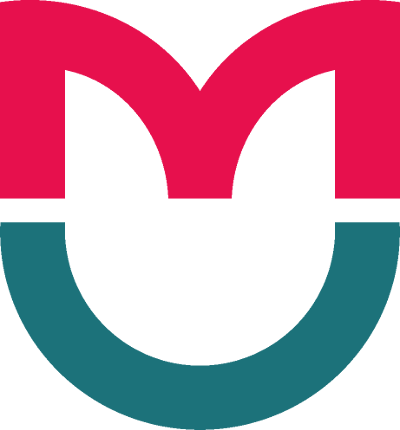
METHOD
Molecular and cellular features of mandibular autografts studied using raman spectroscopy
1 Lomonosov Moscow State University, Moscow, Russia
2 Pirogov Russian National Research Medical University, Moscow, Russia
3 A. I. Yevdokimov Moscow State University of Medicine and Dentistry, Moscow, Russia
4 National University of Science and Technology MISIS, Moscow, Russia
5 Central State Medical Academy of Department for Presidential Affairs of the Russian Federation, Moscow, Russia
Correspondence should be addressed: Georgy V. Maksimov
Leninskie Gory, 1, str. 24, Moscow, 117042; ur.liam@vomiskamg
Author contribution: Maksimov GV — study planning, analysis of the results; Sashkina TI — study planning, literature analysis; Fashutdinov DK — data acquisition and analysis, performing bone grafting; Slatinskaya OV — data processing; Saldusova IV — data analysis; Zaychenko OV — technical support.
Compliance with ethical standards: the study was approved by the Ethics Committee of the Central State Medical Academy of Department for Presidential Affairs of the Russian Federation (protocol № 3 dated September 23, 2021); the informed consent was submitted by all participants; biomaterials were treated in accordance with the World Medical Association Declaration of Helsinki.
It is known that dental implants are being successfully used to restore the dental arch continuity in partially and completely edentulous patients [1, 2]. The patients of the dentists often present with significant bone loss in the alveolar process and a part of the jaw, which suggests the procedure to increase the amount of bone tissue prior to implant installation. Bone augmentation is performed using both allo- and autografts, however, it is better to use autografts [3, 4]. Autogenous cortical bone grafts are good for that, harvested from various extraoral body areas: iliac crest, skull cap, tibia, zygomatic bone, as well as the intraoral sites (rami of the mandible, sites within the retromolar region, chin area). Intraoral autogenous bone grafts are used more frequently, since the harvesting procedure is less invasive, surgical approach is more convenient, and the installation procedure is less time-consuming. It is also essential that the distance between donor and recipient sites becomes closer [5, 6].
Osteoregeneration process depends on a number of factors: microarchitectonics, bone density, percentage of cortical and cancellous bone, as well as the levels of pro- and antiinflammatory cytokines, the markers of bone destruction and regeneration [7–10]. It is well-known that jaws are flat cancellous bones organized into trabeculae. Trabeculae form the shell for red bone marrow, which is responsible for hematopoiesis and blood cell production [11]. Type 1 collagen accounts for 95% of trabecular plate; trabecular plates also contain collagen types 3, 4, 5, 11, and 12 (the other 5%). There are noncollagenous proteins, osteocalcin, osteonectin, osteopontin, bone sialoproteins, phosphoproteins, morphogenetic proteins, and proteoglycans in the intercellular space. Moreover, there are glycoproteins: alkaline phosphatase, osteonectin, thrombospondin, fibronectin, vitronectin, osteopontin, sialoprotein, Wht-glycoproteins. Inorganic components, represented by the hydroxyapatite crystals, account for 30% of bone tissue structure. Bone also contains hydroxyapatite stability regulators: magnesium, strontium and manganese.
Cellular composition of bone tissue consists of osteoprogenitor cells (early progenitors of osteoblasts), osteoblasts, osteocytes, bone lining cells, and osteoclasts. Mature osteoblasts produce type 1 collagen, proteoglycans, and osteocalcin. Immature osteoblasts lay directly adjacent to periosteum. The cytoplasm of those contains small quantities of glycogen granules. Osteoblasts are cells that synthesize bone matrix, cytokines, and growth factors. Alkaline phosphatase and osteocalcin are the osteoblast markers (osteocalcin is the main marker of bone tissue regeneration). Osteocyte formation is the final stage of osteoblast differentiation. Osteocytes do not synthesize bone matrix; osteocytes are involved in osteolysis and metabolic transport. Osteoclasts, being the large multinucleated cells, are responsible for bone resorption. Osteoclasts priduce hydrogen ions together with enzymes (cathepsin and collagenase) involved in the lysis of organic matrix. Alkaline phosphatase is the marker of osteoclasts. The outer surface of spongy osseous tissue is covered by cortical bone, which in turn is covered by periosteum, invaded by blood vessels feeding the bone. The internal layer of periosteum contains osteoblast progenitor cells responsible for bone growth and remodeling [11]. Studying the structure and components of bone tissue is essential for evaluation and forecasting of regeneration, osteogenesis and osseointegration when performing dental implantation [12].
