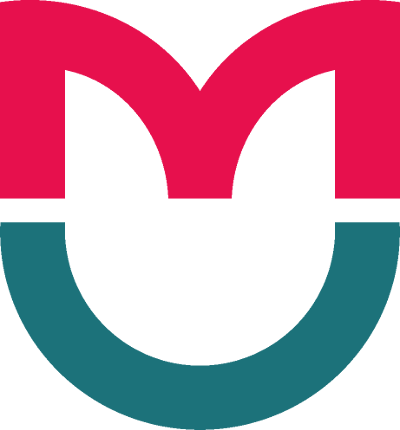
This article is an open access article distributed under the terms and conditions of the Creative Commons Attribution license (CC BY).
ORIGINAL RESEARCH
Assessment of the effect of hypothermia after simulated hypoxic ischemic encephalopathy based on blood metabolome
1 Kulakov National Medical Research Center for Obstetrics, Gynecology and Perinatology, Moscow, Russia
2 Belozersky Institute Of Physico-Chemical Biology, Moscow State University, Moscow, Russia
Correspondence should be addressed: Chupalav M. Eldarov
Akademika Oparina, 4, Moscow, 117997, Russia; ur.val4puhc@em
Funding: the study was supported by the Russian Federation on behalf of the Russian Science Foundation (grant No. 22-15-00454).
Author contribution: Eldarov CM — sample preparation, conducting HPLC-MS/MS, chromatography–mass spectrometry data processing, manuscript writing and editing; Starodubtseva NL — metabolomic data analysis, statistical analysis; manuscript writing and editing; Shevtsova YuA — conducting the model experiment, sample acquisition and characterization, discussing the results, manuscript writing and editing; Goryunov KV — conducting the model experiment, sample acquisition and characterization, manuscript writing and editing; Ionov OV — study planning, manuscript editing; Silachev DN — study planning, discussing the results, manuscript writing and editing.
Compliance with ethical standards: the study was approved by the Ethics Committee of the Belozersky Institute Of Physico-Chemical Biology, Moscow State University (protocol No. 8/22 dated 12 June 2022). The study was compliant with the standards of the Ethics Committee of the Belozersky Institute Of Physico- Chemical Biology, Moscow State University, and the Declaration of Helsinki (1964).
Hypoxic ischemic encephalopathy (HIE) is a severe neurological condition resulting from the lack of oxygen and insufficient blood supply to the brain of a newborn before and during labor. The rate of moderate-to-severe HIE forms is 1–8 cases per 1000 live births in the countries with advanced medicine, it shows no downward trend. HIE causes 6–9% of deaths among newborns and 21–23% of deaths among full-term babies, and 25% affected individuals develop severe neurological disorders (cerebral palsy, epilepsy, seizures, and developmental delay) [1].
The timely diagnosis and accurate determination of HIE severity are crucial for improvement of outcomes and the children’s quality of life [2]. Therapeutic hypothermia (TH) is the key treatment method for moderate-to-severe HIE widely used in intensive care units. This approach significantly decreases the risk of fatality and contributes to improvement of long-term outcomes in infants with HIE [3].
The body or head temperature decrease occurring during TH slows down metabolic processes, reduces the neurons’ oxygen demands, as well as the levels of oxidative stress and its mediators, i.e. glutamate and free radicals. Furthermore, inflammatory response is suppressed and apoptosis is prevented, which mitigates the effects of primary hypoxic ischemic stroke [4]. However, it is necessary to start TH within the first six hours of life (acute phase of hypoxic ischemic injury), before activation of secondary injury mechanisms, such as inflammation and apoptosis, for optimal results [5]. Unfortunately, despite proven efficacy of TH, about 20% of newborns with moderate or severe HIE do not receive treatment they need due to delayed diagnosis [6]. TH has a number of limitations and the use of TH can be dangerous in cases of erroneous diagnosis [7]. This emphasizes the importance of rapid intervention and improvement of diagnostic procedures in neonatology, including the analysis of biochemical markers associated with brain damage.
