans, genetic homogeneity (homozygosity) determining consistency of response to the exposure to physical and chemical, physiological, biopathogenic, and stress factors, well-known level of sensitivity to stimuli; specificity and levels of biochemical, immunological, functional, and morphological indicators [9].
The mucosal flap sized 7 × 4 mm was excised from the animal’s buccal area in order to simulate the oral mucosal wound defect. After that the membrane was secured to the wound edges with the loop sutures in animals of group 2. All surgical procedures were conducted after putting the animals under anesthesia using Zoletil 100 in a dose of 10 mg/kg (Virbac, Carros; France). The criteria for exclusion of animals from the study with subsequent euthanasia were as follows: general deterioration characterized by lethargy, apathy, refusal to eat, sleep disorder or the animal’s premature death. None of the animals was excluded from the study.
The animals were withdrawn from the study on days 3, 7, and 12 (five animals per group) by inducing hypoxia in the СО2 chamber.
Tissue specimens for histological assessment were collected from the wound defect site by seizing 1 mm of the tissue from the margins. The tissues collected during autopsy were fixed in the 10% neutral formalin solution (Biovitrum; Russia), washed with running water, dehydrated with ascending alcohol concentrations and isopropanol (Biovitrum; Russia), and embedded in paraffin (Biovitrum; Russia). The 5 µm histological sections were cut using the МS-1 sliding microtome (Orion-Medic; Russia). Sections were stained with hematoxylin and eosin (ABRIS+; Russia) and van Gieson's picrofuchsin (Biovitrum; Russia). Semithin sections were toluidine blue stained.
We performed qualitative and quantitative histological assessment of the fibrous connective tissue specific area, macrophage and fibroblast numerical density in 1 mm2 of the section. Histological sections were examined using the Axioskop 40 light microscope (Carl Zeiss AG; Germany) with the 40× and 90× lenses, 10× eye lenses). A total of 50 fields of view from the wound defect site per group were examined.
Immunohistochemical staining involved the use of the recombinant rabbit polyclonal antibody CD68 and isotype I IgG TGFβ (Abcam; USA). The McCarthy immunohistochemical staining intensity was graded [10]: 0 — no detectable staining, 1 — weak staining, 2 — moderate staining, 3 — strong staining, 4 — very strong staining. Formula:
Histochemical scores = ∑ P(i) × i,
where i — staining intensity graded on a scale of 0‒4,
P(i) — percentage of cells stained with different intensity.
Enumeration was performed in three cohorts of 100 cells in various fields of view (40×, 90× lens).
Images were process using the Axio Vision 4.8.2 (Carl Zeiss AG; Germany) and ImageJ v.1.54u (National Institutes of Health, Maryland) tools.
To assess the macrophage and fibroblast fine structure, we prepared samples for electron microscopy by standard method: fixed tissues in the 2.5% glutaraldehyde solution and cacodylate buffer (0.2 М; 1 : 9), applied postfixation with 1% osmium tetroxide, dehydrated and embedded in the araldite M and epon mixture. Sections were cut with the LKB-5 ultramicrotome (BROMMA; Sweden); the uranyl acetate and lead citrate contrast enhancement was applied to the slides. Fine structure was assessed using the JEOL JEM -1400 CX electron microscope (Jeol; Japan).
Statistical processing
The Statistica v. 10.0 software (StatSoft Inc.; USA) was used for in vivo analysis. The hypothesis of trait distribution was tested for normality using the Kolmogorov–Smirnov test. All the results were presented as the median and quartiles, М (Q1; Q3). The nonparametric Kruskal–Wallis test with the median test was used to compare independent samples; the Wilcoxon test was used for pairwise comparison. The differences were considered significant at р < 0.05.
RESULTS
On day 3 of the study, there was granulation tissue at the wound defect site in the experimental groups; sporadic bundles of connective tissue fibers were found in the group with covering. Cell infiltration represented mainly by neutrophils was visible at the wound defect site. Furthermore, macrophages and fibroblasts were detected, which were more often visualized in the group with covering (fig. 1A). Thus, the fibroblast numerical density was significantly (1.4 times) higher in the group with the wound covering (р = 0.035), than in the group with no covering, and significantly (14 times) higher (р = 0.012), than in the intact mucosa (see Table). We can say that mostly small immature fibroblasts with the moderately developed organelles involved in synthesis were visualized during ultra-microscopic examination. Macrophage infiltration was also more prominent in the group with membranes, the numerical density of this cell population was significantly (2.3 and 10.7 times) higher, than in the group with open wound defects and the control group, respectively (р = 0.034, р = 0.018). At the ultra-microscopic level, macrophages showed signs of high phagocytic and synthetic activity, which affected the CD68 marker expression that was significantly (2.6- and 4.2-fold) increased in the group with no covering and the group with membranes, respectively, compared to the values for the intact mucosa (р = 0.022, р = 0.031). Higher expression of the TGFβ1 marker was observed in the group with open wound defects: H-score of the group was significantly (1.4 times) higher (р = 0.045), than that of the group with membranes, and significantly (6.3 times) higher, than that of intact mucosa (р = 0.022) (see Table) (fig. 1B).
