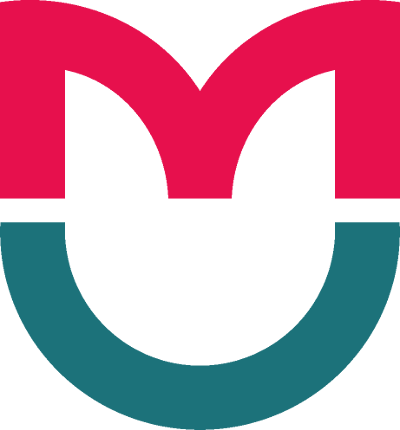
ORIGINAL RESEARCH
Observing the dynamics of the NAD+ to NADH ratio in Danio rerio embryo tissues using a genetically encoded biosensor
1 Laboratory for Molecular Technologies,Shemyakin and Ovchinnikov Institute of Bioorganic Chemistry of the Russian Academy of Sciences, Moscow, Russia
2 Department of Brain-Computer Interfaces, Research Institute for Translational Medicine,Pirogov Russian National Research Medical University, Moscow, Russia
3 Department of Biochemistry, Faculty of Biology,Lomonosov Moscow State University, Moscow, Russia
Correspondence should be addressed: Vsevolod Belousov
ul. Miklukho-Maklaya, d. 16/10, Moscow, Russia, 117997; ur.hcbi@vosuoleb
Funding: this work was supported by the Russian Foundation for Basic Research (Grant 16-34-60175) and the Russian Federation Presidential Grant MK-6339.2016.4. Experiments were partially carried out using the equipment provided by the IBCH сore facility (CKP IBCH, supported by Russian Ministry of Education and Science, grant RFMEFI62117X0018).
The zebrafish Danio rerio is a popular model species for in vivo biomedical research. It has a few advantages over other lab animals: fish are prolific breeders that require little upkeep and develop rapidly. Due to their optical clarity, Danio rerio embryos are a good object for microscopy. Besides, this fish’s genome is very well studied and hence easy to manipulate. Therefore, D. rerio are often used to study gene functions and developmental biology of vertebrates. Because cell biochemistry and functional activity of proteins in D. rerio resemble those of mammals, this species is used for modeling human diseases, testing toxicity of chemical compounds in preclinical trials, and in therapeutic drug screening.
In the last few years D. rerio has been successfully exploited as a model organism to study acute lymphoblastic leukemia [1 – 3], melanoma [4, 5], muscular dystrophy [6], diabetes mellitus [7], pathological conditions of the heart [8 – 10], kidneys [11, 12], and central nervous system [13, 14], including brain ischemia [15, 16]. Such vertebrate models are handy when it comes to investigating effects of various chemical compounds on the organism in health and pathology. The easiest way to administer a compound of interest to the fish is to add it into the water in the tank: the chemical will enter the fish’s bloodstream through the gills. The majority of experiments are carried out in larval fish, though, meaning that drugs are normally injected straight into the yolk sac. This method of drug delivery can be employed to assess cardiotoxicity of aspirin, clomipramine, nimodipine, varapamil and some others medications [17, 18]. Larval fish are also used to study metabolites that are part of normal mammalian biochemistry. For example, in one of the experiments excess amounts of glucose were administered to fish embryos via injections to describe expression profiles of genes involved in carbohydrate metabolism [19]. It is also possible to infect D. rerio larvae with injections of bacterial cells to observe how infection progresses [20 – 22] or even mammalian cells to study tumor growth [23, 24].
Genetically encoded biosensors based on fluorescent proteins have taken in vivo studies of physiology and pathology to a new level. Each biosensor is a chimeric protein molecule consisting, as a rule, of a sensing moiety and a fluorescent domain. The sensor domain detects changes in the surrounding environment, such as fluctuations in the intracellular concentrations of a studied compound. The fluorescent component “reports” interactions between the sensing domain and the compound of interest, and the obtained signal is then interpreted. Such biosensors are encoded by a gene that can be incorporated into any living organism. What is important is that this approach can be used in real-time imaging of biochemical processes in a living organism [25]. Oftentimes genetically encoded biosensors are the only available tool for looking into complex biological events. Fluorescent biosensors have been extensively used in model D. rerio species to study embryogenesis [26], inflammation [27], and organ regeneration [28].
