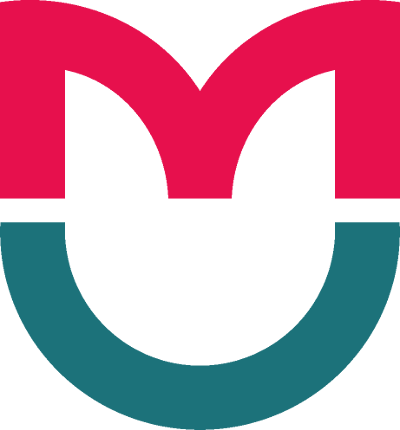
This article is an open access article distributed under the terms and conditions of the Creative Commons Attribution license (CC BY).
ORIGINAL RESEARCH
Cerebral cortex activation during the Sternberg verbal working memory task
1 Research Center of Neurology, Moscow, Russia
2 Skoltech, Moscow, Russia
Correspondence should be addressed: Ilya S. Bakulin
Volokolamskoye shosse, 80, Moscow, 125367; ur.ygoloruen@nilukab
Author contribution: Bakulin IS, Fedorov MV, Gnedovskaya EV, Suponeva NA, Piradov MA — study planning and design; Bakulin IS, Zabirova AH — literature analysis; Bakulin IS, Poydasheva AG — data acquisition; Zabirova AH, Kopnin PN, Sinitsyn DO — data analysis; Bakulin IS, Zabirova AH, Sinitsyn DO, Fedorov MV, Gnedovskaya EV, Suponeva NA, Piradov MA — data interpretation; Bakulin IS, Zabirova AH — manuscript draft preparation; Kopnin PN, Sinitsyn DO, Poydasheva AG, Fedorov MV, Gnedovskaya EV, Suponeva NA, Piradov MA — manuscript draft editing; all the authors — final version of the article preparation.
Working memory is the ability to maintain and process a limited amount of information for a short time for its use in the ongoing cognitive activity. The working memory decline is associated with normal aging [1], it is also observed in patients with various neurological and psychiatric disorders [2], that confirms the importance of the working memory study.
Research data regarding working memory mechanisms remain controversial [3]. One of the brain areas, most investigated in context of working memory, is the dorsolateral prefrontal cortex. The persistent neural activity during the maintenance period of the working memory task was firstly observed in the prefrontal cortex of primates [4]. Later, the similar activity was detected in other regions. However, a number of studies confirmed the importance of the persistent neural activity in prefrontal cortex. For example, despite the simultaneous activation of prefrontal and temporal cortex during the task, inactivation of prefrontal cortex leaded to the inhibition of persistent neural activity in the temporal cortex of primates, while inactivation of the temporal cortex did not affect the prefrontal cortex [5]. The temporary inactivation of the prefrontal cortex led to a more pronounced working memory decline than inactivation of the posterior parietal cortex [6].
The role of the dorsolateral prefrontal cortex in the working memory in humans was confirmed by fMRI with different tasks (n-back, delayed match-to-sample task, Sternberg task, etc.) [7–9], as well as by transcranial magnetic stimulation (TMS) [10]. However, many cerebral cortex regions are activated during a working memory task: parietal lobe (areas around the intraparietal sulcus, superior parietal lobule), insula, anterior cingulate gyrus [7, 11, 12], visual cortex [13]. At the same time, the question of the link between activation and working memory mechanisms (information maintenance and manipulation) remains open, since activation can be caused by additional cognitive processes involved in the working memory task performance, for example, the perception of a stimulus [13]. The use of the delayed response tasks (the Sternberg task in particular) [14] allows to separate some working memory processes by analyzing different task stages: encoding, maintenance and retrieval.
The assessment of temporal dynamics of activation can be helpful in comparison of fMRI results with the studies using causal methods (for example, TMS) where the online effects at the different stages of the task execution were studied. Given the increasing interest in the use of non-invasive brain stimulation techniques for the improvement of cognitive functions [15, 16] the issue is also important for clinical neurology. fMRI results can help in determination of the TMS target which might increase the stimulation effectiveness [17]. The data of the cortical activity dynamics might also be used for combination of TMS protocols with cognitive load.