On day 7 of the study, the newly-formed loose fibrous connective tissue, between the fibers of which clusters of macrophages and fibroblasts were found, was visualized in the experimental groups (fig. 1C). In this phase of wound regeneration, macrophages and fibroblasts were most often found in all fields of view. The macrophage numerical density reached its maximum in the group with no covering and was significantly (1.6 and 11.3 times) higher compared to appropriate values reported for the group with polymeric membranes and the control group (р = 0.019, р = 0.011) (see Table). This correlated with the CD68 marker expression, the H-score of which in the group with no covering was significantly (1.2 and 6.5 times) higher, than in the group with covering and the group with intact mucosa (р = 0.048, р = 0.024). Furthermore, the fibroblast numerical density, in contrast, reached its maximum in the group with membranes and was significantly (1.35) higher compared to the group with no membranes (р = 0.041) (see Table). Immature fibroblasts still prevailed in the group with no covering, while in the group, where membranes were used, the differentiated mature fibroblasts showing ultrastructural signs of increased synthetic activity were most often visualized (fig. 1D). No foreign body reaction at the membrane implantation site was observed in any phase of the experiment. The TGFβ1 marker expression was significantly (2.8 times) higher in the group with open wound defects, than in the group with membranes (р = 0.036), and 8 times higher, than in the control group (р = 0.013).
On day 12 of the study, large foci of dense fibrous connective tissue were found in the group with no wound covering (fig. 1E). Its specific area was significantly (3.9 times) higher, than in the group with covering (р = 0.036). At the macroscopic level, this was reflected in the buccal area deformities with soft tissue loss; the scar, 4.5 mm in length, was determined at the wound defect site (fig. 1F). In the group with polymeric membranes, the scar length reached 1.5 mm, and soft tissue deformation was slight. In the group with membranes, the macrophage and fibroblast numerical density reached the control values. In the group with no wound covering, these values were significantly (6 and 7.2 times) higher compared to the values of the intact group (р = 0.032, р = 0.021). In the group with no wound covering, fibroblasts still showed ultrastructural signs of high synthetic activity, while functionally inactive fibrocytes prevailed in the group with covering (fig. 1G). At the ultrastructural level, the well-developed rough endoplasmic reticulum, Golgi apparatus, and mitochondria were visible in macrophages, which indicated high synthetic activity. The CD68 and TGFβ1 expression was significantly higher in the group with open wound defects, than in the groups with coverings and intact mucosa: 2 and 3 times higher, respectively (р = 0.019, р = 0.025) (see Table) (fig. 1H).
DISCUSSION
During this study we assessed the major predictors of fibrous tissue development at the oral mucosal wound defect site. The following scheme of pathomorphogenesis can be drawn based on the study results and the literature review (fig. 2).
According to the scheme, extensive cell infiltration in the healing area was observed at the first stage of wound defect regeneration, in the inflammatory phase. A large cluster of macrophages, the numerical density of which was significantly higher, than in the group with open wound defects, was revealed in the group, where biocompatible membranes were used. This indicator correlated with the increased macrophage CD68 marker expression in the group with polymeric membranes. At the ultra-microscopic level, the signs of increased synthetic activity could be noted in the group with covering. The welldeveloped synthetic apparatus was essential for the release of bioactive substances, which were also attractants for migration of fibroblasts to the wounded area [11]. We interpreted the data obtained as the fact of the inflammatory phase transition to the proliferative phase, since it is macrophages that are responsible for targeted migration of fibroblasts due to the release of vascular endothelial growth factor (VEGF), platelet-derived growth factor (PDGF), and TGFβ, contributing to angiogenesis, collagen production, and re-epithelization [12].
Furthermore, the numerical density of fibroblast type cells was significantly increased in the group with covering. This also suggested that the major processes observed in the wound shifted from inflammatory response to connective tissue formation in the group with wound covering.