The search for HIE markers is hampered by both low prevalence of the disorder in the population and difficulty distinguishing from concomitant disorders, such as sepsis and congenital metabolic disorders [2, 8]. The use of noninvasive (urine, feces) and minimally invasive (blood, dried blood spots) samples makes it possible to effectively assess the dynamic changes in molecular composition of body fluids. The samples remaining after the routine procedures can be used for further testing without any additional interventions.
Assessment under the controlled conditions in model systems is performed to identify the markers associated exclusively with brain damage [8, 9]. The Rice–Vannucci model proposed in 1981 is most often used as a pre-clinical model for HIE assessment; it is used in laboratory settings all over the world over the last four decades [9, 10].
The omics technologies, including genomics, transcriptomics, proteomics, and metabolomics, contributed greatly to clarification of the pathophysiological mechanisms underlying HIE. These processes include alterations in energy metabolism of brain cells, oxidative stress, disturbed metabolism of neurotransmitters and hormones, as well as apoptosis of neurons and activation of neuroprotective (defensive) mechanisms [5, :lit_8,; 11–13]. The analysis of metabolic alterations in dried blood spots by liquid chromatography–mass spectrometry (HPLC-MS)
is a promising strategy for improving the diagnosis and prediction of HIE in newborns. Together with multivariate analysis techniques, HPLC-MS allows one to isolate the whole patterns of biomolecules specific for hypoxic ischemic (HI) brain injury [5].
The study aimed to clarify the molecular mechanisms and identify the brain damage markers triggered in hypothermia during recovery after the HI brain injury using the modified Rice–Vannucci model by untargeted metabolomics (HPLC-MS) of dried blood spots in negative mode.
METHODS
HIE simulation
The animal handling protocol was approved by the local Ethics Committee in accordance with the principles of the Federation of European Laboratory Animal Science Associations (FELASA). The experiments involved outbred white rats obtained from the animal facility of the Belozersky Institute Of Physico-Chemical Biology, MSU.
The modified Rice–Vannucci model was used to simulate HI brain injury [26–27]. The 7-day-old male and female pups were anesthetized with 1% isoflurane, after which the left carotid artery was surgically isolated and subjected to electrocoagulation. After 1.5 h the pups were placed in the CO2 incubator (Binder; Tuttlingen, Germany) with the gas mixture containing 8% of oxygen and 92% of nitrogen at 37 °C for 2 h.
Therapeutic hypothermia
Rats were randomly distributed into normothermic and hypothermic groups immediately after the end of HI for 6 h, then the dried blood spot samples were obtained. The experiment involved three groups of animals: control group (n = 13), rats with HIE and subsequent 6-h hypothermia (n = 16), rats with HIE and subsequent 6-h normothermia (n = 14). Normothermia was maintained at the level of 37 °C, hypothermic recovery took place at 30 °C. Pups were placed in an open container in a water bath in order to maintain the specified temperature.
Dried blood spot collection
The pups’ blood was collected from the subclavian artery 6 h after normothermia/hypothermia, 40 μL of blood were applied to the PerkinElmer 226 filter paper test sheet. When applying blood to the sheet, we made sure that the tip of the syringe did not touch the paper. Once dry, blood spots were tested for suitability for analysis (the area of the paper impregnated with blood had to be at least 3 mm in diameter). The samples were considered unsuitable, when the blood spot area was insufficient or, in case of small samples, when blood didn't soak through the paper. The spots were also considered to be unsuitable, when there were microclots in the sample suggesting patchy distribution of the spot across the paper.
Sample preparation for HPLC-MS
Metabolites were extracted from the dried blood spots by the Folch’s method [14]. Each sample (three circular fragments of the dried blood spot, 5 mm in diameter each) were added 480 μL of the chloroform and methanol mixture (2 : 1 ratio) and 250 μL of water. The extraction mixture was vortexed for 10 min, and then centrifuged at 15,000 g for 10 min. The organic phase (150 mL) from the lower part of the Eppendorf tube was transferred to a separate tube. This extraction phase was repeated with adding one more portion of the chloroform–methanol mixture. A total of 100 μL of the organic phase after the second centrifugation was mixed with the previously collected organic phase. The resulting mixture was dried under a nitrogen stream at room temperature, and then dissolved in 100 mL of the acetonitrile and isopropanol mixture (1 : 1 ratio) again.
