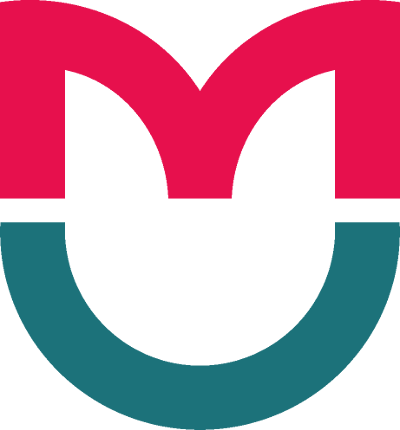
This article is an open access article distributed under the terms and conditions of the Creative Commons Attribution license (CC BY).
ORIGINAL RESEARCH
Analysis of mildronate effect on the catalytic activity of cytochrome Р450 3А4
1 Laboratory of Bioelectrochemistry, Department of Personalized Medicine,
Institute of Biomedical Chemistry, Moscow, Russia
2 Department of Biochemistry, Biomedical Faculty,
Pirogov Russian National Research Medical University, Moscow, Russia
3 Department of Clinical Pharmacology and Propaedeutics of Internal Diseases, Faculty of General Medicine,
I. M. Sechenov First Moscow State Medical University, Moscow, Russia
4 Institute of Bioorganic Chemistry of the National Academy of Sciences, Minsk, Republic of Belarus
Correspondence should be addressed: Victoria Shumyantseva
ul. Pogodinskaya, d. 10, Moscow, Russia, 119121; ur.liam@avestnaymuhs_v
Funding: this work was conducted under the Federal Fundamental Scientific Research Program for 2013–2020.
Translational medicine is a developing branch of molecular medicine aimed to translate basic research into clinical practice. Isozymes of cytochrome Р450 (CYP) are a superfamily of haem-containing monooxygenases responsible for phase I reactions of biotransformation of xenobiotics, including 75 % of drugs, and for the metabolism of endogenous physiologically active compounds [1, 2].
Due to cytochrome Р450-dependent metabolism of drugs, drug pharmacokinetics and response to drug therapy vary in individual patients. Among 57 isozymes of human cytochrome Р450, 5 basic forms are distinguished (CYP1A2, CYP2C9, CYP2C19, CYP2D6, CYP3A4/5) responsible for about 95 % of biotransformation reactions [3, 4, 5]. Isozymes of cytochrome P450 catalyze a variety of chemical reactions, such as hydroxylation, O-, S-, N-dealkylation, epoxidation, sulfoxidation, deamination, dehalogenation, etc. As cytochromes Р450 have broad substrate specificity, research into drug interactions in cytochrome Р450-based systems is of particular clinical importance [6, 7, 8]. To estimate a risk of drug interference with clinical tests at a preclinical research phase and to predict drug biotransformation rates in in vitro systems, methods of electroanalysis have been developed for clinically significant types of cytochrome Р450 enzymes [9, 10, 11, 12].
Among the participants of a catalytic cycle of cytochromes Р450 are their redox partners, namely, cytochrome Р450 reductase and cytochrome b5, and nicotinamide adenine dinucleotide phosphate (NADPH), which serves as an electron donor. To trigger catalysis, all components of a complex electron transport chain must make their contribution. Electrochemical analysis of cytochrome Р450 catalytic activity does not require the presence of redox partners or NADPH electron donors (fig. 1). Electroanalysis of cytochrome Р450 catalytic activity is a noninvasive tool that can be used to study the mechanism of xenobiotic biotransformation and drug-drug interactions. Due to their high sensitivity, electrochemical methods of analysis may be very efficient in studying enzyme-substrate interactions [13]. During cytochrome Р450-dependent catalysis in the electrochemical system, cathodic (reduction) current is registered. Its increase indicates the additional flow of electrons to the organic substrate (drug). Negative cathodic current (unlike positive anodic current) serves to measure the electrocatalytic activity of the enzyme. Study of cytochrome P450 electroanalytical parameters is a crucial step towards the discovery of new substrates/inhibitors of this hemoprotein; it is also important for predicting drug-drug interactions and drug interference with clinical diagnostic tests [14, 15].
We have developed a new method of electroanalysis that allows using enzyme-electrode systems as noninvasive tools for the assessment of cytochrome P450 catalytic activity in preclinical research aimed to discover new substrates, inhibitors and modulators for this type of enzymes [18]. In spite of significant advances in diagnostic and treatment approaches, cardiovascular diseases (CVD) remain the leading cause of death and morbidity in the prime working age population in developed countries, including Russia. New strategies for CVD treatment are being elaborated. When an association between free fatty acids (FA) and a risk of death from an atherosclerotic cardiovascular pathology was identified, studies were launched to investigate inhibitors of partial β-oxidation of FA (pFOX, or partial fatty acid oxidation inhibitors) [19]. Metabolic drugs that aid oxygen uptake, ensure more efficient metabolic pathways and protect tissues from oxidative stress at reperfusion are also expected to produce an anti-ischemic effect due to the impact they have on the myocardial metabolism [20].
