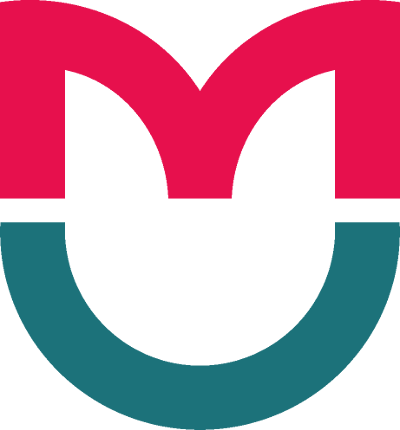
This article is an open access article distributed under the terms and conditions of the Creative Commons Attribution license (CC BY).
ORIGINAL RESEARCH
Transcription profile in preoperative aromatase inhibitor response test in breast cancer patients
Kulakov National Medical Research Center for Obstetrics, Gynecology and Perinatology, Moscow, Russia
Correspondence should be addressed: Olga Vladimirovna Burmenskaya
Akademika Oparina, 4, Moscow, 117997, Russia; ur.liam@aksnemruob
Funding: the study was partially supported under the State Task "Personification of adjuvant treatment of patients with luminal HER2-negative subtypes of breast cancer enabled by a preoperative hormone sensitivity test" (state registry number in the Unified State R&D Efforts Registration System (EGISU NIOKTR): 124020600031-3).
Author contribution: VV Rodionov — study conceptualization and design; MV Rodionova, VV Kometova — material collection and processing; DYu Trofimov, OV Burmenskaya — PCR testing; OV Burmenskaya — analysis of the results, statistical processing of the data, manuscript authoring; VV Rodionov, DYu Trofimov — editing.
Compliance with ethical standards: the study was approved by the Committee on Biomedical Ethics of V.I. Kulakov National Medical Research Center for Obstetrics, Gynecology and Perinatology (Minutes #03 of March 21, 2024). All patients signed an informed consent to participate in the study.
Identification of five main molecular subtypes of breast tumors and formalization of differential approach to the treatment of breast cancer (BC) patients constituted a real breakthrough in oncology. Tumors expressing estrogen (ESR) and progesterone (PGR) receptors are of luminal subtypes; as a rule, they are susceptible to hormone therapy.
In postmenopausal women, estrogens are formed mainly with the participation of the aromatase enzyme, which converts androgens synthesized in the adrenal glands and adipose tissue (testosterone and androstenedione) into estradiol and estrone. Therefore, in the context of treatment of breast tumors of luminal subtypes, this cohort of patients receives selective aromatase inhibitors (letrozole, anastrozole), which block synthesis of estrogens through highly specific competitive binding to the aromatase subunit encoded by the cytochrome P450 CYP19A1 gene.
Tumors expressing estrogen receptor are prevalent among molecular subtypes of BC [1, 2]. However, even within this large group, there are significant differences in the features of the course of the disease and response to hormone therapy, which necessitates study of all learnable biological parameters of a malignant neoplasm with the aim to devise the optimal treatment tactics.
Preoperative hormone response test is one of the tools designed for the purpose. This test enables prescription of a short-term (2–4 weeks) hormonal drugs course before surgery. Evaluation of the dynamics of tumor proliferation by monitoring changes of the level of Ki67 and expression of hormonal receptors allows predicting clinical response to and effectiveness of endocrinotherapy in vivo, and consequently free some patients from unnecessary highly toxic chemotherapy. The recommendation to do hormone therapy testing was included in the St. Gallen International Consensus Guidelines for treatment of early breast cancer issued in 2021, and were kept in the 2023 edition of the paper [3, 4]; Russian breast cancer treatment guidelines contain similar recommendations [5, 6].
BC-specific transcription panels of genes involved in oncogenesis, such as Oncotype DX, PAM50, Mammaprint, and Russian products, can be a useful tool in evaluating the effectiveness of therapy [7, 8].
In this connection, this study aimed to investigate the changes in gene expression caused by the preoperative aromatase inhibitor response test in postmenopausal women with ESR+/HER2-negative breast cancer.
METHODS
The study involved 100 breast cancer patients treated at the Department of Breast Pathology of Academician V.I. Kulakov National Medical Research Center for Obstetrics, Gynecology and Perinatology, from January, 2019 to August, 2024. We studied samples trephine biopsied from each participant before the hormone response test, and samples taken during surgery.