Despite the frequent use of the Sternberg task in neuroimaging studies of verbal working memory [11], the analysis of activation at different time was performed in a relatively small number of studies [9, 18–20]. Considering the limitations caused by the fMRI temporal resolution, the reproducibility of the results of such studies, which is necessary to confirm the possibility of using fMRI for the investigation of individual working memory mechanisms, still remains important.
The study was aimed to determine the activation of cerebral cortex regions at the different stages of the modified Sternberg task for verbal working memory, and to assess the dynamics of individual regions activation by the analysis of temporal dynamics of BOLD signal changes. The following cerebral cortex regions were chosen for assessment (according to their potential functional role in the Sternberg task performance, including participation in the working memory processes): left dorsolateral prefrontal cortex [9, 10], left posterior parietal cortex [7, 11, 21] and left occipital cortex [13].
METHODS
Study participants
The study was performed in 2017–2019 at the Research Center of Neurology.
Inclusion criteria: age 18–55; dominant right hand (according to the Edinburgh Handedness Inventory [22]); normal (corrected to normal) vision. Exclusion criteria: contraindications for MRI [23]; neurological or psychiatric disorders; intake of drugs that affect the central nervous system at the time of the study; severe chronic somatic disorders.
In accordance with the inclusion criteria, 19 healthy volunteers (6 men) aged 22-31 were recruited, all of them right-handed. The study was performed as a part of the project “Effects of high-frequency repetitive transcranial magnetic stimulation over the left dorsolateral prefrontal cortex on working memory in healthy volunteers”.
fMRI with the Sternberg task
fMRI with the modified Sternberg task for verbal working memory was used in the study [9, 14]. The participants received instructions about all stages of the investigation before performing the task in the MRI scanner. During the investigation, participants were wearing headphones to reduce the scanner noise. The participants could see the task screen located in the scanning room through a mirror, which was installed on the 8-channel head coil. Hand-held grips with buttons were given to each participant, which they pressed according to the instructions.
Task-fMRI used in our study had a block design and consisted of 24 task presentations (fig. 1). Each presentation included the activation period (the Sternberg task) and the rest period. The activation period was divided into three blocks (periods): encoding, maintenance and retrieval. During the encoding period (1st–3rd seconds from the start of the task) the stimulus (7 latin consonants selected in random order using the algorithm created in the MATLAB R2017a (Mathworks, Natick, MA; USA)) was shown on the screen. Angular size of the letters was 1.5. During the maintenance period (4–12th second) the participant maintained the stimuli in memory, while the screen remained blank (without fixation point). During the retrieval period (13–15th second) a single latin letter appeared on the screen. The participant was instructed to press a button in the right hand-held grip if the letter presented during the retrieval period matched to the sequence, and a button in the left hand-held grip if the letter had not. Matching and non-matching stimuli were presented in random order, with 12 presentations of each stimulus type. The duration of activation period, as well as of the rest period without any stimulus (black screen), was 15 seconds. Participants were instructed to keep their eyes open during all periods. The experiment duration was 12 minutes.
Image acquisition and preprocessing
The study was performed using a 3 T scanner Magnetom Verio (Siemens; Germany). Anatomical data were acquired using the 3D-T1-gradient echo (T1-MPR) MRI sequence and consisted of 176 sagittal slices with the following parameters: TR 1900 ms, TE 2.47 ms, slice thickness 1.0 mm, distance factor 50%; voxel size 1.0 · 1.0 · 1.0 mm3, field-of-view 250 mm. The functional data were acquired by investigation of the BOLD signal changes using T2*-gradient echo MRI sequence with the following parameters: TR 3000 ms, TE 30 ms, slice thickness 3 mm, distance factor 25%, voxel size 3.0 · 3.0 · 3.0 mm3, field-of-view 192 mm, 36 axial slices. To ensure dynamic magnetic equilibrium, first five images were excluded from the analysis. Preprocessing and statistical analysis were performed using the SPM12 software (Institute of Neurology, London; UK) based on the MATLAB R2017a (Mathworks, Natick, MA; USA).
