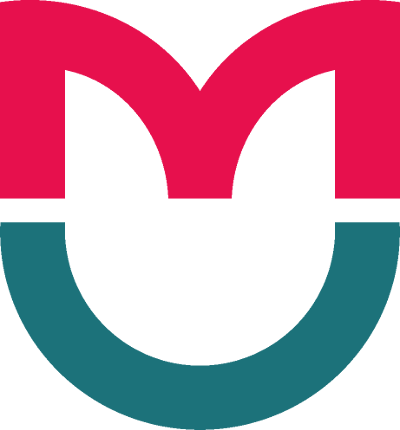
This article is an open access article distributed under the terms and conditions of the Creative Commons Attribution license (CC BY).
METHOD
Comparative analysis of the human sperm cell organelle biochemical markers by confocal Raman spectroscopy
1 Professor Zdanovsky Clinic LLC, Moscow, Russia
2 Institute of Microelectronics Technology and High Purity Materials of the Russian Academy of Sciences, Chernogolovka, Russia
3 Russian University of Medicine, Moscow, Russia
Correspondence should be addressed: Ruslan V. Nazarenko
moc.liamg@davsurzan
Author contribution: Nazarenko RV — data acquisition and analysis, manuscript writing; Irzhak AV — data acquisition and analysis; Gvasalia BR, Pushkar DYu — manuscript editing.
Compliance with ethical standards: the study was approved by the Ethics Committee of the Kulakov National Medical Research Center for Obstetrics, Gynecology and Perinatology (protocol No. 10 dated 28 October 2021).
The last decades were marked by significant advances in various fields related to science-intensive technologies. Reproductive medicine was no exception. Every year more and more methods to assess biomaterials emerge that attract the scientists’ attention.
One new method is Raman spectroscopy (or inelastic scattering spectroscopy), the principles of which were reported more than 80 years ago. Despite long history, only improvement of hardware and software made it possible to use the method to explore the detailed molecular structure (“fingerprint”) of a biological sample.
There is a steadily growing interest of researchers in male infertility in general and identification of the sperm nuclear DNA damage in particular. It is well known that sperm can have good morphology and motility according to assessment criteria of the World Health Organization, but the embryo formed after fertilization of the oocyte with such sperm is characterized by low quality, low implantation rate and often stops developing [1–2]. Some DNA breakages in the sperm can be restored by the oocyte, but when the critical mass of damage is hit, the oocyte repair system is not enough. In this regard, there is growing interest in investigation of the sperm nuclear DNA and assessment of its functional state. Since the routine methods to assess ejaculate make it impossible to obtain such information using light microscopy, supplementary sperm assessment methods have recently became common. This is particularly true for sperm DNA fragmentation assessment methods [3–7]. These include staining with aniline blue, chromomycin A3, chromatin dispersion test (Comet), TUNEL, SCSA, etc. Unfortunately, all these methods are destructive and, therefore, provide very little practically useful information for using in assisted reproductive technologies. There is a demand for the method meeting the following criteria: allowing one to assess a single sperm without damaging it, allowing one to assess the sperm nuclear DNA integrity and its functional state, i.e. biochemical and metabolic profile, and to use this sperm for further fertilization by ICSI (Intra Cytoplasmic Sperm Injection). Considering the above requirements, it seems promising to study the confocal Raman spectroscopy capabilities in terms of obtaining more detailed information about the sperm structure and functional state.
Recently there have been more and more reports focused on using confocal Raman spectroscopy to assess biological tissues, living cells, subcellular organelles, and intracellular metabolic processes. However, there are not so many studies focused on assessing the sperm spectral characteristics. In 1980, Raman spectroscopy was applied to living cells, which involved the use of salmon sperm [8]. When comparing the Raman spectra acquired for sperm with normal and abnormal morphology [9], the researchers have drawn a conclusion that sperm morphology is not always correlated to proper packaging of its nuclear DNA. When assessing spectra acquired from subcellular organelles and the effects of UV radiation on those [10], spectra of the sperm acrosome, nucleus, and midpiece were reported. Other authors resumed working on the use of Raman spectroscopy for imaging of the sperm nuclear DNA damage [11]. They used UV radiation exposure to disrupt the DNA structure and reported typical band changes in the 1095/1050 cm–1 region. Oxidative DNA damage in the Fenton reaction with hydrogen peroxide was also reported [12]. The spectra acquired from spermatozoa damaged in this way fully matched the earlier reported ones [11]. We assumed the association between sperm morphology, its nuclear DNA integrity, and the Raman spectra acquired for the nuclear region [13]. Later these results were confirmed by other authors using the chromomycin A3, aniline blue, and acridine orange dyes for comprehensive assessment of the sperm nucleoprotein complex [14].
In the era of the omics (metabolomics, proteomics, etc.) revolution, Raman spectroscopy represents a powerful tool for investigation of biochemical processes in the cell. However, it is impossible to use the method and interpret the results without engaging experts, i.e. physicists, chemists, mathematicians, since the knowledge and skills essential for working with this method are far beyond the limits of biological or medical education.
The study aimed to acquire information about spectral characteristics of the human sperm cellular organelles, as well as to estimate the damaging effect of x-ray radiation on the human sperm nuclear nucleoprotein complex based on changes in Raman spectra.
METHODS
Healthy donors gave sperm samples through masturbation. The sexual abstinence period was 2–7 days.
Inclusion criteria for sperm donors: age 18–35 years; no hemotransmissive infection; no sexually transmitted infection; no severe chronic somatic disorder; no mental disorder; normal male karyotype 46 XY.
Exclusion criteria for sperm donors: fever at the moment of giving ejaculate; taking antibiotics, glucocorticoids, antidepressants at the moment of giving ejaculate; lack of the required (2–7 days) sexual abstinence period.