In this work we have pioneered the use of the genetically encoded biosensor SoNar [29] as a tool for monitoring the redox state of nicotinamide adenine dinucleotide (NAD) in the cytoplasm of D. rerio tissues. The ratio of its oxidized to reduced forms (NAD+/NADH) is critical not only for cell metabolism but also for the regulation of many signaling cascades [30 – 32]. We have found out that the NAD+/NADH ratio changes both in the yolk sac and body of a D. rerio embryo following lactate and pyruvate injections.
The SoNar biosensor [29] is based on the bacterial protein T-Rex of Thermus aquaticus that regulates transcription of a few enzymes in response to fluctuations in the intracellular NAD+/NADH ratio. One of the biosensor’s components is the fluorescent protein cpYFP integrated into the mobile region of T-Rex. Conformational changes accompanying the binding of T-Rex to NAD+ or NADH are propagated to the fluorescent protein affecting its spectral properties (fig. 1). SoNar fluorescence excitation spectrum typically has two peaks at 420 and 490 nm; the sensor emission peak is at 518 nm. The signal is calculated as a ratio of fluorescence intensities excited at 420 nm and 490 nm (F420/F490) [29]. Thus, the signal is ratiometric, which is particularly important for in vivo research, as it helps to avoid artifacts related to different expression levels of the sensor in different cells, motion, changes of the object’s shape or tissue thickness.
METHODS
AB/TL Danio rerio fish were kept in a specially equipped room at 26.5 °С under 12:12 light conditions. The experiments were conducted in compliance with the Declaration of Helsinki. To obtain progeny, parent fish were paired in separate tanks containing 500 ml of E3 medium 4 hours before the lights went out. Eggs were collected when the lights were turned back on.
In our experiment we used SoNar [29] and SypHer-2 [33] biosensors. To obtain larval D.rerio fish expressing these biosensors in their tissues, we synthesized biosensor mRNA in vitro using the commercial mMessage mMachine SP6 Transcription kit (Ambion, USA). Then 1 nl of 0.1 μg/μl mRNA was injected into the yolk sac of single-cell fish embryos using Eppendorf Microinjector 5242. The treated fish were kept in Petri dishes at 26.5 °С under standard 12:12 light conditions. Twenty four hours after the injection, the embryos were examined under the fluorescence microscope to select those that were fluorescent. Before microscopy the embryos were manually stripped of chorions, immobilized in 0.02 % tricaine solution and mounted in a drop of 1.5 % low-melting point agarose. One nl of 200 mM sodium lactate and sodium pyruvate solutions was injected in the yolk sac of each immobilized embryo. Prior to the injections of metabolites, biosensor signals were recorded in the yolk sac and body of each larval fish.
Microscopy was performed using the wide field fluorescence microscope Leica DM6000 (Leica, Germany), equipped with the HC PL FLUOTAR 10.0*0.30 DRY lens. Fluorescence was excited using excitation filters CFP BP436/20 and GFP BP470/40. Frame rate was 1 frame per minute. Images were processed in ImageJ (NIH). Graphs showing the dynamics of sensor signals were constructed using OriginPro 8.6 (OriginLab, USA). The same software was used to process data obtained at each time point.
RESULTS
We have tested the use of the genetically encoded sensor SoNar [29] in monitoring the NAD+/NADH ratio in D. rerio tissues. Briefly, we synthesized biosensor mRNA and injected it into the yolk sac of single-cell fish embryos. Twenty four hours after the injection we selected fluorescent embryos for further microscopy. SoNar fluorescence was recorded in two independent channels; fluorescence excitation wavelengths in each channel matched the two peaks of SoNar’s fluorescence excitation spectrum (fig. 1). We obtained two images of each fish embryo at 420 nm (F420) and 490 nm (F490) excitation. Using ImageJ we divided the images by one another; the resulting image was then painted in pseudocolors corresponding to the values of the F420/F490 ratio (fig. 2, A, D). Again, using ImageJ we selected a few zones on the embryo’s body and made further calculations. Graphs showing the dynamics of the F420/F490 ratio were constructed in OriginPro 8.6.