The inclusion criteria were: invasive breast cancer, luminal Her2-negative tumor subtype, postmenopausal age, preoperative test for tumor sensitivity to aromatase inhibitors. Exclusion criteria: inadequate quality of trephine biopsied samples (unfit for molecular genetic studies).
The preoperative hormone sensitivity test implied taking aromatase inhibitors (letrozole 2.5 mg or anastrozole 1 mg) once a day for 2–4 weeks.
We did histological and immunohistochemical studies of the biopsy and intrasurgery samples, which were taken within 30 minutes after tumor removal and preserved for 48 hours in neutral buffered formalin. After preservation, the samples were processed in an automatic LOGOS microwave histoprocessor (Milestone, Italy). The sections, 4–5 microns thick, were stained with hematoxylin and eosin. Microscopic preparations were subjected to survey microscopy enabled by an Olympus BX46 light microscope (Olympus Corp., Japan). For immunohistochemical diagnostics, we used a BenchMark ULTRA system (Ventana, Roche) employing antibodies to estrogen receptors (ER, clone SP1, VENTANA), progesterone (PgR, clone 1E2, VENTANA), to Ki67 (clone MIB–1, VENTANA), to the oncoprotein of the human epidermal growth factor type 2 gene (c–erbB2).
For molecular genetic studies, we used a transcription panel that included 45 target genes (ESR1, PGR, AR, ERBB2, GRB7, EGFR, FGFR4, MKI67, MYBL2, CCNB1, AURKA, BIRC5, MYC, CCND1, CCNE1, CDKN2A, KIF14, PPP2R2A, PTTG1, SFRP1, TMEM45B, TMEM45A, TPX2, MMP11, CTSL2, EMSY, PAK1, ANLN, BCL2, BAG1, PTEN, TYMS, EXO1, UBE2T, TPT1, SCGB2A2, KRT5, MIA, GATA3, FOXA1, ZNF703, NAT1 CD68, TRAС, CD274/PD-L1) and three reference genes (B2M, GUSB, HPRT1). Two to three paraffin sections 4-5 microns thick were placed in dry plastic 1.5 ml tubes. The material was selected and prepared by a pathomorphologist, with the results of the pathomorphological study factored in.
Total RNA was isolated after pretreatment of samples with proteinase K (PREP-PK kit; DNA-Technology LLC, Russia), then we used a PREP-NA PLUS kit (DNA-Technology LLC, Russia) to isolate nucleic acids in alcohol deposits. The resulting RNA preparations were immediately used to set up a reverse transcription reaction with a mixture of oligonucleotides specific to each gene. The reverse transcription reaction was maintained for 30 minutes at 40 °C, followed by a 10-minute reverse transcriptase inactivation at 95 °C.
The transcription profile of the genes was determined by multiplex Real-time reverse transcription polymerase chain reaction (RT-qPCR). The PCR was enabled by DTprime detecting amplifiers (DNA-Technology LLC, Russia); the program was as follows: 94 °C for 5 minutes; five cycles of 94 °C for 30 s — 64 °C for 15 s; then 45 cycles of 94 °C for 10 s — 64 °C 15 s, and storage.
The reaction mixtures included oligonucleotides specific to mRNA, not amplifying from genomic DNA template, flanking amplicons measuring up to 100–120 bp, which is important when working with DNA after preservation in formalin. The amplification products were detected at the primer annealing temperature by using fluorescently labeled TaqMan probes (Fam, Cy5).
After amplification, we calculated the abundance of transcript by comparing indicator cycles (∆Ct) with the norm relative to the reference genes B2M, GUSB, HPRT1. The data are given in relative units (RU) on the ln scale.
To improve perception of the results of the study, we calculated the ratio (R) of expression before and after therapy: R = Eb/Ea, where Eb is the normalized expression level before therapy, and Ea is the normalized expression level after therapy. R > 1 was regarded as dropping expression, R < 1 as rising expression.
Statistical methods
The results obtained are presented as Me [1Q–3Q] (median, 1st and 3rd quartiles). Comparing the transcription profiles of genes before and after aromatase inhibitor therapy, we employed the Wilcoxon signed-rank test. The values were considered statistically significant at p < 0.01. The calculation was done in SPSS 17 statistical processing program (IBM, USA).
