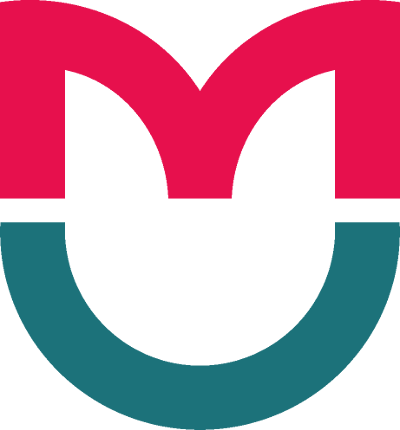
ORIGINAL RESEARCH
The nature of genotypic resistance to fluoroquinolones in Mycobacterium tuberculosis circulating in Russian Federation
Central Tuberculosis Research Institute, Moscow, Russia
Correspondence should be addressed: Sofia N. Andreevskaya
Yauzskaya Alleya, 2, str. 1А, Moscow, 107564, Russia; ur.liam@aifosdna
Funding: the study was conducted as part of the State Assignment № 122041100246-3 for the Central Tuberculosis Research Institute, “Intra- and Inter- species Polymorphism of Mycobacteria in Patients with Tuberculosis and Mycobacteriosis Who Receive Specific Therapy”.
Author contribution: Ergeshov A, Chernousova LN — study design; Larionova EE, Kiseleva EA — data acquisition; Smirnova TG — data analysis; Andreevskaya SN — manuscript writing, literature review; all authors contributed to the discussion.
The spread of tuberculosis caused by drug resistant pathogen is a major public health concern. Particularly worrisome is the wide spread of multidrug-resistant tuberculosis (MDR-TB), i.e. resistant to both of two most effective antituberculosis drugs, rifampicin and isoniazid. According to the WHO, the effectiveness of MDR-TB therapy is only 59% [1]. Russia is among the countries with a high burden of MDR-TB. Despite the fact that the prevalence of MDR-TB in the country has started to decline in recent years (from 20.6 per 100,000 population in 2020 to 18.1 per 100,000 population in 2021), the rate is still high [1, 2].
Regimens for treatment of MDR-TB necessarily include fluoroquinolones (group A drugs according to the WHO classification reflecting priorities of the drug inclusion in the treatment regimens) [3]. DNA gyrase, the enzyme essential for replication and transcription in the M. tuberculosis (MTB) cell, is a target of fluoroquinolones [4, 5]. In 60–90% of cases, fluoroquinolone resistance is associated with mutations in the quinolone resistance-determining region (QRDR) of the gyrA gene encoding the DNA gyrase α-subunit [6, 7]. The TBDreamDB database (http://www.tbdreamdb.com) contains information about 17 variants of QRDR mutations associated with fluoroquinolone resistance, among which 10 variants show high reliability in terms of resistance to this group of medications [8].
The development of additional resistance to fluoroquinolones by the MDR MTB results in pre-extensively drug resistant tuberculosis (pre-XDR TB), the treatment of which requires expensive long-term chemotherapy. The WHO estimates that up to 20% of tuberculosis cases in 105 countries fall in this category. To improve the effectiveness of pre-XDR TB treatment it is necessary to adjust the course of chemotherapy based on the fluoroquinolone susceptibility defined by molecular genetic methods as early as possible. However, according to the WHO, the global coverage of the fluoroquinolone susceptibility testing is still low: it accounts for 50% of the identified worldwide cases of tuberculosis [1]. Two domestic test systems for rapid detection of MTB susceptibility to fluoroquinolones are used in Russia. The first one, TB-BIOCHIP-2 (Biochip-IMB; Russia), includes biochips that detect 10 variants of the QRDR spot mutation. The second one, Amplitube-FQ-RV (Syntol; Russia) based on the allele-specific PCR, detects six mutations in the gyrA QRDR.
The study was aimed to assess the diversity of mutation in the gyrA gene and to evaluate the association of gyrA mutations with phenotypic resistance to levofloxacin and the general drug resistance profile of the pathogen.
METHODS
Research object
Diagnostic materials obtained from patients of all age groups admitted to the diagnostic and clinical departments of the Central Tuberculosis Research Institute in 2011–2019 were assessed.