However, to properly interpret these indicators, it was necessary to analyze their dynamic changes, since preservation of extensive infiltration, especially with macrophages, at the later stages of wound regeneration could be a prerequisite for the development of fibrosis. That is exactly what we saw in the group with no wound covering, where the macrophage numerical density was significantly higher, than in the group with wound covering, on day 7 of the study and did not reach the control values on day 12 of the study. The fibroblast numerical density, on the contrary, was significantly lower in the group with no covering on day 7. On day 12, the fibroblast numerical density increased relative to the intact mucosa and the group with covering. Such a relationship between macrophages and fibroblasts in the group with open wounds indicated a prolonged inflammation that contributed to further development of fibrosis [11]. In the group, where biocompatible piezoelectric membranes was used, the numerical density of the studied cell populations recovered to the control values on day 12 of the study, which was considered a positive prognostic sign.
The TGFβ1 release by the cells is a primary trigger for the wound regeneration initiation. In this regard, we observed increased TGFβ1 expression on day 3 of the study in both experimental groups [12]. However, TGFβ1 expression was significantly higher in the group with no wound covering, than in the group with biocompatible membranes; the expression reached its maximum on day 7 of the study and did not return to the control values on day 12 of the study. This marker is released by a number of cells at the wound defect site: platelets, macrophages, fibroblasts, keratinocytes, etc. [4]. And this specific marker plays a critically important role in the shift of regeneration stages, since it has an effect on cooperation of macrophages and fibroblasts at the wound site [5]. Furthermore, it is reported that not only different cell types respond differently to TGFβ1, but also the same cells can show contradictory responses depending on the experimental conditions [5].
The TGFβ1 expression increase reported on day 7 of the study mediated preservation of extensive infiltration with the synthetically active fibroblasts and macrophages at the wound site on day 12 of the study in the group with open wound defects. At the same time, their numerical density in the group with covering returned to the control values in the final phase of wound regeneration against the background of lower H-score values for TGFβ1. Moreover, the fibroblast type cells were represented mainly by the synthetically inactive fibrocytes, which indicated termination of connective tissue development at the wound defect site in the group with polymeric membranes. In the group with open wound defects, the synthetically active fibroblasts were mostly found at the wound site on day 12 of the study. Proliferation, migration, and differentiation of fibroblasts were observed under exposure to TGFβ1, while aberrant signal transmission from TGFβ1 was associated with activation of the mechanism underlying the scar tissue formation [12]. The scar tissue formation was related to the abnormal, disturbed wound healing process associated with chronic inflammation [13] occurring against the background of increased TGFβ1 expression, which represented an important phase of chronic wound regeneration [14]. Thus, the TGFβ1 expression increase can be considered an important pro-fibrotic marker [15].
That is why dense fibrous connective tissue constituting the basis for fibrotic changes was formed in the group with open wound defects during interaction between fibroblasts and macrophages under exposure to TGFβ1, which was indicated by the presence of the scar, 4.5 mm in length, and soft tissue loss at the macroscopic level.
The polymeric piezoelectric membranes made of vinylidene fluoride/tetrafluoroethylene copolymer by electrospinning represent the most recent creation of the laboratory of hybrid biomaterials of the Tomsk Polytechnic University. There are no earlier studies focused on assessing the effectiveness of using the analogues with similar physical and chemical properties for the oral mucosal wound defect regeneration in global practice. Based on this study we assume that piezoelectric properties of biocompatible polymeric membranes contributed to the TGFβ1 expression decrease, which resulted in inhibition of TGFβ1 effects on the activity of fibroblasts and macrophages. Consequently, loose fibrous connective tissue was formed, while dense fibrous tissue formation and, as a result, scarring was reported in the group with open wounds. This hypothesis is confirmed by the data of the comparative study of piezoelectric and dielectric coverings in regeneration of oral mucosal wounds, during which smaller specific area of scar tissue was reported for the group with piezoelectric covering [7].
CONCLUSIONS
The study revealed positive effects of biocompatible piezoelectric membranes on prevention of fibrosis during wound regeneration, which was confirmed by the analysis of interplay between the macrophage and fibroblast type cells and expression of the CD68 and TGFβ1 prognostic markers. The use of polymeric biocompatible membranes in dental practice will make it possible to reduce the risk of postoperative complications in the form of oral soft tissue deformation in the future. It is planned to further assess the effects of covering membranes on the oral mucosal regeneration in order to improve the membrane properties.