To monitor the dynamics of the NAD+/NADH ratio in the embryo tissues of D. rerio using the SoNar biosensor, we injected sodium lactate and pyruvate into the embryos. It is well known that the lactate/pyruvate pair is in equilibrium with free cytoplasmic NAD+/NADH owing to the reaction catalyzed by lactate dehydrogenase [34, 35]. Bearing that in mind, we injected 1 nl of 200 mM sodium lactate solution in the yolk sac of 10 embryos and 1 nl of 200 mM sodium pyruvate solution in the yolk sac of another 11 embryos. Prior to the injections, we recorded the signal emitted by the biosensor in the untreated tissues. This initial value was taken as 1. After the injection, the fish were quickly put under the microscope and imaging was resumed with the same settings. Embryos injected with lactate demonstrated an increase in the F420/F490 ratio, indicating reduction of the NAD pool (fig. 2, B). Similar signal dynamics observed in different parts of the embryo’s body can be explained by rapid delivery of the metabolites from the yolk sac to other organs. The signal reached its maximum 5 min after the injection, gradually losing its intensity for the next 30 min until it finally reached its initial value, while lactate was being metabolized by cellular organelles. We expected to see an opposite effect with pyruvate. But the F420/F490 ratio decreased only in the yolk sac and even slightly increased in the embryo’s body (fig. 2, E). It means that small doses of pyruvate trigger different redox events in the intracellular NAD pool: oxidation in the yolk sac and reduction in the embryo’s body.
Lactate or pyruvate transport into the cells can happen against the background of changing pH because it occurs in symport with Н+ [36, 37]. The specific chromophore structure makes fluorescent proteins sensitive to pH fluctuations [38]. To assess a possible effect of pH on the SoNar signal intensity, we used SypHer2 previously developed in our lab as a control biosensor [33]. We synthesized SypHer2 mRNA and conducted the same series of experiments as with SoNar. Lactate and pyruvate injections did not induce any changes in pH in the embryos’ bodies. Injected into the yolk sac, both metabolites caused a slight and slow increase in pH in this tissue which lasted for 20 min following the injection (fig. 2, C, F).
DISCUSSION
Our findings lead us to conclude that the genetically encoded biosensor SoNar developed to record NAD+/NADH fluctuations can be successfully used in the experiments involving D. rerio model species. The NAD+/NADH ratio is an important biological parameter that can be significantly affected by pathology. A fish that expresses the SoNar biosensor in its tissues or cells can be effectively used as a model organism to assess the impact of different compounds on the NAD+/NADH ratio and therefore on disease progression.
We have found out that lactate injections in the yolk sac cause significant simultaneous reduction of the NAD pool in all tissues of the fish. However, pyruvate injections induce NAD oxidation only in the yolk sac; moreover, they cause slight reduction in the embryo’s body. One of the recent works studied the role of redox processes in embryogenesis using the D. rerio model to reveal that massive oxidation starts at gastrulation and goes on to subside when the fish is three days old. That work demonstrates that oxidative stress plays a key role in the development of some organs in the course of embryogenesis [26]. Perhaps, we have not observed significant oxidation in embryo tissues following the pyruvate injection because at this developmental stage redox homeostasis is shifted towards oxidation. Notably, in the article cited above the minimum level of reactive oxygen species (hydrogen peroxide) was observed in the yolk sac – the organ in which (in our experiment) NAD tended to be oxidized after the injection of pyruvate. Insignificant reduction of the NAD pool in the embryo’s body is probably compensation.
Another possible explanation is that in the yolk sac pyruvate is reduced to lactate, which can be confirmed by decreased NADH concentrations, but it is mainly lactate that is transported to the embryo’s body. Perhaps, this is why we observed a signal change indicative of a slight elevation of NADH concentrations. This hypothesis needs to be tested.
CONCLUSIONS
We have demonstrated that the genetically encoded biosensor SoNar developed to measure the NAD+/NADH ratio can be used in D. rerio tissues. Lactate injections into the yolk sac of a one-day old fish embryo lead to rapid reduction of the NAD pool in all tissues of the fish, showing that substances present in the yolk sac can be easily transported to other tissues. Pyruvate injections induce oxidative reactions in the yolk sac only, accompanied by slight reduction in the embryo’s body. Using the control SypHer2, we have established that pyruvate and lactate cause slight pH fluctuations only in the yolk sac.