Study design
Retrospective analysis of the data on mutations in the gyrA QRDR and MTB phenotypic drug resistance obtained within 9 years (2011–2019) from patients with pulmonary tuberculosis, who were treated in the Central Tuberculosis Research Institute, was conducted. Diagnostic materials were assessed in accordance with the standard algorithm accepted by Microbiology Department of the Central Tuberculosis Research Institute: each sample of diagnostic material was simultaneously assessed by the culture-based and molecular genetic methods. Diagnostic materials were decontaminated using the standard procedure, then the MGIT tubes were inoculated for further growth in the BACTEC MGIT 960 system [9]. DNA was isolated from the amount of diagnostic material remaining after inoculation, and PCR was conducted to detect the MTB DNA. Upon receipt of a positive PCR result, mutations in the genes associated with resistance to rifampicin, isoniazid, and fluoroquinolones were identified using biochips or allele-specific PCR. The MTB culture obtained was tested for susceptibility to eight antituberculosis drugs.
DNA extraction
DNA was extracted from diagnostic materials using the Amplitube-RV reagent kit for isolation, detection and quantification of the Mycobacterium tuberculosis complex DNA by real-time PCR, kit № 1 (Syntol; Russia) according to the instructions.
Identification of MTB DNA
To detect the MTB DNA, PCR was conducted using the Amplitube-RV reagent kit for isolation, detection and quantification of the Mycobacterium tuberculosis complex DNA by real-time PCR, kit № 2 (Syntol; Russia) according to the instructions. Amplification was performed in the CFX96 thermal cycler with optical module (Bio-Rad; USA).
Genotypic resistance to rifampicin and isoniazid
The test was performed either by the microchip technique using the TB-BIOCHIP-2 kit (Biochip-IMB; Russia), or using the Amplitube-MDR-RV kit (Syntol; Russia). Both procedures were conducted in accordance with the manufacturers' instructions.
Genotypic resistance to fluoroquinolones
The samples obtained in 2011–2015 were tested by the microchip technique using the TB-BIOCHIP-2 kit (Biochip-IMB; Russia), while the samples obtained in 2015–2019 were tested using the Amplitube-FQ-RV kit (Syntol; Russia). Both procedures were conducted in accordance with the manufacturers' instructions. In case the tests conducted during various stages of therapy were available for one patient or different diagnostic materials (for example, sputum and excision material) obtained from one patient were tested, the results of testing each of the samples for gyrA mutations were compared.
Culture-based diagnosis
MTB was detected in the Middlebrook 7H9 liquid growth medium with the BACTEC MGIT 960 system (BD; USA) in accordance with the standard protocol developed by the manufacturer [9].
Phenotypic drug resistance
The test for susceptibility to eight antituberculosis drugs (rifampicin, isoniazid, ethambutol, pyrazinamide, ethionamide, amikacin, capreomycin, and levofloxacin) was performed by the modified proportion testing method in the BACTEC MGIT 960 system (BD; USA) in accordance with the manufacturer's guidelines [9, 10].
Methods of statistical analysis
Descriptive statistics was used to assess the results: number of observations, frequency, share (percentage), and 95% confidence interval (95% CI) were taken into account. Chi-squared test (χ2) was used for intergroup comparison. The differences were considered significant at p < 0.05. Analysis was performed using Microsoft Excel (Microsoft; USA).
RESULTS
In 2011–2019, microbiological diagnosis of tuberculosis was performed in 4451 patients. The study involved materials obtained from 2836 patients with positive MTB DNA PCR test results. Of them in 2082 cases (73.41%, 95% CI: 71.76–75.01%) no gyrA mutations were detected by molecular genetic methods (hereinafter, the wild type gyrA).
MTB with single gyrA mutations were isolated from 699 patients (24.65%, 95% CI: 23.10–26.27%). In MTB isolated from 55 patients (2.31%, 95% CI: 1.78–3.00%), the results of determining genotypic resistance to fluoroquinolones changed over time or according to the diagnostic material type. These cases designated as heteroresistance will be described in detail below.
Frequency of single mutations in the gyrA QRDR
Single mutations detected in the gyrA QRDR were located in codons 88, 90, 91 or 94 (tab. 1). Mutations were most often found in codon 94 of the gene (496/699, 70.96%; 95% CI: 67.49–74.20%). These were represented by five single-nucleotide variants, among which a D94G substitution was the most common (291/496, 58.67%; 95% CI: 54.29–62.92% among mutations in codon 94 and 291/699, 41.63%; 95% CI: 38.03–45.32% among MTB with single gyrA mutations). The A90V substitution was the second most frequent mutation (149/699, 21.32%; 95% CI: 18.44–24.50%). In total, MTB carrying mutations D94G and A90V accounted for more than half of all cases of MTB carrying single gyrA mutations (440/699, 62.95%; 95% CI: 59.31–66.45%).