HPLC-MS analysis and identification of substances
Further analysis was performed by HPLC-MS. The reverse-phased Atlantis T3 C18 column (particle size 3 μm, length 15 cm, inner diameter 1 mm; Waters, Milford, Massachusetts, USA) and the Ultimate 3000 Nano LC chromatography system (Thermo Scientific; Waltham, Massachusetts, USA) were used for chromatographic separation of the complex mixture of substances [30–31]. The mobile phase A consisted of the ACN : H20 mixture (60 : 40), and the mobile phase B consisted of IPA : ACN : H2O (90 : 8 : 2). Both phases contained modifiers (0.1% of formic acid and 5 mm of ammonium formate). Elution was performed with the mobile phase B gradient at the flow rate of 40 μL/min: 0–0.5 min at 10%, then the 20-minute gradient from 10% to 99%. After that the 10-minute washing with the 99% phase B was performed, then the phase was returned to its initial concentration (10% of phase B) within 1 min, and the column was equilibrated for 3 min. The total chromatographic run time per sample was 34.5 min at the flow rate of 40 μL/min and the column temperature of 50 °С. Metabolites were determined using the Bruker MaXis Impact hybrid quadrupole time-of-flight mass spectrometer (Bruker Daltoniks; Bremen, Germany), with two repeats per sample and the following device settings: capillary voltage 4500 V; nebulizer pressure 0.6 Pa, drying gas flow rate 5.0 L/min at 200 °C. Mass spectra were acquired at the 50,000 resolution within the range of 50–1700 m/z, with negative polarity.
Peak detection and grouping, as well as retention time adjustment were performed using the xcms software package. The Centwave algorithm was used to detect peaks with certain parameters: the maximum m/z deviation was 15 ppm, and the peak width was 10–45 s. Peaks were grouped by the Peak Density method with the default parameters [15–16]. Metabolites were identified based on the Human Metabolome database (https://www.hmdb.ca).
Statistical methods
To identify and visualize the differences between groups, we conducted multivariate analysis by the partial least squares method (sPLS-DA) for multiple groups and the orthogonal partial least squares method (OPLS-DA) for pairwise comparison implemented in the Metaboanalyst 6.0.0 package (https:// metaboanalyst.ca). Significance of intergroup differences in relative concentrations (average integrated peak areas) of certain metabolites was estimated using the t-test, and p-value below 0.05 was considered to be significant. As an additional criterion for identification of potential biomarkers, the fold change was limited to the value of 1.5. The analysis of enrichment pathways was performed using the over-representation analysis function of the Metaboanalyst 6.0.0 package.
RESULTS
The analysis of mass spectra conducted in the negative ion mode allowed us to identify 3425 molecular ions. To assess the differences between the groups of animals, we used the multivariate partial least squares method (sPLS-DA). According to the results provided in fig. 1, the groups can be clearly distinguished, which is indicative of significant metabolic alterations typical for each group. This highlights that there are specific molecular differences underlying the dividing factors (fig. 2).
To search for potential biomarkers, we conducted univariate statistical analysis of the normothermia-hypothermia pair (fig. 2). A total of 55 molecular ions were found, among which 27 showed the concentration increase in the samples of animals subjected to hypothermia relative to the normothermic group. At the same time, concentrations of 28 molecular ions were decreased. We took into account significant differences in concentrations only (at least 1.5-fold with the significance level p < 0.05). The compounds detected were identified using the LIPID MAPS and HMDB databases; the results are provided in tab. 1. The data obtained show significant changes in metabolism of phosphoethanolamines and phosphatidic acids in hypothermia compared to normothermia, while the fatty acid levels remain relatively stable. Extensive analysis was conducted using the multivariate statistical method (orthogonal partial least squares (OPLS)), which allowed us to clearly delineate normothermic and hypothermic groups. The findings confirm that there are significant metabolic differences between these physiological states (fig. 3). We selected molecular ions (top 200) that made the greatest contribution to delineation of the groups (VIP score >1) based on the analysis results; identification and search for the most involved metabolic pathways in the SMPDB database were performed for these ions. The changes in activity of biosynthesis pathways of bile acids, ubiquinone and plasmalogens, as well as in metabolism of a number of fatty acids, estrogens and androgens associated with hypothermia were identified (tab. 2).