In the mid 1970s, researchers of the Latvian Institute of Organic Synthesis synthesized trimethylhydrazinium propionate (commonly referred to as mildronate, or meldonium) that inhibits FA transport across membranes [21]. It was shown that mildronate reduces the rate of β-oxidation of FA in mitochondria, which is important in cases of excessive FA accumulation [22]. Mildronate triggers ischemic preconditioning by reducing the rate of FA transmembrane transport, inhibiting accumulation of acyl-CoA and acylcarnitine inside the cell, optimizing oxygen consumption, inhibiting β-oxidation of FA and increasing the rate of γ-butyrobetaine synthesis. It also induces NO synthesis in the vascular endothelium reducing peripheral vascular resistance and platelet aggregation; increases elasticity of red blood cell membranes; minimizes metabolic acidosis caused by anaerobic glycolysis with subsequent accumulation of lactic acid. Mildronate is used in combination therapies of CVD; in patients presenting with fatigue or physical stress; in a post-operative period to expedite recovery; in abstinent patients with chronic alcoholism [23, 24].
Though mildronate is widely used in combination therapies as a metabolic antihypoxant, its effect on the catalytic functions of cytochrome Р450 enzymes, i. e. enzymes involved in phase I of biotransformation of xenobiotics, has not been studied. When planning a combination therapy, it is necessary to remember that drug-drug interactions may have both therapeutical and adverse effects on the patient. Therefore, of particular importance is information about substrate properties of pharmaceutical agents used in combination therapies or their capacity to inhibit or induce cytochrome Р450 isozymes.
Previously, we used electrochemical methods to study antioxidant vitamins (vitamins С, А and Е) and vitamin-like compounds (taurine and coenzyme Q) that were shown to have a positive effect on the electrocatalytical activity of cytochrome Р450 3А4 [18, 25]. Therefore, the aim of this work was to investigate the effect of mildronate on catalytic functions of cytochrome Р450 3А4, an isozyme that participates in the biotransformation of more than 50 % of existing drugs.
METHODS
Electrochemical experiments were carried out using Autolab PGSTAT 12 potentiostat/galvanostat (Metrohm Autolab, Netherlands) with GPES software (version 4.9.7). All measurements were performed at room temperature. Electrochemical analysis of cytochrome Р450 3A4 was carried out in 0.1 М potassium phosphate buffer (рН 7.4) containing 0.05 М NaCl. We used three-pronged screen-printed electrodes (LLC ColorElectronics, Russia) — graphite working and auxiliary electrodes and a silver chloride reference electrode. The working electrode was 2 mm in diameter. All potentials are referenced to the Ag/AgCl reference electrode. Spectral measurements were done using Cary 100 UV-Vis spectrophotometer (Agilent Technologies, USA) and Cary WinUV software supplied by the vendor.
Cyclic voltammograms were recorded at a scan rate of 10–100 mV/s. Parameters for cathodic square-wave voltammetry were as follows: initial potential of +100 mV, final potential of –600 mV, step potential of 5 mV, amplitude of 20 mV, frequency of 10–100 Hz. The following reagents were used: didodecyldimethylammonium bromide (DDAB) and erythromycin by Sigma-Aldrich (USA); mildronate by Grindeks (Latvia); acetic acid, ammonium acetate and acetylacetone by LLC Spektr-Chim. Recombinant human cytochrome Р450 3А4 (182 µM in 550 mM potassium phosphate buffer with рН of 7.2 containing 0.2 % CHAPS, 1 mM dithiothreitol and 20 % glycerol) was engineered, isolated and described at the Institute of Bioorganic Chemistry (Minsk, Belarus). Enzyme concentration was measured spectrophotometrically based on the formation of a reduced enzyme-CO complex using the extinction coefficient ε450–490 = 91 mM–1cm–1 [26].