Image preprocessing included following stages: 1) image realignment for correction of motion artifacts using the shifting and rotation transformations; 2) co-registration of anatomical and functional data; 3) segmentation of the obtained structural images and their spatial normalization to transform the individual images into the Montreal Neurological Institute (MNI) space; 4) spatial smoothing with a Gaussian kernel 8 mm FWMH to reduce the impact of anatomical variability.
The low-frequency drift was removed using the high-pass filter (time constant 128 s).
Statistical analysis
Statistical parametric mapping was performed using general linear models, estimated separately for the signal of each voxel. During the 1st level analysis, individual activation data were obtained for the encoding, maintenance and retrieval periods. The last 9 seconds of the rest period (22–30th seconds) were used as the control state for the activation level calculation. Head motion parameters were included as nuisance regressors. The data of 19 participants were included in the group analysis (separately for each period). Activation was assessed both at peak and cluster levels. The threshold for the inclusion of an individual voxel in the clusters was set at р < 0.0001. The clusters in the gray matter of cerebral hemispheres and cerebellum were selected for the analysis with a significance level of p < 0.05 (with the family-wise error, FWE correction for multiple comparisons ).
For regions showing significant activation during the maintenance period, the additional comparison with activation of the frontoparietal control network (FPCN) was performed, according to the resting state fMRI data [24]. The results were visualized using xjView [25] through overlay of the FPCN from the atlas [24] with the activation map of the maintenance period.
The temporal dynamics of the changes in BOLD signal intensity was analyzed in three regions of interest: left dorsolateral prefrontal cortex, left posterior parietal cortex and left occipital cortex. To determine the specific regions that were active during the task performance , the difference between the signal intensity in the maintenance period and the rest period was used as contrast within the selected regions of interest. Voxels with activation above the threshold were selected in the regions of interest (p < 0.0001). Activation clusters containing selected voxel and restricted to spheres with the centers in a voxel with locally maximal activation were identified on group data. Radii were selected manually to obtain the region of intersection of the sphere and cluster with a sufficient number of voxels without spreading to adjacent anatomical regions (7 mm for dorsolateral prefrontal cortex, 8 mm for posterior parietal cortex, 10 mm for occipital cortex). At the individual level, BOLD signal was extracted from voxels in the determined cluster, as well as a signal adjusted for alignment effects and temporal non-sphericity in the framework of the autoregressive process AR(1). The extracted and simulated signals were averaged over the cluster voxels, and then over all participants. After that, the signal was averaged over the 24 task presentations and interpolated with periodic boundary conditions by the fast Fourier transformation method with a step of TR/10 (equal to 0.3 s). By the interpretation of the data, 5 s were subtracted from the onsets of BOLD events to account for the hemodynamic response delay.
RESULTS
The activation of the cerebral cortex regions at different stages of the modified Sternberg task is shown in fig. 2.
During the encoding period significant activation was detected in the clusters within the right and left parietal lobes (supramarginal and postcentral gyri, superior parietal lobule), and also in the cerebellar cortex and occipital lobe (cuneus) bilaterally. Activation was observed in the right middle frontal and superior frontal gyri, and in the lower part of the left middle frontal gyrus spreading to the inferior frontal gyrus. A cluster of activation was also detected in the left rolandic operculum (Table 1;, fig. 2А).
During the maintenance period significant activation was found in the medial parts of the frontal lobes (bilaterally), prefrontal cortex (right middle frontal gyrus , left middle frontal and inferior frontal gyri), parietal lobe (supramarginal gyrus and superior parietal lobule bilaterally, right angular gyrus). In addition, activation was detected in the anterior parts of the insula (bilaterally) and in the left prefrontal cortex (middle frontal gyrus) (Table 2;, fig. 2B).
Comparing the activation pattern in maintenance period with the FPCN, a partial overlap of the activation zones in the parietal and frontal lobes was noted, as well as in the medial parts of the frontal lobes and insular cortex (bilaterally) (fig. 3).
