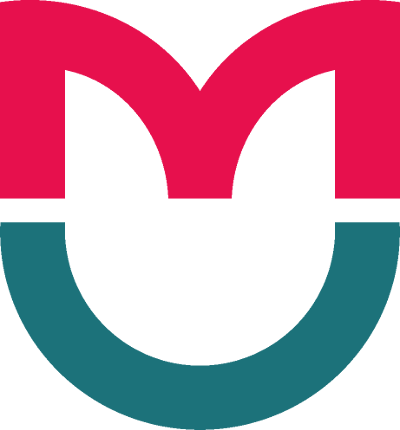
This article is an open access article distributed under the terms and conditions of the Creative Commons Attribution license (CC BY).
ORIGINAL RESEARCH
Retinal abnormalities in transgenic mice overexpressing aberrant human FUS[1-359] gene
1 Belgorod State National Research University, Belgorod, Russia
2 Institute of Physiologically Active Substances, Moscow, Russia
3 Kursk State Medical University, Kursk, Russia
4 Sechenov First Moscow State Medical University, Moscow, Russia
Correspondence should be addressed: Vladislav O. Soldatov
Pobedy, 85, 308015, Belgorod; moc.liamg@votadlosmrahp
Funding: the study was carried out with the financial support of the Russian Foundation for Basic Research within the framework of the scientific project № 19-315-90114.
Author contribution: Soldatov VO — the main idea, design of the experiment, ophthalmoscopy, writing an article, design of primers for gene expression assay; Kukharsky MS — the main idea, design of the experiment, writing an article, western blot analysis; Soldatova MO — RNA isolation, qPCR; Puchenkova OA — retina and spinal cord collection, RNA extraction, qPCR; Nikitina YuA — preparation of animal populations, genotyping, western blot analysis; Lysikova EA — preparation of animal populations, genotyping, western-blot analysis, writing an article; Kartashkina NL — interpretation and scoring of ophthalmoscopic picture; Deykin AV — consultation on the main idea and design of the study; Pokrovskiy MV — consultation on the main idea and design of the study.
Compliance with ethical standards: animal procedures were approved by the local ethics committee of the Belgorod State National Research University (protocol № 5 / 19–25 dated september 25. 2019). All manipulations were carried out in compliance with the requirements of the International Recommendations of European Convention for the Protection of Vertebrate Animals used for Experimental and Other Scientific Purposes (1997).
The retina is the most accessible for examination part of the nervous system and it is one of the most vulnerable sensory tissues. Such properties make the study of neurodegeneration ophthalmic correlates highly relevant, opening up opportunities for improving the diagnostics and study of neurodegenerative processes. Due to embryonic commonality and similarity of proteomic composition, the retina can serve as a platform for the development of pathological cascades the central nervous system is prone to [1]. In particular, retinal damage was found in amyotrophic lateral sclerosis (ALS) [2, 3], a disease caused by accumulation of insoluble protein aggregates leading to progressive death of motor neurons [4].
Protein inclusions in ALS have a complex composition and can consist of various proteins, such as RNA-binding proteins or the antioxidant enzyme superoxide dismutase 1, as well as neurofilaments or ubiquitin [5]. In 5% of familial cases, ALS is associated with the accumulation of aggregates, the main component of which is the FUS protein. One of the cause for the development of FUS proteinopathy is mutations in the nuclear localization signal (NLS) domain and the leak of the protein from the nucleus into the cytoplasm, where it is prone to form insoluble aggregates [6].
With all this background the aim of this study was to assess the relationship between neuronal expression of the pathological form of the FUS protein and the activation of several pathological pathways in the retina.
METHODS
Animals
Transgenic mice carrying the sequence of the aberrant human FUS gene encoding a protein with artificially truncated NLS (FUS[1-359]) [7] were used as a model recapitulating FUS proteinopathy. The FUS[1–359] murine model is characterized by progressive paralysis with onset at the age of 3–4 months accompanied by the development of morphological and molecular signs of neurodegeneration, including neuronal death and neuroinflammation [8].