Clearly, it is important to put advanced effective, reliable and non-invasive optical methods, allowing one to allocate the components of bone tissue, oral fluid, and peripheral blood, into dental practice in order to monitor bone tissue structure, resorption and remodeling. Assessment of bone-to-implant integration and augmentation of the alveolar processes is relevant to surgical dentistry, and is of crucial importance, since it determines the success of treatment.
Prompt diagnosis of osseointegration and bone tissue regeneration impairments is essential. Markers of resorption and regeneration, as well as bone tissue protectors, are well-known. There is a number of methods for detection of those. Biochemical analysis makes it possible to evaluate the treatment and complication prevention results, however, the analysis requires a fairly large amount of the material being sampled. Gas chromatography is a more accurate method, which has the advantage of high reliability of the results. The method’s disadvantages are as follows: considerable complexity of investigation, high cost, time-consuming interpretation of the results, and the need for expert assessment.
In recent years, many optical methods have been used in biology and medicine. One of the methods is Raman spectroscopy, the highly sensitive optical method providing express and accurate determination of the composition and conformation of molecules, constituting biological objects: cells, cell fragments, bacteria, viruses, proteins, peptides, lipids [13, 14]. It is worth mentioning that in dentistry there is an emerging body of research using both Raman spectra and giant Raman spectra acquisition [15]. Such approach exhibits high sensitivity; it has been used for identification of the main pathogens of purulent-inflammatory processes in maxillofacial area [16]. What's important is that with the use of giant Raman scattering effect, certain markers of the pathogens have been detected: in Bacillus subtilius there were peaks at 657, 726, 1248, 1377, 1466, 1617 cm–1; in E. coli these were found at 1140, 1551 cm–1; in S. aureus peaks were found at 959, 1006, 1160, 1284, 1530 см–1; in S. haemolyticus there were peaks at 1327, 1369; in Ps. aeruginosa these were found at 675, 1353, 1404, 1605, 1630 cm–1. Thus, giant Raman spectra acquired during treatement make it possible to determine the cause of the inflammation. However, this approach needs firther detailed testing in practice, since the giant Raman scattering effect realization requires the use of nanoparticles (colloidal silver and gold nanoparticles), the toxic effect of which on the cells and tissues of the oral cavity is not yet khown.
Taking into account the informative nature and efficiency of Raman spectroscopy, as well as lack of time-consuming sample preparation, it is important to develop the Raman scatteringbased technology for assessment of osseointegration after installation of dental implants in order to define the condition of autograft bone, as well as to search for markers of osteogenesis, resorption and osseointegration involved in the process of reparative regeneration.
The study was aimed to investigate molecular and cellular composition of mandibular autografts using Raman spectroscopy.
METHODS
Augmentation of the mandible prior to implant installation was performed during the study. For this purpose the patients underwent surgical restoration of bone tissue amount using autografts, the condition of which was investigated later. Four samples of cortical and cancellous bone tissue obtained from the left and right retromolar regions of the mandible during the surgical procedure were assessed (see table). Four autografts were investigated, obtained from three patients aged 51–73: two men and one woman. Inclusion criteria: no somatic pathology; medium-sized mandibular bone defect; no drug intolerance; age 40–60 years. Exclusion criteria: severe somatic illness; drug intolerance; large-sized mandibular bone defect; age under 40 and over 60.
Bone block harvesting from the donor site
After giving the conduction and infiltration anesthesia (sol. Articaini 3.4 mL), the incision was made across the mandibular alveolar process apex in the area of missing teeth, mucoperiosteal flap was peeled off, mandibular alveolar bone was exposed, and the length and width of the bone defect were defined. After giving the conduction and infiltration anesthesia (sol. Articaini 3.4 mL), the 3 cm incisions were made in the left and right retromolar regions, mucoperiosteal flap was peeled off. Using the disc with a protector and the fissure burr, autograft sized 2 × 1 × 0.3 cm or 1.5 × 1 × 0.3 cm was harvested. The autograft bone was split into two thin bone plates using the disc. The wound was closed by the 4–0 Vicryl suture.
The autograft bone samples (size 0,1 mm) were placed into glass capillaries with cross sectional diameter of 1 mm (Agat-Med; Russia) filled with buffer (145 мМ NaCl, 5 мМ KCl,
4 мM Na2HPO4, 1 мM NaH2PO4, 1 мМ MgSO4, 5 мМ glucose (Sigma; USA), рН 7.4). They were stored at a temperature of 4 °С for the period not exceeding 3 hours.