DISCUSSION
HIE is a major cause of neurological disorders and neonatal mortality. Ischemia followed by hypoxia leads to neuronal death within 12–36 h, which makes the timely diagnosis of HIE and estimation of injury severity one of the factors important for successful prediction of outcomes and selection of adequate therapy. MRI and aEEG of the brain that allow one to somehow estimate physical and functional brain damage represent the standard methods to diagnose HIE. However, these methods have limited informative value: thus, MRI is often impossible in newborns with severe conditions, it is associated with the need for sedation and is conducted 24 h or more after birth, while aEEG has insufficient sensitivity for early diagnosis. Furthermore, these methods involve no quantitative markers allowing one to reliably determine HIE severity. Therefore, prediction of outcomes remains a challenging task.
At the same time, therapeutic hypothermia, during which the newborn’s head is cooled to 32–35 °С for 48–72 h, represents one of the main HIE therapy methods. To ensure the neuroprotective effect, it is necessary to start the procedure within the first 6 h of the newborn’s life. Assessment of blood biomarkers represents one of the HIE early diagnosis methods, since HIE is associated with not only brain damage, but also damage to the heart, liver, and other organs causing alterations of their metabolism. When assessing the dynamic changes in blood biomarkers, it is possible to correlate the changes with the developing injury and body’s response, later adapting the therapy received by the patient to these changes. The use of changes in pro-inflammatory and other blood markers for the diagnosis of HIE has been previously reported. While venous blood collection for further testing is a gold standard in medicine, the use of this method is associated with a number of nuisance due to its invasive nature, the need to teach personnel, and difficulty ensuring conditions for storage and transportation of the samples collected. This is particularly true when working with newborns having severe disorders. In this study we used dried blood spots to assess metabolome. This method representing one of blood microsampling methods is becoming increasingly common due to low invasiveness, these physiological states (fig. 3). We selected molecular ions (top 200) that made the greatest contribution to delineation of the groups (VIP score >1) based on the analysis results; identification and search for the most involved metabolic pathways in the SMPDB database were performed for these ions. The changes in activity of biosynthesis pathways of bile acids, ubiquinone and plasmalogens, as well as in metabolism of a number of fatty acids, estrogens and androgens associated with hypothermia were identified (tab. 2).
DISCUSSION
HIE is a major cause of neurological disorders and neonatal mortality. Ischemia followed by hypoxia leads to neuronal death within 12–36 h, which makes the timely diagnosis of HIE and estimation of injury severity one of the factors important for successful prediction of outcomes and selection of adequate therapy. MRI and aEEG of the brain that allow one to somehow estimate physical and functional brain damage represent the standard methods to diagnose HIE. However, these methods have limited informative value: thus, MRI is often impossible in newborns with severe conditions, it is associated with the need for sedation and is conducted 24 h or more after birth, while aEEG has insufficient sensitivity for early diagnosis. Furthermore, these methods involve no quantitative markers allowing one to reliably determine HIE severity. Therefore, prediction of outcomes remains a challenging task.
At the same time, therapeutic hypothermia, during which the newborn’s head is cooled to 32–35 °С for 48–72 h, represents one of the main HIE therapy methods. To ensure the neuroprotective effect, it is necessary to start the procedure within the first 6 h of the newborn’s life. Assessment of blood biomarkers represents one of the HIE early diagnosis methods, since HIE is associated with not only brain damage, but also damage to the heart, liver, and other organs causing alterations of their metabolism. When assessing the dynamic changes in blood biomarkers, it is possible to correlate the changes with the developing injury and body’s response, later adapting the therapy received by the patient to these changes. The use of changes in pro-inflammatory and other blood markers for the diagnosis of HIE has been previously reported. While venous blood collection for further testing is a gold standard in medicine, the use of this method is associated with a number of nuisance due to its invasive nature, the need to teach personnel, and difficulty ensuring conditions for storage and transportation of the samples collected. This is particularly true when working with newborns having severe disorders. In this study we used dried blood spots to assess metabolome. This method representing one of blood microsampling methods is becoming increasingly common due to low invasiveness, protocol simplicity, and easy storage of the acquired material collections. To use the method, it is important to estimate the effectiveness of its use for identification of early blood biomarkers.