RESULTS
The study included 100 BC patients aged 49–84 years (63.3 [51–79]). Table tab. 1 gives the pathomorphological characteristics of their tumors.
In 75% of cases, the tumor was smaller than 2 cm. In 2/3 (76%) of the patients, there were no metastases to the regional lymph nodes diagnosed. No cases involved distant metastases.
The nonspecific variant was the prevailing one: 65 cases (65%). Eighteen percent of samples were from low grade tumors (G1), 74% from intermediate grade cancers (G2), and 8% from high grade neoplasms (G3).
Using the IHC test, we established that 44 (44%) patients suffered a luminal A subtype of BC, and 56 (56%) participants had luminal B HER2-negative subtype of the tumor.
Aromatase inhibitors taken preoperatively as a hormone sensitivity test caused significant changes in the mRNA expression of 37 genes, as registered in the studied tumor samples, with the expression level dropping for 35 genes (ESR1, PGR, AR, ERBB2, FGFR4, MKI67, MYBL2, CCNB1, AURKA, BIRC5, CCND1, CCNE1, CDKN2A, KIF14, PPP2R2A, PTTG1, TMEM45B, TPX2, ANLN, MMP11, CTSL2, EMSY, PAK1, BCL2, BAG1, PTEN, TYMS, EXO1, UBE2T, NAT1, SCGB2A2, GATA3, FOXA1, ZNF703, CD274/PD-L1), and rising for two SFRP1 and KRT5 genes (tab. 2).
Figure figure shows the results for five proliferation markers, estrogen and progesterone receptors, the levels of which decreased after treatment.
A statistically significant drop of expression was registered in the group of markers of proliferation and regulation of the cell cycle. The most pronounced changes were noted for the MYBL2, AURKA, PTTG1, TPX2, and ANLN genes, with the expression decreasing 3.5-5.5 times in 96–97% of women.
We have also registered significant expression drops:
- in the group of markers of cell migration and cytoskeleton organization, with the most pronounced decrease observed for MMP11, the expression level of which went down 3.4 times on average (interquartile range 1.9–6.3) in 94% of women;
- in the group of DNA repair markers, with the most pronounced decrease observed for EXO1, the expression level of which went down 6.4 times on average (interquartile range 3.1–15.3) in 96% of women;
- in the group of transcription factors, with the most pronounced decrease observed for ZNF703, the expression level of which went down 6.6 times on average (interquartile range 2.9–12.6) in 97% of women;
- in the group of hormonal receptors, with a 2.3-fold (average) decrease of the expression of the ESR1 mRNA gene in 84% of women, and the most pronounced decrease observed for the PGR and AR genes, the expression of which dropped more than 2-fold in over 90% of the participants;
- in the ungrouped markers of the ubiquitination gene of UBE2T proteins and the arylamine-N-acetyltransferase NAT1 gene (more than 90% of women).
A slightly less impressive but statistically significant decrease in expression was observed for apoptosis markers (BCL2, BAG), growth factor receptors (FGFR4, ERBB2), secretory protein mammoglobin SCGB2A2, and PTEN.
The level of expression increased significantly for the marker of epithelial cell differentiation of cytokeratin KRT5 and suppressor SFRP1: this was observed in more than half of the patients, 67% and 57% of cases, respectively.
DISCUSSION
Estrogen-positive or luminal breast tumors account for up to 70–75% of all BC cases [1, 2], and ESR1-dependent signaling pathways regulated by estrogens play one of the key roles in tumor progression.
The theory by E.V. Jensen, who described the model of action of the hormone, laid the foundation of the current understanding of how steroid hormones act. Within the framework of this model, a steroid hormone molecule, having penetrated into the target cell, binds to a specific receptor, and the resulting hormone-receptor complex is translocated into the cell nucleus, where it interacts with specific DNA sequences and changes the expression of the corresponding genes [9].
