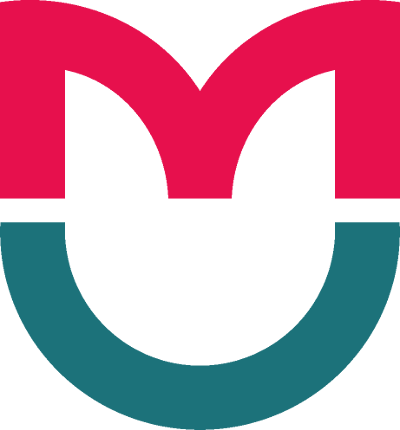
ORIGINAL RESEARCH
Contrast enhanced MRI of tumors using gadopentetic acid linked to cyclodextrin by an ester bond
1 Burnasyan Federal Medical Biophysical Center of Federal Medical Biological Agency, Moscow, Russia
2 N. N. Blokhin Russian Cancer Research Center, Moscow, Russia
3 P. V. Sergeev Molecular Pharmacology and Radiobiology Department, Biomedical Faculty,
Pirogov Russian National Research Medical University, Moscow, Russia
4 Department of Petroleum Chemistry and Organic Catalysis, Faculty of Chemistry,
Lomonosov Moscow State University, Moscow, Russia
Correspondence should be addressed: Nikolay Shimanovsky
ul. Ostrovityanova, d. 1, Moscow, Russia, 117997; ur.umsr@namihs
Funding: this work was supported by Government Contract No. 11411.1008700.13.081 dated September 13, 2011 as part of the Federal Targeted Program for the Development of Pharmaceutical and Medical Industry of the Russian Federation until 2020.
Gadolinium enhancement is a must in cancer diagnosis assisted by magnetic resonance imaging (MRI). The most common contrast agent is gadopentetic acid (Gd-DTPA, marketed as Magnevist). Gd-DTPA-enhanced MRI is highly informative; however, it must be ordered with caution due to a risk of nephrogenic systemic fibrosis that can be induced by gadolinium release from the chelate in patients with slow Gd-DTPA clearance [1]. Therefore, finding a chelating agent with increased relaxivity (imaging capacity) remains a high priority issue, since it can facilitate switching to lower doses of the contrast agent and thus reduce the risk of nephrogenic systemic fibrosis. Cyclic hydrocarbons, such as α-, β- and γ -cyclodextrins (CDs) [2], may have good prospects here, as they are already enjoying a wide application in pharmacy as solubilizers for poorly soluble compounds and are employed in other areas of science and engineering [3].
A CD has an ability to selectively form inclusion complexes with other molecules, ions and even radicals that determines its role in basic and applied research. CDs can form complexes in solutions and in the solid phase; a good example would be CD-based chemical sensors whose mechanism of action has been well studied [4].
Gadolinium-based contrast agents are not manufactured in Russia. This fact seriously limits the scope of pathologies that could be detected on MRI (malignant tumors in the first place) and impedes treatment monitoring.
The potential of cyclodextrins in the development of gadolinium-containing contrast agents makes them an attractive research object. Cyclodextrins have good prospects due to the availability of hydroxyl groups on the molecule surface and the ability to form inclusion compounds. Numerous attempts to create gadolinium-modified cyclodextrins demonstrated that gadolinium relaxivity in such complexes significantly increases [5, 6]. However, their imaging capacity in vivo has not been studied yet.
The basic requirements for a CD-based complex compound are as follows: small molecule size (<5.0 nm); nontoxicity (the molecule must remain nontoxic and stable when exposed to physiological conditions for several hours); the chemical bond between a Gd3+-based complex compound and a CD is expected to break inside the body; a Gd3+ complex must be stable at pH = 6.0–8.0.
The aim of this work was to evaluate relaxivity of Gd-DTPA linked to β-CD by an ester bond and to compare MRI scans enhanced with the experimental compounds and Magnevist.
METHODS
The following reagents were used in the experiment: reagent grade diethylenetriaminepentaacetic acid, melting point above 219 °С; β-cyclodextrin (β-CD, Aldrich, USA), purity >99 %; Gd(NO3)3·6H2O (Aldrich, USA), purity of 99.99 %; reagent grade or chemically pure organic solvents. Synthesis of gadopentetic acid linked to β-CD was performed according to the patent [7]. In total, 5 samples were synthesized. Their properties are described in tab. 1. Magnevist (Bayer, Germany) was used as a comparator. Gadolinium concentration in the experimental formulations (aqueous solutions with pH 7.2 ± 0.2) was 0.3 М.
Gd content in the samples was measured on the X-ray fluorescence analyzer Х-Art М (Comita, St. Petersburg). The weighted amount of the substance was dissolved in distilled water to a concentration of 30 mg/ml. 1 ml of the obtained solution was placed into a special cuvette. Then, 1 ml of distilled water was added to the solution, 1 ml of the obtained sample was placed in a cuvette for X-ray fluorescence analysis that was performed subsequently. 1 ml volumes were selected to ensure complete wetting of cuvette surface and measurement uniformity. Quantitative assessment of the ligand (chelator) and CD-based complex content in the synthesized samples was performed using mass spectrometry. ESI-MS spectra were registered with the Agilent LS/MS 1100 SL ion trap mass spectrometer (Agilent Technologies, USA) using electrospray ionization (ESI) in the positive/negative ion mode. The results are presented in tab. 1.