The use of multivariate statistical analysis methods revealed significant differences both from the intact group and between normothermic and hypothermic groups (fig. 2), which reflects metabolic changes associated with HIE and HIE under conditions of normothermia. Pairwise statistical analysis with subsequent identification made it possible to reveal changes in the classes of phosphatidic acids (PA), phosphatidylinositols (PI) and phosphatidylethanolamines (PE) that belong to glycerophospholipids, as well as triglycerides and acylcarnitines. Furthermore, we revealed elevated PI levels. According to the literature data, brain ischemia leads to degradation of PI and diacylglycerols. Thus, expansion of the pool of PI and phosphatidylinositol diphosphate in the group with therapeutic hypothermia can indicate inhibition of its degradation in hypothermia, which has a neuroprotective effect. The hypothermic group also showed elevated levels of short-chain acylcarnitines (С6). It has been earlier shown in the cell-based model that short-chain acylcarnitines increase the expression of genes associated with antioxidant activity under conditions of oxidative stress [17]. As for phosphatidic acids, these are messengers and intermediates of other glycerolipids, such as PE and phosphatidylserines (PS) [18]. In the normothermic group we can see the decreased levels of intermediates in the form of phosphatidic acids, as well as the elevated PE and PS levels. In turn, PE are precursors of a number of endocannabinoids, the neuroprotective role of which is well known [19–22]. Thus, the use of therapeutic hypothermia led to the increase in concentrations of a number of metabolites associated with reduction of the oxidative stress resulting from ischemia-reperfusion and neuroprotectors, as well as their precursors, which definitely can reduce the overall damage resulting from HIE.
During the study we also analyzed the major metabolic pathways altered in hypothermia relative to the normothermic group. For that we identified molecular ions that had made the greatest contribution to the differences between groups in the OPLS-DA multivariate statistical analysis model (VIP, 200 most “weighty” molecular ions) and revealed metabolic pathways, these belonged to. The most involved pathways included synthesis of ubiquinone, bile acids, unsaturated fatty acids and plasmalogens (tab. 2). The presence of bile acids in the list can seem extraordinary. However, a number of studies have already shown that bile acids can cross the blood-brain barrier, performing the important functions of messengers and neuromodulators [23–24]. Bile acids have neuroprotective properties, these attenuate inflammation and the effects of oxidative stress, such as apoptosis [23–24], which is of special interest in the context of extensive oxidative stress resulting from ischemia-reperfusion associated with HIE [25–27]. The same is true for unsaturated fatty acids: both linoleic and alpha-linolenic fatty acids play an important role in body’s response and adaptation to acute ischemia through regulation of the neuronal signaling pathways by using oxylipins, the oxidized derivatives [28], show well-defined neuroprotective and even antidepressant properties [29]. As for ubiquinone, its role in protection against oxidative stress is widely known ([30], etc.).
CONCLUSIONS
Thus, our study has revealed a number of metabolome alterations when using therapeutic hypothermia, suggesting mitigation of brain damage caused by HIE, along with involvement of pathways responsible for synthesis of various neuroprotectors and neuromodulators. These findings confirm understanding of therapeutic hypothermia as an effective method for therapy of the HIE sequelae. The use of mass spectrometry makes it possible to obtain information about metabolic alterations as early as within the first hours after birth. At the same time, the targeted quantitative studies of the metabolites identified (glycerophospholipids, bile acids, etc.) in patients with HIE of varying severity can make it possible to develop an effective system for early classification of HIE severity and prediction of outcomes.