Phenotypic susceptibility to levofloxacin
Phenotypic susceptibility to levofloxacin was defined for MTB isolated from diagnostic materials obtained from 1326 patients by the culture-based method. Testing of these MTB isolates for gyrA mutations revealed MTB with the wild type gyrA in 846 cases and MTB carrying single gyrA mutations in 480 cases. MTB with the wild type gyrA were largely susceptible to levofloxacin (814/846, 96.22%, 95% CI: 94.71–97.31%), while MTB carrying mutations usually were levofloxacin-resistant (448/480, 93.33%, 95% CI: 90.74–95.24%) (tab. 2).
Polymorphism of MTB variants with mutant gyrA isolated in the new cases and previously treated patients with tuberculosis
Among 2836 patients with pulmonary tuberculosis, whose diagnostic materials were included in the study, there were 1253 new cases and 767 previously treated ones. No information about the status of another 816 patients was available.
MTB with the wild type gyrA were most often isolated in the new cases than in the previously treated ones: among 1475 isolates of MTB with the wild type gyrA obtained from patients with known status, 1012 (68.61%) were obtained in the new cases and 463 (31.39%) were isolated in the previously treated ones (p-value ≤ 0.001). The gyrA mutant variant A90V was significantly more common in the group of MTB isolated from the new cases; no significant differences were revealed for other mutant variants (tab. 3).
gyrA mutations in MTB showing resistance of different types
MTB isolates with known genotypic susceptibility to fluoroquinolones were divided into five categories based on the resistance type (cases of heteroresistance were not included in the analysis): MDR MTB fell into the first category, polyresistant MTB (resistant to all combinations of antituberculosis drugs except the combination of rifampicin and isoniazid) fell into the second, fluoroquinolone-monoresistant MTB fell into the third, MTB monoresistant to other antituberculosis drugs fell into the fourth, and MTB susceptible to all antituberculosis drugs fell into the fifth one (tab. 4). MTB carrying gyrA mutations usually showed MDR or polyresistance (in total, 689/694, 99.28%, 95% CI: 98.32–99.69%). Fluoroquinolone-monoresistance was extremely rare (5/694, 0.72%, 95% CI: 0.31–1.68); in four cases, such MTB carried gyrA mutation D94A, and in one case mutation D94G was found. MTB with the wild type gyrA were almost equally divided between the categories of MDR MTB and MTB susceptible to all antituberculosis drugs.
Heteroresistance and multiple mutations
The listed above MTB isolates showed the same structure of the gyrA QRDR (wild type or single mutation) in all samples obtained from the same patient over time. However, the data on the gyrA QRDR structure obtained by dynamic monitoring of 55 patients differed (tab. 5).
Thus, when assessing diagnostic materials during chemotherapy, MTB with varying structure of the gyrA QRDR were isolated from 35 patients. Of them in 22 cases both MTB with the wild type gyrA and MTB carrying mutations were revealed in the samples obtained from one patient. The wild type gyrA and gyrA with single mutations (mostly D94G) were found in MTB isolated from 15 patients (tab. 5, item 1.1.1). MTB with the wild type gyrA and gyrA carrying multiple mutations were isolated from seven patients (tab. 5, item 1.1.2). In three out of these seven cases, co-existence of two pools of MTB carrying single mutations instead of the existence of one pool with the double gyrA mutation was proven, since the samples with single mutations were also isolated over time. In four out of seven cases, the existence of two pools with single mutations or one pool with the double gyrA mutation was not proven.
On different dates samples were obtained from 13 patients, from which MTB carrying various single mutations were isolated (eight out of 13), or among which samples carrying double or single mutations alternated (five out of 13). This could indicate that there were several MTB pools with mutant gyrA in the patient's body (tab. 5, item 1.2).