Т1 relaxometry was performed on the ClinScan MRI scanner by Bruker BioSpin, Germany (field strength of 7 Tesla, proton resonant frequency of 300 MHz). Tubes filled with various concentrations of the experimental compounds were placed in water for fine tuning of the magnetic field. Then, data acquisition was performed. Magnevist aqueous solution was used as a control (Magnevist concentration was identical to that of the studied compounds). Relaxation time (Т1) was calculated using Turbo Inversion Recovery sequence [8]. Signal intensity from each sample was measured using ImageJ software (National Institutes of Health, USA). Т1 values of the studied compounds were computed using the following formula, where SI is signal intensity; Т1 is spin-lattice relaxation time; TI is inversion time; TR is repetition time; TE is echo time; α is an impulse deflection angle.
Computation was done using Mathcad software (PTC, USA). Imaging capacity of the studied samples was evaluated based on Т1 values using the ClinScan MR scanner by Bruker BioSpin, Germany (field strength of 7 Tesla, proton resonant frequency of 300 MHz) and a two-segment surface receiver coil. First, fast orthogonal Т1-weighted images (WIs) were obtained. Then, coronal fat-suppressed Т2-weighted images were obtained using Turbo Spin Echo impulse sequence (TR = 2220 ms, TE = 49 ms, section thickness of 1 mm, 16 sections, matrix of 320 × 320, FOV read = 50 mm, BLADE K-space trajectory with 100 % coverage). Axial fat-suppressed Т2-WIs were obtained using Turbo Spin Echo sequence (TR = 3310 ms, TE = 51 ms, section thickness of 1 mm, 26 sections, matrix 256 × 256, FOV read = 40 mm). Prior to MR scanning, each animal was anesthetized with 6 mg chloral hydrate diluted in normal saline. The animals fell asleep 4 to 10 minutes after the injection. Unenhanced control scans were performed in multiple positions for 20 minutes. Then, the animals received retro-orbital and intraperitoneal injections of Magnevist (the comparator) or the experimental compound in the amount of 1 mg of Gd per mouse (2 × 10−3 mmol of Magnevist, 1.5 × 10–3 mmol or К14-1). Retro-orbital route of administration was used instead of tail vein injections. Then, dynamic MRI was performed (fig. 1, fig. 2, fig. 3, fig. 4). After the experiment, the animals were sacrificed.
To assess how the experimental compound was accumulated in tumor tissues, we analyzed signal intensity in the tumor before and after 2 × 10–3 mmol of К14-1 or Magnevist had been administered. We selected a tumor region on the MR image and a similar region on the contralateral side and calculated average signal intensities (SIс and SIn, respectively). Average noise levels were measured in the areas outside the mouse body. For each compound, contrast-to-noise ratio (CNR) at a specific time point was calculated according to the following formula.
We also compared time-dependent accumulation of gadolinium in pathological and normal tissues by computing signal-to-noise ratio (SNR) in tumor and healthy tissues at each time point (formula).
fig. 5 shows dependency between SNR values and time relapsed after i. v. injection of K14-1 and Magnevist in mice with transplanted melanoma.
Bio-experiments
The experiments were carried out on С57BL/6 male and female mice (weight of 18–20 g) and Wistar male and female rats (weight of 150–200 g) provided by Stolbovaya breeding nursery. All animals had a veterinary certificate (Form 1).
The experimental model complied with the guidelines for preclinical laboratory studies of novel formulations [9, 10]. For MRI-based assessment of imaging capacity of synthesized compounds, three mouse cancer cell lines from different tissue types were ordered from the Cell Bank of Blokhin Russian Cancer Research Center, namely, Lewis lung carcinoma LLC1, breast adenocarcinoma Ca755 and melanoma В16-F10.
Cancer cell transplantation was performed in compliance with standard regulations [9, 10, 11]. A suspension of cancer cells (4 × 106 cells per mouse) was subcutaneously injected in the mouse thigh. The experiment went on after tumors had reached a 1 cm diameter.
Acute toxicity tests. The animals received single intravenous doses of 3,000, 6,000, 9,000, 12,000 and 15,000 mg/kg of the studied compounds. Observation lasted for 30 days. Acute toxicity assessment was based on the number of animals found dead during the test and the day of their death [9].
Cytotoxicity of the studied compounds was assessed according to the guidelines [12] using the MTT-assay [12]. Color development was measured by detecting optical density of the sample at 530 nm wavelength using a plate photometer (AIFR-01 UNIPLAN Immunoassay Analyzer by PIKON, Russia). Percentage viability was defined as the ratio of the average optical density of the studied compound at a given concentration to the average optical density of the control sample. Data were processed using the Mann-Whitney nonparametric test. Difference was considered statistically significant with p <0.05.