Sample morphology and molecular composition of bone tissue, soft tissues and cells of the mandible were studied using Raman spectroscopy. The following settings of the confocal Raman/fluorescence microscopy NTEGRA-SPECTRA system (NT-MDT; Russia) were used: spectral range of 1000–3000 cm–1; step size of 0.8 cm–1; detection with CCD detector with Peltiercooling to –50 ° (objective 20× with an aperture of 0.15, grating 600 lines/mm); laser power at sample not exceeding 3 mW, laser excitation wavelength 532 nm, exposure time 10 s, 3 sample exposures. Number of repetitions for the experiment (sample): 12. Spectra processing involved background subtraction and smoothing of spectra using the Origin2017 software (OriginLab Corporation; USA).
The pilot study was carried out in order to define the possibility of using Raman spectroscopy to assess the condition of the tissues in the maxillofacial region; no statistical analysis was performed.
RESULTS
During the study the sample images and the Raman spectra of the components of autografts (bone tissue, soft tissues and cells), harvested from the retromolar region, were obtained.
Raman spectra of bone tissue of autografts harvested from the retromolar region
During this series of experiments the analysis of autograft bone tissue markers revealed characteristic Raman bands in the spectra of samples, corresponding to collagen, phosphate groups, apatite (PO43–), type B carbonate ion (such as СО3–2), as well as proteins adsorbed to the cell surface (bending N–Н vibrations and stretching С–N vibrations), lipids (С–Н groups of fatty acids of lipids), and their ОН groups.
Thus, in the patient’s Raman spectrum of the sample № 2, a number of bands was observed corresponding to phosphate groups PO43 (563, 943, 975 cm–1), collagen (1270 cm–1), type B carbonate ion СО3–2 (1025, 1051 cm–1), proteins and lipids (С–Н groups of fatty acids of lipids, 2862, 2890, 2946 cm–1), and their ОН groups (3192, 3420, 3559, 3635 cm–1) (fig. 1). A number of bands were observed corresponding to phosphate groups (426, 583, 938 cm–1), type B carbonate ion СО3–2 (1009, 1023, 1056 cm–1), proteins (bending N–Н vibrations and stretching С–N vibrations of protein, 1607 cm–1), lipids (С–Н groups of fatty acids of lipids, 2857, 2889, 2948 cm–1), and their ОН groups (3204, 3408, 3621 cm–1), together with a number of bands defining the inorganic composition of bone tissue: phosphate groups PO4–3 (415, 457, 570, 580, 892, 936 cm–1 (phosphate group of apatite)), type B carbonate ion CO3–2 (1007, 1022, 1050 cm–1), as well as ОН groups of lipids and proteins, 3209, 3399, 3625 cm–1). The bands were observed defining the inorganic composition of the tissue: phosphate groups PO43– (567, 582, 941 cm–1), type B carbonate ion CO3 –2 (1004, 1021, 1054 cm–1), and ОН groups of adsorbed lipids and proteins (2952, 3214, 3620 cm–1) (see fig. 1).
Raman spectra of the tissue of autografts harvested from the retromolar region
When developing markers for detection of the autograft components during the operation, it was found that in the Raman spectrum there were a number of bands corresponding to collagen, blood hemoglobin, proteins (amide II groups of proteins), lipids (С–Н groups of fatty acids of lipids), and their ОН groups. In the patient’s Raman spectrum of the sample No. 2 a number of bands were observed, which indicated the presence of collagen (1270 cm–1), hemoglobin (1109, 1151, 1228, 1289, 1353, 1382, 1545, 1570, 1615 cm–1), proteins (C–N bonds, 3157, 3204 cm–1), lipids (С–Н groups of fatty acids, 2697, 2877, 2911, 2964 cm–1; phosphate groups of phospholipids, 924, 961 см–1), and their ОН groups (3402, 3588 cm–1) (fig. 2).
In the patient’s Raman spectrum of the sample № 1 a number of characteristic Raman bands were observed, corresponding to vibrations of certain bonds in the protein molecules (for example, 1632 cm–1, amide II of proteins; 2848, 2876, 2918, 2930 cm–1), lipids (С–Н groups of fatty acids of lipids), and their ОН groups.