The ESR1 receptor is an estrogen-activated transcription factor. As such, it regulates the expression of genes involved in cell cycles, proliferation and apoptosis. Activation of ESR1 triggers the expression of MYC, Cyclin D1, FOXM1, GREB1, BCL2 or amphiregulin, IGF-1 and CXCL12, which possess oncogenic potential [2]. It is believed that ligand-activated ESR1 binds to estrogen responsive elements (ERE) in promoters of target genes, and it can also interact with transcription factors, i.e., activator protein 1 (AP1) and specific protein 1 (SP1). Thus, this genomic action regulates the transcription of hundreds of target genes involved in cell growth and differentiation [2, 10].
ESR1 can also embed in caveoles, special recesses in the cytoplasmic membrane of cells, and interact with G proteins, thus participating in the production of secondary messengers (cAMP) and stimulation of various signaling pathways, like PI3K/ AKT or Ras/MAPK. This non-genomic activity of ERα eventually leads to the activation of transcription factors involved in the regulation of cell proliferation and survival [2, 10].
Therefore, there is no doubt that a complete lack or smaller amounts of the ESR1 ligand (estrogens) associated with aromatase inhibitor therapy translate into an impressive drop of the level of expression of mRNA of a large number of genes. The results we received confirm a significant slowdown of mRNA expression for transcription factors GATA3, FOXA1, ZNF703, apoptosis BCL2, BAG1, proliferation and cell cycle regulation (MKI67, MYBL2, CCNB1, AURKA, BIRC5, CCND1, CCNE1, CDKN2A, KIF14, PPP2R2A, PTTG1, TMEM45B, ANLN, TPX2). Deterioration of proliferative activity also decelerates the expression of markers of replication and control of DNA repair (TYMS, EXO1), cytoskeleton and cell migration markers (MMP11, CTSL2, EMSY, PAK), and the process of ubiquitination of UBE2T proteins.
Interestingly, another study that focused on atypical breast hyperplasia showed an increase in the expression of mRNA genes from our panel, namely, ESR1, AR, epidermal growth factor receptor ERBB2, transcription factors FOXA1, GATA3, ZNF703, and decreasing amounts of cytokeratin KRT5 and tumor suppressor SFRP1 [11]. The authors of that study associated these changes with activation of signaling pathways involved in oncogenesis.
In our study, aromatase inhibitor therapy caused a slowdown of the expression of mRNA of the ESR1, AR, FOXA1, GATA3, ZNF703, ERBB2 genes, and acceleration of the expression of mRNA of the KRT5 and SFRP1 genes, which may indicate a positive effect the said therapy has on signaling pathways that participate in oncogenesis.
We registered significant acceleration of expression only for two markers, KRT5 and SFRP1. High level of expression of the cytokeratin gene KRT5 is characteristic of the breast basal epithelium. Luminal epithelium expresses cytokeratins KRT8 and KRT18 [2].
Currently, it is generally accepted that the main physiological regulator of the expression of nuclear receptors in the body is the concentration of circulating steroid hormones. Estrogens boost synthesis of their own receptors as well as receptors of progesterone androgen [10]. Therefore, the attenuating effect of the therapy on the expression of mRNA of ESR1, PGR, and AR was predictable. It should be noted that initially, we noted decreasing expression of the progesterone receptor in the first place, and the slowdown of expression of that for ESR1 was registered secondary to that.
The mRNA expression of the PGR gene is directly regulated by the activated estrogen receptor. Some authors even consider PGR to be an indicator of the ESR1 functionality [12]. Therefore, with dropping estrogens and the transcriptional activity of the ESR1 gene in the background, the expression of the PGR gene's mRNA decreases, too.
CONCLUSIONS
Aromatase inhibitors taken preoperatively in the context of the hormone sensitivity test cause significant changes in the mRNA expression of 37 genes, with that for 35 genes (MKI67, MYBL2, CCNB1, AURKA, BIRC5, CCND1, CCNE1, CDKN2A, KIF14, PPP2R2A, PTTG1, TMEM45B, TPX2, ANLN, ESR1, PGR, AR, ERBB2, FGFR4, MMP11, CTSL2, EMSY, PAK1, BCL2, BAG1, GATA3, FOXA1, ZNF703, TYMS, EXO1, PTEN, UBE2T, NAT1, SCGB2A2, CD274) dropping, and for 2 genes (KRT5 and SFRP1) growing. The results of this study can be used in the development of the hormone sensitivity test effectiveness assessment method and personification of adjuvant systemic treatment for breast cancer patients.