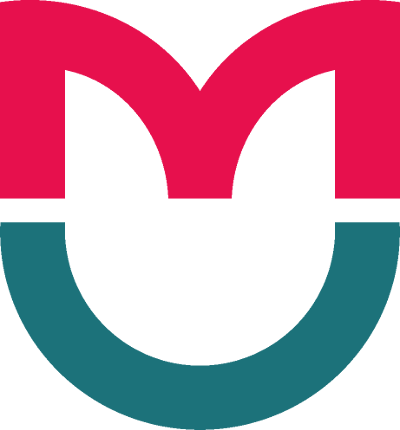
This article is an open access article distributed under the terms and conditions of the Creative Commons Attribution license (CC BY).
ORIGINAL RESEARCH
Complex antibacterial action of enzymes acting on Staphylococcus aureus biofilms
1 Sirius University of Science and Technology, Sirius, Sochi, Russia
2 Institute of Biochemistry and Physiology of Microorganisms, Russian Academy of Sciences, Pushchino, Russia
Correspondence should be addressed: Maxim O. Nagornykh
Prospekt Nauki, 5, Pushchino, 142290, Russia; moc.liamg@rennabred
Funding: the study was supported by the program of the Ministry of Science and Higher Education of the Russian Federation (agreement No. 075-10-2021-113, unique project ID: RF----193021X0001).
Author contribution: Zagoskin AA, Mirzoyan RA — creating genetic constructs, сhromatographic purification of recombinant proteins; Rezvykh LF — creating genetic constructs; Zakharova MV, Mubarakshina EK — selection of condition for recombinant protein production, experiments on production involving various E. coli strains; Nagornykh MO — study concept, genetic construct design, manuscript writing; Ivanov RA — general management.