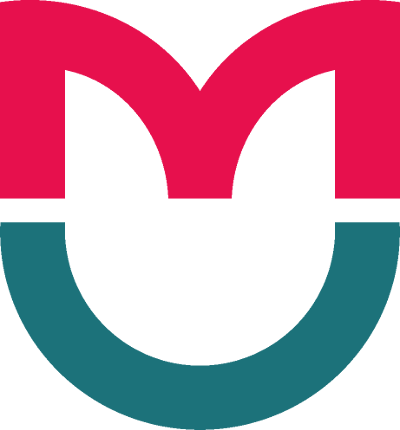
This article is an open access article distributed under the terms and conditions of the Creative Commons Attribution license (CC BY).
ORIGINAL RESEARCH
PD1 expression in immune cells within the tumor microenvironment of patients with non-small cell and small cell lung cancer
The Laboratory of Molecular Therapy of Cancer, Cancer Research Institute, Tomsk National Research Medical Center, Russian Academy of Sciences, Tomsk, Russia
Correspondence should be addressed: Evgenia S. Grigoryeva
Evgenia S. Grigoryeva
pereulok Kooperativnyj, 5, Tomsk, Russia; moc.liamg@se.aveyrogirg
Funding: the study was supported by the Russian Science Foundation (grant № 20-75-10033-П).
Author contribution: Kalinchuk AYu — literature search, obtaining and statistically processing the results, writing the article; Tsarenkova EA — obtaining and analyzing data; Loos DM — obtaining and analyzing data; Mokh AA — patients’ curation; Rodionov EO — patients’ curation; Miller SV — data collection; ES Grigoryeva — editing the article; Tashireva LA — study planning and supervision, analysis, and interpretation of results, writing the article.
Compliance with ethical standards: The study was approved by the Ethics Committee of the Tomsk National Research Medical Center Oncology Research Institute (protocol № 7, 25 August 2020; protocol № 18, 25 August 2023), conducted in accordance with federal laws of the Russian Federation and the 1964 Helsinki Declaration with all subsequent additions and amendments regulating scientific research on biomaterial obtained from humans. All participants signed informed voluntary consent to participate in the study.
The immune microenvironment within tumors actively participates in the processes of cancer development and progression. This role is underscored by the rapid expansion of therapeutic approaches that aim to modulate the immune microenvironment, including those for lung cancer. A series of fundamental studies in model systems have revealed pathogenetic mechanisms of progression and microenvironment factors associated with resistance to immunotherapy in lung cancer [1]. For example, a high percentage of PD-1-expressing cells among CD8+ lymphocytes have been correlated with a limited response to PD-1 blockade during polyclonal T-cell stimulation [2]. During the study of the relationship between immunological parameters and the clinical course of the disease in patients, patterns were identified that indicate the significance of the immune microenvironment [3]. Thus, the classification of the tumor immune microenvironment (TIMT) based on PD-1/PD-L1 and CD8+ tumor-infiltrating lymphocytes (TIL) effectively stratifies lung adenocarcinoma patients into groups with varying survival rates. Notably, the group with low CD8+ lymphocyte counts and high PD-1/PD-L1 expression demonstrates the poorest outcomes [4]. The microenvironment demonstrates equally importance in studies examining its association with responses to immunotherapy. For instance, the IKCscore (ImmuneKeratin-Immune Checkpoint score), which is derived from the expression ratios of various genes encoding immune markers and cytokeratins, is a promising indicator for predicting the efficacy of immunotherapy and immunotherapy-based combination therapies in non-small cell lung cancer (NSCLC). Furthermore, the infiltration levels of CD8+ lymphocytes, resting memory CD4+ T cells, and resting dendritic cells are significantly higher in the high IKCscore group than in the low IKCscore group [5]. Expression of programmed death ligand 1 (PD-L1) on tumor cells and tumor mutational burden (TMB) have been identified as predictors of response to immunotherapy. However, the actual clinical scenario shows that these biomarkers alone are insufficient for effectively selecting patients for such type of therapy. Interestingly, despite extensive research into the characteristics of the tumor microenvironment in lung cancer, certain aspects relevant to immunotherapy remain unexplored. One class of immunotherapeutic agents includes antiPD1 antibodies, which target the receptor rather than the ligand. Nonetheless, the expression of PD1 within the lung cancer microenvironment is poorly understood. Given the wide availability of therapeutic options that incorporate immune checkpoint inhibitors, understanding the specificities of the tumor microenvironment becomes crucial. This could enhance the identification of associations with the efficacy of immunotherapy. The aim of this study was to evaluate PD-1 expression in tumor-infiltrating immune cells and its association with clinical outcomes in patients with lung cancer.