In five cases, heteroresistance was revealed when checking exceptions of the tests for phenotypic and genotypic resistance: MTB DNA carrying gyrA mutations was obtained from the diagnostic sample, while MTB culture obtained from the sample showed phenotypic susceptibility to levofloxacin, or vice versa. In such a case the available DNA samples were repeatedly (up to eight times) tested for gyrA mutations, and fresh DNA was isolated from the diagnostic sample and tested for gyrA mutations. The data both consistent with the initial results and different from these results were obtained in each of these five cases in a series of tests for gyrA mutations. This could somehow prove the presence of the MTB mixed population in one diagnostic sample (tab. 5, item 2).
In another 15 cases, we detected double mutations in one sample (only one sample per patient was available for testing) (tab. 5, item 3). It was usually one of the most common mutations (D94G or A90V) combined with one rare mutation (nine cases out of 15). However, in five cases out of 15, two rare mutations were simultaneously detected: it was the S91P + D94A combination only. MTB carrying two most common mutations (D94G and A90V) were isolated from only one patient. In all 15 cases, another PCR test of DNA isolated from the diagnostic sample revealed two mutations again. This indicated that either one MTB pool with the genomes containing double gyrA mutations, or two MTB pools carrying single gyrA mutations and represented in equal proportions were isolated from one patient. In these cases, diagnostic materials were collected from patients only once, that is why no ongoing monitoring allowing us to clarify the data obtained was performed.
Thus, we have shown that the patient could be infected with 2–4 MTB pools showing different structure of the gyrA QRDR. Mixed populations were most often represented by two MTB pools (46/55, 83.64%, when 15 cases of double mutations with unproven membership in two different pools were included in the analysis). Of those in 19 cases the population consisted of the MTB pool with the wild type gyrA and MTB pool carrying single gyrA mutations. In all other cases, (27, when 15 cases of double mutations for which no data of the ongoing monitoring were available were included in the analysis) MTB population was represented by two MTB pools with various gyrA mutations
In seven cases, MTB population found in one patient was represented with three pools showing different gyrA structure. In six cases, one MTB pool contained the wild type gyrA and two pools carried different gyrA mutations, while in one case all three MTB pools carried various gyrA mutations. Co-existence of three MTB pools showing different gyrA structure in one patient was proven by ongoing monitoring in five cases out of seven.
The presence of four MTB pools showing different gyrA QRDR structure could be suspected, since in one case susceptible MTB were isolated, and in another case tree gyrA mutations were identified in two samples of diagnostic material. It is hard to imagine that independent sequential processes of spontaneous mutagenesis could result in the emergence of three mutations at once within the same gene region, that is why it is reasonable to assume that there are three independent MTB pools carrying different mutations.
Mixed populations of MTB were usually isolated from the previously treated patients (40/55, 72.73%, 95% CI: 59.77–82.72). These populations were represented mostly by MDR MTB (45/55, 81.82%, 95% CI: 69.67–89.81). However, in two cases out of 55, mixed populations of MTB characterized by levofloxacin monoresistance were isolated from the new cases.
DISCUSSION
A retrospective study that covered a significant number of tuberculosis cases diagnosed in 2011–2019 was carried out to assess the diversity of mutation in the gyrA gene QRDR of MTB.
Of the eight identified nutant variants, seven were highly credible in terms of developing resistance to fluoroquinolones [8]. Mutations D94G and A90V were the most common, which was typical for the entire world's population [6, 7]. Our data on the frequency of these mutations (40.42% for D94G and 21.26% for A90V) showed that it was slightly higher compared to the global population (21–32% and 13–20%, respectively) [6].
The featured study shoes that mutations in the gyrА QRDR were most often associated with phenotypic resistance to fluoroquinolones, however, in rare cases, testing of MTB for gyrА mutations by the culture-based method revealed no fluoroquinolone resistance. This was explained by the MTB population heteroresistance after conducting additional studies. It is known that in case the share of one strain in the mixture is less than 5% when assessing phenotypic and genotypic resistance to fluoroquinolones, it is impossible to define its genotype and phenotype, and the results reflect the characteristics of the strain that dominates in the mixture [11]. This is important to consider when interpreting the mismatching results of testing for fluoroquinolone resistance by the culturebased and molecular genetic methods, since the initial low concentration of MTB with certain genotype in the cell mix and the likelihood of uneven distribution of MTB cells with various genotypes among samples collected for further molecular genetic and culture-based tests cannot be excluded.