The surface of the working graphite electrode was coated with 1 µl of 0.1 М chloroform solution of DDAB. After evaporation of chloroform (10 min) 1 µl of 18.2 µM cytochrome Р450 3А4 was applied on the surface of the working electrode. The electrodes were allowed to stay for 12 h at +4 °С in a humid chamber to prevent from total drying. The N-demethylase activity of cytochrome Р450 3А4 towards erythromycin was estimated by the accumulation of formaldehyde, that forms a colored compound with Nash reagent (4 М ammonium acetate, 0.1 М glacial acetic acid, 0.04 М acetylacetone); the extinction coefficient ε412 was 4 mM–1cm–1 [27, 28]. The enzyme-coated electrode was then immersed in 1 ml of electrolytic buffer containing 100 µM erythromycin. Electrolysis was performed at the controlled potential of –0.5 V for 20 min. After the electrolysis Nash reagent was added to the incubation mixture at 1:1 ratio, and the mixture was incubated at +37 °С for 30 min to develop the color. The concentration of formaldehyde that had been produced during electrocatalysis was measured spectrophotometrically. Figures 3, 4 and the table below show mean values and standard deviation obtained in 3–5 individual experiments.
RESULTS
Mildronate belongs to a class of antihypoxant agents and is used in a combination drug treatment of various diseases. Its effect on the catalytic functions of cytochrome Р450 3А4 immobilized on a screen-printed graphite electrode (SPE) modified with didodecyldimethylammonium bromide (SPE/DDAB) was studied by registering peak maximum of cathodic current using cyclic voltammetry. As shown in fig. 2, mildronate does not have any effect on the electrochemical reduction of cytochrome Р450 3А4, does not increase or decrease reduction current, i. e., does not exhibit substrate or inhibitor properties towards the enzyme. Besides, the spectral analysis of cytochrome P450 3A4 binding to mildronate showed that the latter does not induce type I (substrate) or type II (inhibitor) changes of cytochrome Р450 3А4 difference spectrum, which corresponds to the data obtained using the electrochemical system. Analysis of the dependence of the reduction current on mildronate concentrations at a 10–75 µM concentration range also confirmed the absence of mildronate effect on cytochrome Р450 3A4 reduction (fig. 3).
Effect of mildronate on cytochrome Р450 3А4-dependent biotransformation of erythromycin was studied using 50 µM mildronate and 100 µM erythromycin. Erythromycin N-demethylation catalyzed by cytochrome Р450 3А4 was registered by the accumulation of formaldehyde. A product of the Hantzsch reaction is a colored formaldehyde derivative, which was registered in our experiment spectrophotometrically at 412 nm [26]. As shown in the table below, catalytic constants kcat of electrocatalytic cytochrome Р450-dependent reactions have comparable values.
DISCUSSION
Catalysis and drug-drug interactions were estimated based on the electrochemical activity of cytochrome P450 3A4 enzyme immobilized on the electrode surface. In the course of electroanalysis, we registered voltammetric electrode response by cyclic voltammetry and square-wave voltammetry. Substrates of cytochrome Р450 enzymes caused a significant increase in the catalytic current (fig. 4, experiments 2 and 5) while their inhibitors did not alter or reduce the maximum amplitudes of the currents [13]. Binding of itraconazole, a cytochrome Р450 3А4 inhibitor, to the enzyme did not cause increase of the cathodic current, because no additional electrons transfer occurred in the system (fig. 4, experiment 3).
Previously, we studied a stimulating effect of metabolic antioxidants on phase I of the catalytic cycle of cytochrome P450, which is the reduction of haem iron [18]. A study of interactions between diclofenac, a substrate of cytochrome Р450 3А4, and a number of pharmaceutical agents, such as L-carnitine and a vitamin-like antioxidant thioctic (alpha-lipoic) acid showed that these drugs do not affect the catalytic current registered during diclofenac interaction with the enzyme (fig. 4, experiments 6–8). All drugs were studied at concentrations of 10–400 µM. Such range of working concentrations was chosen based on Michaelis constant calculations and blood plasma drug concentrations [29]. Directed regulation of the catalytic cycle of cytochrome Р450 can both reduce drug metabolism rates and induce substrate biotransformation [30, 31, 32].
No increase in the value of the electrochemical constant of P450 3A4-dependent erythromycin N-demethylation was observed in the presence of 50 µM lipoic acid in the electrochemical system. Comparison of kinetic parameters allows us to conclude that the macrolide antibiotic erythromycin and the metabolic antioxidant thioctic acid can be used together in a combination therapy as they do not interact.
Mildronate, as well as L-carnitine and lipoic acid, does not affect the electrocatalytic activity of cytochrome Р450 3А4.
CONCLUSIONS
A study of drug-drug interactions between the typical cytochrome Р450 3А4 substrates (diclofenac and erythromycin) and metabolic antioxidants and also the antihypoxant drug mildronate was carried out using electroanalytical methods. Mildronate, as well as L-carnitine and lipoic acid, does not affect electrocatalytic activity of cytochrome Р450 3А4, which indicates a lower probability of drug-drug interactions with regard to their metabolism in a combination drug therapy. This fact must be considered by physicians when deciding on the optimal antihypoxant and antioxidant in a combination therapy of comorbid patients.