The study was carried out on 25 CD-1 mice (both sexes), 12 of which were FUS hemizygotes, and 13 served as wild-type controls. The mice were kept in conditions of constant access to water and food. Light cycle — 12 h/12 h, illumination — 40–50 lx, temperature — 23 ± 1 °С, humidity — 42 ± 5%. At the age of 80–90 days, mice were sedated (Zolazepam + Tiletamine + Xylazine) for ophthalmoscopy. A brief clinical examination performed before sedation allowed to exclude animals with signs of inflammatory changes in the anterior chamber of the eyeball. Ophthalmoscopic examination was performed after application of 1% atropine sulfate. To perform quantitative objective analysis, the ophthalmologist assessed the fundus picture on a point scale from 0 to 5, where 0 points — no violations, and 5 points — severe abnormalities. Ophthalmoscopy scoring results presented as M ± SD; statistical significance for intergroup differences was challenged using the Kruskell–Wallis test. After ophthalmoscopic examination, the animals were euthanized with an overdose of anesthesia for further collection of biomaterial: totally 24 retinal samples were taken from 6 animals from each group for gene expression assay, and 8 retinas and 4 lumbar spinal cord samples were collected from 4 animals for western blot analysis.
Quantitative PCR
Contralateral retinas from each animal were pulled and incubated for 15 min in ExtractRNA solution (Evrogen; Russia).
After lysis of the sample in the reagent, it was subjected to chloroform extraction. The formed RNA precipitate was washed sequentially with isopropyl alcohol and 70% ethanol. The resulting precipitate was diluted in 20 μL of water, and the concentration of the isolated RNA (~ 200 ng/μL) was measured using an IMPLENNanoPhotometer® spectrophotometer (Implen; Germany). Reverse transcription was performed using the MMLVRTSK021 kit in accordance with the manufacturer's protocol (Evrogen; Russia).
Primers for quantitative PCR were designed with use of Primer-BLAST resource (NCBI) in compliance with the following requirements: 1) melting temperature 59–61°C; 2) one of the primers in a pair should span exon-exon junction; 3) forward and reverse primers should not form auto- and cross dimers; 4) the size of the PCR product should be from 95 to 200 bp; the primers must be specific to the maximum number of gene transcripts.
Quantitative PCR was performed in a BioRad CFX96 amplifier using the SYBR® Green Master Mix intercalating dye (Bio-Rad Laboratories, Inc .; USA) and oligonucleotide primers (Evrogen; Russia) (table). The expression level of genes of interest (GOI) was assessed relative to the housekeeping genes (HKG) Gapdh and Actb. Expression at a specific point was calculated using the formula: Gene expression = 2 ^ [(Ct (HKG) -Ct (GOI)].
Western blot
Retinal samples were pulled from two animals belonging to the same group. After separation in the gel, the proteins were transferred by semi-dry electroblotting onto a Hybond-P polyvinylidene fluoride membrane (Cytiva; UK) pretreated with 100% methanol, washed with MilliQ water, and soaked in transfer buffer containing 25 mM Tris, 0.15 mM glycine and 20% methanol. Then the membrane with gel placed between tightly pressed two sheets of soaked 3MM Wathman paper and transferred into in a semi-dry blotter (GE Healthcare Amersham; USA) allowing proteins to diffuse to the membrane for 30 min at 50 mA (1.2 mA by 1 cm2). After electroblotting, the membrane was washed in Tris-Tween buffer (TTB; 50 mM Tris-HCl pH 7.4, 150 mM NaCl, 0.1% Tween-20) 3 times for 5 min. The membrane was blocked in a 4% solution of nonfat dry milk in TTB for 1 h at room temperature, then incubated with primary antibodies in the same solution at 4 °C overnight. After incubation with primary antibodies, the membrane was washed in TTB 3 times for 5 min and incubated with secondary antibodies for 1.5 h at room temperature, and then rewashed in TTB 3 times for 5 min. Detection of specific binding of antibodies was performed using ECL Plus reagents (Cytiva; UK) according to the manufacturer's instructions. X-ray film was used to detect chemiluminescence. Semi-quantitative assay of the westerns was performed using densitometric analysis using a BioSpectrum AC Chemi HR410 instrument and Vision Works LS software (UVP; Great Britain). When carrying out densitometric analysis, the specific signal from the analyzed protein was normalized in relation to the signal from β-actin (after the membrane reincubation with the corresponding antibodies) for each lane separately.