METHODS
Patients
The study involved 20 patients (3 women and 17 men) with a morphologically verified diagnosis of lung cancer. Among them, 12 patients had non-small cell lung cancer and 8 had small cell lung cancer. All patients were at disease stage III–IV (10 patients were stage III, 10 — stage IV), with a mean age of 56 ± 6.9 years. Exclusion criteria were stage I–II lung cancer, concomitant autoimmune and inflammatory diseases. The patients completed the full course of treatment in accordance with clinical guidelines recommendations (combination therapy, including first-line and subsequent-line chemotherapy according to the following regimens: Carboplatin/Paclitaxel, Pemetrexed/ Carboplatin, Carboplatin/Etoposide, monochemotherapy with taxanes, Denosumab, chemoimmunotherapy; if indicated, patients underwent external beam radiation therapy). The median follow-up period was 12 months.
Tumor microenvironment immunophenotyping
Tumor tissue samples were collected from all patients prior to any treatment, fixed in formalin, and embedded in paraffin using standard techniques. Subsequently, multicolor staining of the lung cancer tissue was carried out employing TSA (tyramide signal amplification) modification in immunohistochemical analysis. The following primary antibodies were used: human anti-CD8 (Ventana, Switzerland, clone SP57, dilution 1 : 10), anti-PD-1 (ABclonal, China, clone AMC0439, dilution 1:500), anti-CD20 (Leica Biosystems, Germany, clone L26, dilution 1 : 600), anti-CD163 (Diagnostic Biosystems, USA, clone 10D6, dilution 1 : 150) and anti-FoxP3 monoclonal antibody (Invitrogen, USA, clone 236A/E7, dilution 1 : 800). The EnVision FLEX/HRP system (Dako, Denmark) and Opal 7-color Fluorophore Kit (Akoya Biosciences, USA), which includes tyramide conjugated with fluorophores, were utilized for detection. The staining procedure was executed on a BOND RXm automated immunohistostainer (Leica, Germany). The protocol included antigen retrieval using Epitope Retrieval Solution 2 buffer (Leica Biosystems, Germany) at 98.5 °C for 20 minutes, followed by five sequential staining cycles. Each cycle consisted of the following steps: a 10-minute incubation of EnVision FLEX Peroxidase-blocking reagent (Dako, Denmark), a 10-minute incubation with Novocastra Protein Block (Leica Biosystems, Germany), a 30-minute incubation with the primary antibody, a 30-minute incubation with EnVision FLEX/HRP, and a 20-minute incubation with Opal dye. After each cycle, depletion complex of primary and secondary antibodies was performed using Epitope Retrieval Solution 2 buffer. Cell nuclei were counterstained manually using Fluoroshield™ with DAPI (Sigma-Aldrich, USA). Visualization and image acquisition were carried out using the Vectra® 3.0 system (Akoya Biosciences, USA), and quantitative cell analysis was performed using inForm® software (Akoya Biosciences, USA) based on data collected from seven representative tissue regions.
The densities of CD8+ cytotoxic lymphocytes, CD20+ B-lymphocytes, CD163+ tumor-associated macrophages, and FoxP3+ regulatory T lymphocytes were quantified in tumor tissues obtained from patients with lung cancer (fig. 1). The results were represented as the percentage of each cell type relative to the total cell count in the tumor stroma. Statistical analyses of the results were performed using Prism 10 software (GraphPad, USA). The Friedman test was used to detect differences in the number of immune cells within the tumor microenvironment, while the nonparametric Mann-Whitney test was employed for comparing independent groups. Both univariate and multivariate Cox regression analyses were conducted to determine the associations between key clinical and pathological parameters, as well as tumor microenvironment parameters, with progression-free survival. All criteria were two-sided, and differences were deemed significant at p < 0.05.