In each case routine semen analysis by light microscopy was performed. The results were interpreted in accordance with the WHO Guidelines 2010; the results corresponded to normozoospermia.
Sample preparation for confocal Raman spectroscopy
Ejaculate was centrifuged using the double density gradient technique (FertiPro, Breenem; Belgium) for 20 min at 415 g. After removing supernatant, precipitate was resuspended in phosphate buffered saline (PBS; Sigma–Aldrich, USA) at 37 °C and centrifuged again for 10 min at 415 g. Then 10 µL of the sperm suspension were applied to the aluminum substrate, the preparation was fixed using 70% ethanol and air dried.
Confocal Raman spectroscopy
The Senterra confocal Raman microscope (Bruker, Germany) was used for assessment. The instrument consists of two main blocks: a confocal binocular microscope with the image digitalization option and a spectrometer. A fully confocal system can adjust to three different excitation wavelengths ensuring the maximum possible spatial resolution. Since Senterra is based on the optical microscope, all the tools needed for visual characterization of the sample are available. Spectral analysis of various sperm compartments (nucleus, acrosome, midpiece) was performed at the laser excitation wavelength of 532 nm and power of 10 mW within the range of 280–1730 cm–1 with the 3–5 см–1 resolution. The signal accumulation time for the point was 3x 20 s. Safety of the cell exposure to laser radiation and the lack of photo-induced damage with the specified power parameters have been confirmed in the literature [15].
Spectral pre-processing
Each spectrum was analyzed within the range of 680–1700 cm–1 after baseline correction through polynomial baseline fitting and smoothing by the 21 point moving average method. Furthermore, standard normal variate (SNV) normalization was applied to all spectra.
The pre-processed spectra were used to make a data matrix X (176 × 510), where each row contained the Raman spectra intensities within the range of 680–1700 cm–1 with an increment of 2 cm–1 and the number of rows corresponded to the number of spectra.
In the second phase we assessed the sequelae of the x-ray radiation damaging effects on the sperm. For that the sperm suspension was divided into three aliquots. The first aliquot represented a control sample; the second and the third aliquots were exposed to the focused x-ray beam for 5 and 10 min, respectively. The results obtained were compared with each other.
RESULTS
A total of 19 sperm samples collected from 19 healthy donors aged 18–35 years were tested. A total of 176 spectra were acquired; the spectra inherent to sperm with normal morphology were identified.
Figure fig. 1А presents a morphologically normal sperm. Dots mark the areas, for which spectra has been acquired. Label 1 corresponds to the sperm nucleus, label 2 corresponds to the acrosome, label 3 corresponds to the midpiece. Figure fig. 1B presents a comparative chart of the spectral characteristics obtained for each area.
The Raman spectra acquired for nuclei of the intact spermatozoa and spermatozoa exposed to x-ray radiation for 5 and 10 min are provided in fig. 2.
Despite different intensity of the spectra acquired, we noted no differences in the major Raman bands inherent to cells with DNA damage under 5 and 10 min exposure to x-ray radiation compared to intact samples.
DISCUSSION
The causes of abnormal sperm development or further damage are extremely diverse, and manifestations of such abnormalities are multivalent.
Based on the literature data we matched the bands we had acquired by Raman spectroscopy to the corresponding groups of atoms (see ).
When assessing the sperm spectra (fig. 1B), the following characteristic bands can be distinguished.
Inherent to nuclear DNA (1092 cm–1 and 780 cm–1). The 1092 cm–1 band is B-DNA and Z-DNA marker. It is normally characterized by a gently sloping or horizontal arm in the 1063 cm–1 region. This feature is inherent to the nucleus with intact DNA. The band in the 780 cm–1 region corresponds to vibrations of thymine and cytosine, as well as to the DNA core. Some authors believe that this band is a marker of protamine DNA packaging, which has been confirmed by the later research [9, 16]. A triple band in the 1420 cm–1, 1445 cm–1 and 1486 cm–1 regions is characterized by specific ladder-like shape with the uppermost part in the 1486 cm–1 region. The band typical for phenylalanine amino acid (1002 cm–1) is permanent, it is found in almost all graphs with different intensity.
Inherent to the acrosome. When assessing acrosomal spectral characteristics, it can be noted that there is an overall intensity reduction in almost all bands. Changes in the shape of the triple band 1420 cm–1, 1445 cm–1, and 1486 см–1 with predominance of the middle part in the 1445 cm–1 region are typical, which corresponds to methylene deformation of endogenous lipids.
Inherent to the sperm midpiece (neck). Spectral characteristics of the midpiece are distinguished by the high intensity band in the 748 cm–1 region being a marker of the presence of mitochondria [4]. Intensity also grows of the 1576 cm–1 band, which, together with nucleic acids, corresponds to large amount of ATP [10]. Extinction of the 1092 cm–1 band and its transfer to the 1127 cm–1 region attract attention. This can be due to the presence of mitochondrial DNA, but we have found no confirmation in the literature.
The lack of significant spectral changes under exposure to x-ray radiation can be explained by high enough stability of the intact nucleoprotein complex of the mature sperm, in contrast to the actively dividing sperm precursor cells.
CONCLUSIONS
The pilot study conducted has made it possible to show the capabilities of Raman spectroscopy in terms of assessing the sperm biochemical processes and ultrastructure. We have created our own library of the spectra acquired for normal spermatozoa and the model with the sperm artificially damaged with the x-ray beam. The study involved fixed sperm. However, it is theoretically possible to select the intact live sperm for fertilization by ICSI using confocal Raman spectroscopy equipped with the optic (laser) tweezers. Further research in this field is necessary.