RESULTS
FUS[1-359] transgenic mice show mild ophthalmoscopic abnormalities.
In both groups, some of the animals were found to have vascular anomalies and edema of the optic disc, which is routine finding for CD-1 mice [9, 10]. Statistical analysis did not reveal significant differences between transgenic and wildtype animals; however, FUS[1-359] mice showed a tendency towards more pronounced violations in all studied parameters (fig. 1).
Truncated FUS is not expressed in the retina
Western blot analysis did not reveal the presence of a FUSimmunopositive signal in the retinal tissues of transgenic mice (fig. 2). Furthermore, quantitative PCR also did not detect FUS at the mRNA level. Thus, expression assay did not confirm retinal expression of the transgene at either the transcriptome or protein levels.
Transgenic FUS[1-359] mice are characterized by increased expression of proinflammatory genes in the retina
Gene expression assay found increased levels of proinflammatory factors Vegfa, Icam1, Il6, Il1b, and Tnfa in the retina of transgenic FUS[1-359] mice. No pronounced changes in the expression of genes related to neuroplasticity (Bdnf), autophagy (Atg7, Atg5), and regulation of apoptosis (Bax, Bcl2) were found (fig. 3).
DISCUSSION
Retinal involvement has been found in various neurodegenerative diseases, including Alzheimer's disease [11], Parkinson's disease [12, 13], and frontotemporal dementia [14]. Not surprisingly, mild ophthalmic abnormalities are also a frequent non-motor symptom of ALS [15]. Among typical clinical findings, color vision impairment [16], as well as thinning of the retina [17] and macula [18–20], are described.
In our study, we did not find significant differences in the severity of ophthalmoscopic changes in the studied animals. Nevertheless, for all studied parameters, an obvious tendency towards more pronounced disorders was revealed in transgenic FUS[1-359] mice. Particularly bright differences were found in relation to the retinal vasculature, which is consistent with the results of the previous report [21].
Since transgene is not expressed in the retina, we aimed to elucidate the mechanisms of retinal degeneration by studying the activity of the most common molecular pathways of neurodegenerative damage to motor neurons. In this regard, as the primary targets we focused on genes related to inflammation, apoptosis, and autophagy. Although pathways of autophagy [22–24] and apoptosis [25] are deeply involved in ALS as confirmed by various in vivo and in vitro studies, we did not find changed expression of genes Atg7, Atg5, Bax, Bcl2. However, the expression of the Vegfa, Icam1, Il6, Il1b, and Tnfa genes was altered markedly, indicating the role of inflammation in the mechanisms of retinal degeneration in FUS[1-359] mice. These findings are consistent with previous results regarding the dramatic role of inflammatory activation (and its pharmacological suppression) in the FUS[1-359] mouse model [26, 27].
Thus, despite truncated FUS protein is not expressed in retinal tissue, transgenic animals show signs of retinal abnormalities. Potential mechanisms of damage to the eye fundus may include activation of microglia [28], vascular regression [20], and neuro-phthalmic interactions through the glymphatic system [29].
CONCLUSIONS
The study has demonstrated that transgenic FUS[1-359] mice are prone to the development of structural and functional abnormalities of the eye fundus, despite the absence of transgene expression in the retina. Further detailing of identified violations can highlight the most significant pathways of FUS-associated retinopathy.