RESULTS
In the tumor microenvironment of NSCLC, the median proportion of PD-1-positive cells was 1.04 (0.17–1.70)%, in small cell lung cancer (SCLC) — 0.27 (0.03–2.93)%. The frequency of PD-1-positive CD8+ cytotoxic lymphocytes was 83.3% (10/12) in patients with NSCLC and 37.5% (3/8) in patients with SCLC. While the frequency of PD-1-positive CD20+ B-lymphocytes were 8.3% (1/12) and 12.5% (1/8), PD-1positive FoxP3+ T-regulatory lymphocytes were 25% (3/12) and 12.5% (1/8), and PD-1-positive CD163+ macrophages were 25% (3/12) and 20% (2/8), respectively. We studied the proportion of PD-1-positive CD8+ cytotoxic lymphocytes, CD20+ B-lymphocytes, FoxP3+ T-regulatory lymphocytes and CD163+ macrophages, as well as the proportion of other PD-1positive cells (without immunophenotype determination) in the tumor microenvironment of patients with non-small-cell and small-cell lung cancer (fig. 2).
When assessing the cell fraction among all cells in the immune infiltrate of NSCLC, PD-1-positive CD8+ cytotoxic lymphocytes and other PD-1-positive immune cells were predominant among lymphocytes, accounting for 0.24% (range: 0.08–0.46%) and 0.39% (range: 0.00–1.05%), respectively. No significant differences in the quantity of PD-1-expressing immune cells were observed in the SCLC microenvironment. The proportions of PD-1-expressing cells among all studied immunophenotypes were also determined for both NSCLC and SCLC (tab. 1).
When comparing the proportions of PD-1-expressing cells between patients with NSCLC and SCLC, no significant differences were observed (fig. 3).
The analysis of immune microenvironment parameters in conjunction with clinical and pathological factors in lung cancer patients revealed no significant correlations between the studied cell populations and patient age, smoking status, or the presence of distant metastases (stage M1). However, a statistically significant association was observed between disease stage and the proportion of CD8+ lymphocytes in patients with SCLC. Specifically, patients with stage III–IV disease demonstrated a significantly lower proportion of CD8+ lymphocytes in the tumor microenvironment compared to those with stage II (3.49% [range: 3.07–1.19%] vs. 4.82% [range: 3.76–9.88%], p = 0.0286). Furthermore, the relationship between tumor microenvironment characteristics and longterm treatment outcomes was evaluated using Cox regression analysis (tab. 2).
An increased proportion of CD20+ lymphocytes in the tumor microenvironment has been identified as a significant independent factor associated with poor progression-free survival in lung cancer patients (HR = 0.17 [0.02–0.65], p = 0.0454), compared to factors such as stage, histological type, smoking status, presence of regional lymph node metastases, and distant metastases (stage M1). Furthermore, an analysis of the prognostic value of PD-1-expressing immune cell populations in relation to clinicopathological parameters revealed that independent predictors of shorter progression-free survival in lung cancer include the presence of distant metastases (stage M1), a high proportion of PD-1-expressing CD163+ macrophages, and a low proportion of PD-1-expressing FoxP3+ lymphocytes (tab. 3).
DISCUSSION
The clinical significance of PD-1 expression, particularly in the context of immunotherapy, remains poorly understood. Limited and controversial data on the prognostic value of determining PD-1 expression are available in the literature. The study by Dan A. et al. demonstrated that patients with early-stage nonsmall cell lung cancer (NSCLC) who exhibited PD-1 expression of ≥10% on tumor-infiltrating lymphocytes experienced unfavorable 10-year overall survival rates. [6]. Conversely, the study by Sun C. et al. revealed that positive PD-1 expression, coupled with high lymphocyte counts (CD3, CD4, CD8, and FOXP3), was associated with significantly improved survival rates compared to negative PD-1 expression in conjunction with lower lymphocyte counts. [7]. In our study, for the first time, we assessed PD-1 expression while considering the immunophenotype of cells. We focused on the four most relevant populations for which there is evidence suggesting an association with a response to immunotherapy, since the patients included in our study are potential candidates for antiPD-1 antibody therapy in future treatment lines.