Fibroblast preparation. A parietal bone consists of actively proliferating cells. The bone of a neonatal rat was isolated aseptically. Briefly, after decapitation and surface sterilization, the skin was removed and the parietal bone was taken out. The parietal bone (1 cm2 in size) was placed into a sample bottle with DMEM culture medium (PanEco, Russia) and cut into 1–2 mm3 fragments. Then, the medium with tissue fragments was placed onto the bottom of 50 ml Costar flat-bottom culture flasks by Corning, USA (bottom square area of 25 cm2), positioned at a 45° angle and incubated for 30 minutes at 37 °С. The fragments adhered to the inclined surface. 30 minutes later, the medium was removed, the flasks were repositioned horizontally and 10 ml of DMEM were added supplemented with 20 % heat-activated fetal bovine serum, 100 µg /ml of L-glutamine and 40 µg /ml of gentamicin sulfate.
Fibroblasts were cultured under sterile conditions. The cells were incubated at 37 °С and 5 % СО2. All procedures were carried out in the LС laminar flow cabinet (Laminar Systems, Russia). A week later, patches of cell monolayers were observed on the flask surface. For better distribution, the cells were treated with trypsin; another week later, a multilayer culture was obtained.
Data were statistically processed using GrapthPad Prism software (GraphPad Software, USA).
RESULTS
Т1-relaxivity values of the synthesized Gd-DTPA-CD compounds and Magnevist are presented in tab. 1. Т1-relaxivity of all synthesized samples is higher, compared to Magnevist, but the highest relaxivity is demonstrated by К14-1, К13-1 and К13-2 samples. The β-CD compound (sample code К14-1) has a number of advantages over the comparator: its Т1-relaxivity is 50 % higher, compared to Magnevist, while gadolinium concentration in it is 40 % lower. It means that malignant tumors can be visualized using lower gadolinium doses while retaining the same quality of MR images and reducing the risk of complications.
Experiments on animals were carried out in compliance with international guidelines [13]. Intravenous injections of 3,000–6,000 mg/kg of the studied samples did not induce toxicity in rats of both genders. Death was observed in male and female rats at 9,000 mg/kg and over. The animals died no earlier than 24 hours after the injection. The animals who received sublethal doses retained normal weight during the observation. Autopsy did not detect any visual changes in the organs. Males were more sensitive to Gd-DTPA-CD than females; 2 out of 6 females and 1 out of 6 males survived after receiving 12,000 and 15,000 mg/kg of the compound. LD50 was 7 ± 1 mmol/kg.
Sample К14-1 did not exhibit significant toxicity toward rat fibroblasts at all studied concentrations (tab. 2).
DISCUSSION
Considering the criteria for imaging capacity required of MR contrasting agents, namely, the maximum content of Gd3+ paramagnetic ions and the maximum relaxivity value (tab. 1), we selected samples К13-1, К13-2, К14-1, К14-2 for in vivo assessment of the diagnostic value of MRI enhanced with these compounds. The best tumor enhancement was observed with К14-1 sample (fig. 1, fig. 2, fig. 3, fig. 4). With Magnevist and β-CD (code K14-1), enhancement quality was practically the same, although a covalently bonded Gd-DTPA — β-CD complex contains 40 % less gadolinium than Magnevist. Animals with transplanted tumors received the same amounts of gadolinium, but the β-CD derivative (code К14-1) substantially increased magnetic resonance signal in well vascularized tumors, such as breast adenocarcinoma Ca755, compared to Magnevist, which is definitely an advantage (fig. 5).
Cytotoxic effects of various concentrations of К14-1 and Magnevist were evaluated based on cell viability in the following cell cultures: Lewis lung carcinoma LLC1, melanoma B-16 and breast adenocarcinoma Ca755. With K14-1, results of all cytotoxicity tests in B16-F10, LLC1, and Ca755 cell lines were similar (cell growth was inhibited): IC50 = (1 ÷ 2) × 10−4 М; IC90 = (0.2 ÷ 0.5) × 10−4 М. IC50 computed for К14-1 fell within the range of standard cytotoxicity values suggested for active compounds (IC50 ≤ 10−4 М), therefore, we conclude that sample К14-1 does not exhibit conspicuous toxicity.
Biological activity of gadolinium compounds was assessed using normal fibroblasts of the rat parietal bone. These cells are highly viable and easy to culture in vitro. Fibroblasts are a common test object in the assessment of toxicity of various compounds [10].
Unlike Magnevist, sample К14-1 did no exhibit significant toxicity toward rat fibroblasts. Magnevist reduced cell viability by 83 and 27 % at 10 and 1 % concentrations, respectively (tab. 2).
CONCLUSIONS
Magnevist, a contrast agent for MRI enhancement, and a modified Gd-DTPA-CD complex (sample К14-1) have relatively equal imaging capacity in mice with transplanted tumors.
Sample К14-1 is original and easy to formulate; it exhibits high relaxivity and allows using 40–50 % less gadolinium for in vivo imaging, compared to Magnevist, thus reducing the risk of adverse effects, such as nephrogenic systemic fibrosis. Gd-DTPA-CD provides better enhancement in well vascularized tumors, compared to Magnevist.