Raman spectra of the cells of autografts harvested from the retromolar region
In order to reveal the markers of distinct cells of the autografts, a number of characteristic bands was detected in the spectrum, corresponding to collagen, red blood cell hemoglobin, proteins (amide and C–N bonds), and cellular lipids (С–Н groups of fatty acids and phosphate groups of lipids), as well as their ОН groups. In the patient’s Raman spectrum of the sample № 1 a number of Raman bands were observed corresponding to proteins and lipids (С–Н groups of fatty acids of lipids, 2848, 2876, 2930 cm–1), and their ОН groups (3230, 3420, 3612 cm–1) (fig. 3, fig. 4, fig. 5), collagen (1274 cm–1), red blood cell hemoglobin (1303, 1335, 1363, 1407, 1531, 1550, 1593 cm–1), proteins and lipids (phosphate groups, 924, 961 cm–1), С–Н groups of fatty acids of lipids (2848, 2876, 2930 cm–1), and their ОН groups (3230, 3420, 3612 cm–1) [18–20]. In the patient’s Raman spectrum of the sample № 2 there were a number of characteristic Raman bands, corresponding to proteins (amide, 1621; 2841, 2887, 2950 cm–1) and lipids (phosphate groups, 888, 1432 cm–1), as well as their ОН groups (3212, 3402, 3626 cm–1). In the patient’s Raman spectrum of the sample No. 2, proteins and lipids were detected (С–Н groups of fatty acids of lipids, 2836, 2876, 2946 cm–1), as well as their ОН groups (3214, 3397, 3614 cm–1). In the patient’s Raman spectrum of the sample № 3 there were a number of characteristic Raman bands corresponding to collagen (1314 cm–1 and 1603 cm–1), proteins (C–N bonds, 2974, 3002, 3932 cm–1), lipids (С–Н groups of fatty acids of lipids, 2846, 2902, 2938 cm–1), and their ОН groups (3238, 3406, 3607 cm–1) (see fig. 3, fig. 4, fig. 5).
DISCUSSION
In the course of our study we looked for the possibility of Raman signal acquisition of the molecules of tissues and cells during surgical harvesting of tissue for augmentation of alveolar processes with bone tissue deficiency (elective bone grafting) [15–17]. It's obvious that continuous monitoring of the tissue condition during surgery allows the surgeon to promptly change the surgical procedure and to perform further diagnosis [18–20]. During our study we used the optical non-invasive technique for rapid and effective diagnosis of changes in composition and conformation of molecules in the patient’s mandibular cells and tissues. We conducted preliminary research and acquired characteristic signals of bone tissue and periosteum tissue. We assume that the use of fiber optic probes and Raman spectroscopy would make it possible to identify problems and defects occurring during the surgical procedure at the molecular level. It has been found that the bands, indicating the presence of phosphate groups (426, 583, 938 cm–1) and type B carbonate ion СО3–2 (1009, 1023, 1056 cm–1), can be considered a characteristic marker for diagnosis of bone tissue condition. This, acquisition of data on these markers makes it possible to evaluate the contribution of these components and the changes of those during the surgical procedure. It is obvious that acquisition of the Raman signal of the cell and tissue population allows to acquire the combined spectrum. In order to identify the specific signal of the periosteum tissue (not bone tissue), the Raman signals have been acquired, indicating the presence of collagen, hemoglobin, various proteins (C–N bonds), lipids (С–Н groups of fatty acids and phosphate groups of phospholipids), and their ОН groups. Thus, the proposed approach would make it possible to detect changes both in bone tissue and periosteum tissue of the patient’s mandible [21, 22].
The important thing about our study is the fact that we have managed to acquire the Raman signals of the distinct cells of autografts, corresponding to distinct molecules of collagen, hemoglobin, proteins and lipids, and their ОН groups.
It has been found that Raman scattering may be used for evaluation of the autograft bone condition during transplantation. The data obtained suggest that the spectra of various autograft samples differ. The proposed method of autograft condition evaluation would be used for identification of certain proteins, lipids, and other molecules, which constitute the bone tissue, as well as for quantification of these molecules. During osseointegration, a large number of biologically active molecules are being synthesized, which may indicate enhanced or decreased integration [3, 6].
Thus, Raman signals of specific substances have been acquired in various tissues and cells. Perhaps, the use of advanced equipment and light guides for aquision of signal in the oral cavity during surgery might help to localize the effect on the bone tissue and distinct molecules of the autograft tissue (for example, hemoglobin, collagen, proteins or lipids) or the stem cells of the graft [23].
CONCLUSION
Thus, in the course of the study it has been found that Raman spectroscopy is appropriate for rapid and efficient assessment of molecular composition, as well as qualitative and quantitative assessment of changes in autograft bone used for mandibular bone augmentation. Early detection of factors, impairing osseointegration, and contributing to resorption of bone tissue surrounding the implant, is a key issue of modern dentistry. Therefore, the research directed to address the issue is essential and relevant. The results obtained allow us to suggest the possibility of using Raman spectroscopy for this purpose. The use of Raman spectra acquisition in combination with detection using light guides would allow the dentist to monitor the process of reparative regeneration and osseointegration during dental implantation. Combination of optical diagnosis and rapid digital feature analysis can significantly improve the efficiency of surgical procedure and treatment.