When discussing the prognostic significance of PD-1 expression in the context of immunotherapy, it is important to mention the findings of the study by Mazzaschi G. et al., which demonstrated that, a low incidence of PD-1 expression among CD8+ lymphocytes was a distinguishing feature of patients treated with nivolumab, and this was also associated with clinical benefits and extended PFS (HR = 4,51; 95% CI, 1,45–13,94) [8]. In our study, PD-1 expression was found predominantly on CD8+ lymphocytes, although the proportion of PD-1+ cells in the CD8+ lymphocyte population was about 5% and was not associated with PFS. It is known that effector functions are gradually lost during the progressive depletion of T cells; in a recent study, an inverse correlation between T cell function and the level of PD-1 expression was noted [9]. Thus, PD-1 expression on cytotoxic lymphocytes indicates a state of depletion. It is important to note that our study reveals that PD-1-expressing immune cells are extremely sparse within both the non-small-cell and small-cell lung cancer microenvironments. This indicates that the majority of immune cells in the lung cancer environment are predominantly in an active functional state. Our findings suggest that the cells targeted by therapeutic anti-PD-1 antibodies are few within the lung cancer microenvironment, and mainly consist of PD-1expressing cytotoxic lymphocytes. Blocking the checkpoint in these few cells could potentially restore their depleted function. At the same time, the low number of these cells may explain the limited therapeutic effects observed with the use of antiPD-1 antibodies.
Currently, we are unable to evaluate the correlation between specific characteristics of the tumor microenvironment's cellular composition and treatment outcomes in these patients, as their therapy is still ongoing. Nevertheless, this study enabled us to assess the relationship between various tumor microenvironment parameters and PFS. Our results underscore the crucial importance of immune cell composition within the tumor microenvironment in determining PFS in lung cancer patients. Notably, an increased presence of CD20+ lymphocytes has been identified as a significant independent predictor of poorer PFS, exhibiting a hazard ratio (HR) of 0.17 (95% CI: 0.02-0.65, p = 0.0454). This association appears more substantial than conventional prognostic factors, including tumor stage, histological subtype, smoking status, and the presence of regional or distant metastases (stage M1). The results suggest that B-cell infiltration may play a previously underestimated role in lung cancer progression, possibly due to its implications in immune suppression or modulation within the TME. Further studies are required to explore the functional impact of CD20+ lymphocytes in lung cancer, aiming to elucidate their role in tumor biology and resistance to treatment effectively.
Moreover, our analysis concerning PD-1-expressing immune cell populations has unveiled new insights about the links between immune checkpoint expression and clinical outcomes. The presence of distant metastases (stage M1), a high proportion of PD-1-positive CD163+ macrophages, and a low proportion of PD-1-positive FoxP3+ lymphocytes have been identified as predictors of shorter PFS. These observations underscore the complexity of immune regulation within the TME, where a delicate balance between immunosuppressive and immunostimulatory cell populations critically influences disease progression. The association of PD-1+CD163+ macrophages with poor outcomes has predominantly been observed in animal models [10]. Conversely, the anti-tumor effect observed in PD-1+FoxP3+ lymphocytes suggest a potential regulatory function, although Tregs are generally associated with poor outcomes in the existing literature [11].
These findings carry substantial implications for the development of predictive biomarkers and the formulation of immunotherapeutic strategies in lung cancer. The identification of PD-1-expressing immune subsets as prognostic markers underlines the necessity for an enhanced understanding of the TME. Targeting these populations, either through direct modulation or in combination with existing immune checkpoint inhibitors, could pave the way for improved therapeutic outcomes. Nevertheless, the functional heterogeneity within these cell populations calls for more detailed mechanistic studies to clarify their roles in tumor immunity and identify optimal therapeutic targets.
CONCLUSION
Our study provides compelling evidence that the immune landscape of the TME, particularly the composition of PD1-expressing immune cells, is significantly associated with lung cancer outcomes. These findings highlight the critical importance of the immune contexture in determining cancer prognosis and informing therapeutic strategies. Future research should concentrate on validating these findings in larger, independent cohorts and further exploring their predictive potential concerning immunotherapy in lung cancer patients.