During the retrieval period the regions of significant activation were detected in the right medial orbital and anterior orbital gyri, right middle temporal gyrus, in the cerebellar hemispheres bilaterally (spreading to the fusiform gyrus on the left). A cluster of activation was also detected in the right anterior insula and in the operculum. Within the parietal lobe clusters of activation were found in the right supramarginal gyrus, as well as in the left postcentral and supramarginal gyri. A cluster of activation was also identified in the right middle frontal gyrus. Activation was detected in the cerebellar vermis spreading to the lingual gyri bilaterally (Table 3;, fig. 2C).
A cluster of activation in the left middle frontal gyrus at the intersection with a sphere with a radius of 7 mm and center at the point of maximal activation [–36; 47; 5] (according to MNI space) containing 21 voxels was selected as the region of interest, in which temporal change of BOLD signal intensity was analyzed. According to the analysis results, an increase in signal intensity in the left middle frontal gyrus begins at the beginning of the task. The activation curve contains two peaks, 6 and 15 seconds from the beginning of the block. After the latter the signal intensity gradually decreases and reaches a plateau (fig. 4).
The temporal change of BOLD signal intensity was analyzed for a cluster in the left inferior parietal lobule at the intersection with a sphere with a radius of 8 mm and the center at the point of maximum activation [–42; –49; 54] (according to MNI space) containing 23 voxels. The temporal pattern of the BOLD signal intensity change in this region is similar to the curve obtained for the dorsolateral prefrontal cortex. It contains two peaks, 6 and 18 seconds from the beginning of the block. Between the peaks, a decrease in activation was observed with a local minimum at 13 seconds from the beginning of the block. Similar to the left dorsolateral prefrontal cortex, an increase in signal intensity begins with the beginning of the block, and the minimum is detected 24–29 seconds from the beginning of the module (fig. 4).
In the left middle occipital gyrus a cluster of activation was selected at the intersection with a sphere with a radius of 10 mm and the center at [–24; –82; 1] (MNI space), containing 33 voxels. In this region of interest an increase in the BOLD signal intensity is observed from the beginning of the task. The maximum is observed 5–6 seconds after the beginning of the block, then the signal level decreases. The minimum is registered 11 seconds from the beginning followed by a slight increase in signal intensity with a subsequent decrease in activation (fig. 4).
DISCUSSION
In this study, the activation in regions of cerebral cortex at different stages of the modified Sternberg verbal working memory task was assessed in 19 healthy volunteers using fMRI. Temporal pattern of changes in the left dorsolateral prefrontal, left posterior parietal and left occipital cortex activation was analyzed. During the encoding and retrieval periods activation in prefrontal cortex was observed (bilaterally and in the right hemisphere, respectively), as well as bilateral activation in parietal cortex, cerebellar hemispheres and occipital cortex. During the maintenance period the activation in FPCN components was found: symmetrical regions of the prefrontal and posterior parietal cortex, insular cortex, and medial parts of the superior frontal gyrus. The maximal activation of the left prefrontal and parietal cortex was observed during the encoding period and at the end of the maintenance period, between them it remained relatively constant, while for the occipital cortex, the peak of activation matched to the encoding period. Thus, according to the results of fMRI analysis, activation of prefrontal and posterior parietal cortex (components of FPCN) was detected during maintenance of verbal information in working memory.
Regions of activation in our study were generally consistent with the data of other researchers who used a similar modification of the Sternberg task for verbal working memory assessment [9]. Activation of the upper and lower parts of the left prefrontal cortex , parietal cortex bilaterally and the occipital cortex was observed during the encoding period. However, in our study, we detected additional activation in the right prefrontal cortex and the cerebellar hemispheres (bilaterally). In addition, activation of the parietal cortex was more prominent, and the activation region in the visual cortex was relatively small. During the maintenance period we detected activation regions in the parietal and prefrontal cortex bilaterally, while in the study described above the activation was observed mainly in the left hemisphere. At the retrieval period the regions of activation in the parietal cortex on the left and bilateral regions of the operculum are consistent. Compared to [9], additional regions of activation were found in the right parietal cortex and the temporal lobe, but no activation of the left prefrontal cortex was observed. This difference could be due to the different task complexity (the researchers [9] used sequences of 4–6 letters, unlike 7 letters in our study) or due to the different duration of the encoding, maintenance and retrieval periods (2.16; 6.48 and 2.16 seconds respectively [9]). The differences could also result from the different task performance strategies: the activation pattern in the maintenance period of our experiment was similar to the activation detected during the working memory task performance with articulatory suppression [26], which could indicate the use of non-articulatory maintenance mechanisms by participants.
Activation of clusters in the functionally different regions during the encoding and maintenance periods can be explained by the combination of several types of activity: visual information processing, subvocal speaking, choosing the strategy of maintenance, as well as attention and motor activity. Thus, during the encoding period, significant activation of the occipital cortex regions can be observed, associated with the visual information processing [9]. The activation of left inferior frontal gyrus region at encoding period might be associated with the verbal information retention [12]. Bilateral activation of parietal cortex at the same time with activation of the inferior frontal gyrus is consistent with the preferential activation of dorsal attention network (DAN) during the encoding period [27, 28]. Activation of a region in the cerebellar hemisphere has also been shown in a meta-analysis and is consistent with the hypothesis that the right cerebellar hemisphere is involved in the verbal working memory [11].
The activation of FPCN components was revealed during the maintenance period [24], which is consistent with the data of a meta-analysis demonstrating a gradual increase in FPCN activity and a decrease in DAN activity from the encoding period to the maintenance period in delayed response tasks [27]. At the same time, a decrease in the total number of activation regions during the maintenance period compared to encoding and retrieval may be associated with fewer types of activity involved (only maintenance of information).
During the retrieval period, activation was detected in the parietal lobes bilaterally with the predominance of the right one, which might be due to attention processes [13, 29]. Activation of the right middle temporal gyrus may be due to attention switching [29]. As in the encoding period, activation of the occipital cortex might be associated with visual perception. Observed activation in the right anterior insula is consistent with the evidence of their control function [28]. As opposed to the meta-analysis results [27], in our study, the activation of both FPCN and DAN components has been confirmed in the retrieval period. Activation of the right prefrontal cortex during the retrieval period with presentation of a verbal stimulus emphasizes the ambiguity of the idea about the lateralization of the regions with a functional role in working memory.
According to the results of the BOLD signal changes analysis with a correction for the hemodynamic response delay, activation of the left dorsolateral prefrontal cortex achieves its maximum in the middle of the encoding period. Then, during the maintenance period it experiences a slight decrease remaining relatively high, after that it increases slightly at the end of the maintenance period and decreases during the retrieval period (neural events in the range of 12–15 seconds from the beginning of the task). This is mostly consistent with the data of other researchers [9, 18, 20] and the hypothesis of the importance of persistent neural activity in working memory [4]. Compared to dorsolateral prefrontal cortex, the parietal cortex activation decreases more strongly in the middle of the maintenance period. Then it experiences a slight increase with a maximum at the beginning of the retrieval period, which is consistent with the results of another study and is especially characteristic for the high-load Sternberg task (6–9 letters) [18]. On the contrary, the occipital cortex activation achieves its maximum during the encoding period and then decreases, which suggests its role in the processes of visual stimulus perception, but not the working memory itself [9, 13, 18].
When interpreting the results, it is necessary to take into account the limitations of the method used to determine the task stages, during which every brain region is active. Since the task periods follow each other in a fixed order, it is impossible to separate the effects of each state on the BOLD signal completely using a model based on the canonical hemodynamic response function, in the absence of detailed information about this function for each subject and brain region. A number of approaches has been proposed in the literature to reduce the impact of this problem, but has not completely solved it [20, 30]. Other limitations include different individual task performance strategies, which could affect the group analysis. The short duration of the encoding and retrieval periods compared with the rest period should also be noted.
CONCLUSION
The study revealed the activation of prefrontal and posterior parietal cortex (parts of the frontoparietal control network, FPCN) in healthy volunteers during the verbal working memory delayed response task. The results are consistent with the ideas about the key role of prefrontal cortex in the working memory mechanisms of information maintenance, while during the encoding and retrieval periods predominant activation of the parietal cortex was found. Despite the limitations of the method, the study results are consistent with the results of other research using the Sternberg task for the investigation of visual and verbal working memory by fMRI, which speaks in favor of the reproducibility and possible use the results to study the distinct working memory mechanisms.