When D94A mutation was detected in gyrA in 24 cases out of 73 (32.88%), MTB showed phenotypic resistance to levofloxacin. It is hard to explain such a high percentage by the undetected heteroresistance or errors in the culture-based or genotypic testing. Furthermore, other papers also report cases of the gyrA_D94A genotype corresponding to the susceptible phenotype: phenotypic resistance to fluoroquinolones was detected in one out of seven and four out of 12 MTB strains carrying this mutation, depending on the studied population [12, 13]. Therefore, it can be concluded that regardless of the fact that mutation D94A is highly credible in terms of developing resistance, mutation is not strongly associated with the fluoroquinolone resistance.
There is a theory that the widespread use of fluoroquinolones for treatment of nontuberculous infections may result in fluoroquinolone resistance developed by patients with undiagnosed tuberculosis [14]. In this regard it was important to evaluate the nature of resistance in the fluoroquinolone-resistant MTB: association of this parameter with multiple drug resistance of the pathogen can indicate that resistance to fluoroquinolones develops during treatment of MDT-TB, while identification of the cases of fluoroquinolone monoresistance, especially in the new cases, may be an indicator of fluoroquinolone resistance developed by MTB during treatment of other infectious diseases. We showed that the MTB genotypic resistance to fluoroquinolones was most often associated with MDR: 649 isolates carrying single gyrA mutations out of 694 (93.52%) and 45 cases of mixed populations out of 55 (81.00%) fell into the MDR category.
The findings prove that fluoroquinolone resistance is developed by MTB during treatment of MDR-TB. However, we cannot rule out the fluoroquinolone resistance developing during treatment of nontuberculous infections, since MTB showing fluoroquinolone monoresistance have been also found. Unfortunately, we do not know, whether these patients were previously treated with fluoroquinolones.
The study of the MTB genome structure, that involved MTB circulating in Samara region, performed by whole genome sequencing, allowed the authors to conclude that MTB were more likely to acquire fluoroquinolone resistance during therapy, and the cases of human infections caused by the fluoroquinolone-resistant MTB clones were rare. Based on this observation, it was assumed that the development of fluoroquinolone resistance resulted in the reduced MTB fitness [13]. The population-based study of the MTB primary fluoroquinolone resistance distribution across the Novosibirsk region also showed that resistance to fluoroquinolones most often resulted from the use of drugs of this group in chemotherapy of MDR-TB [15].
The findings presented here also confirm that the development of fluoroquinolone resistance during treatment is more frequent: MTB carrying gyrA mutations were more often isolated from the previously treated patients than from the newly diagnosed ones. However, we have found that fluoroquinolone-resistant MTB could be also isolated from the newly diagnosed patients with tuberculosis. In these cases gyrA mutations D94G and A90V were the most common, although the frequency of A90V mutation in MTB isolated from the newly diagnosed patients was significantly higher than in that isolated from the previously treated ones. Therefore, it can be concluded that MTB carrying this mutation are transmitted rather actively between humans.
The described possibility of the co-existence of several MTB population varying in gyrA mutations in one patient refers to the development of fluoroquinolone resistance in the population. A number of studies also showed that there could be several MTB clones with different structure of gyrA in one diagnostic sample; such samples accounted for 1–3% of the total number, which was consistent with our results [16–18].
Thus, we have shown that fluoroquinolone resistance is currently developing in the population of MTB circulating in the RF, which is usually associated with the pre-existing MDR. MTB resistance to fluoroquinolones has good prospects in terms of evolution, since it is developed in favorable genetic conditions during treatment of tuberculosis, caused by pathogen showing MDR, that results from the combination of mutations, that do not reduce MTB fitness [19]. The fact, that MTB carrying gyrA mutations D94G and A90V were also rather frequent in the new cases, allows us to conclude that it is these mutants that would play a key role in the spread of pre-XDR tuberculosis across the RF.
CONCLUSIONS
Retrospective analysis of the range of mutations in the QRDR of the gyrA gene of MTB isolated in 2011–2019 showed that genotypic resistance to fluoroquinolones was detected in 26.96% of the MTB clinical isolates, including cases of heteroresistance. Mutations D94G and A90V were the most common, their frequency totaled 62.95% of the number of MTB carrying a single gyrA mutation. It was also shown that these two mutations were rather common in MTB isolated in the new cases (D94G was found in 46.03%, and A90V was found in 25.10% MTB in this group), thus confirming successful spread of these MTB mutant variants in the modern population. The presence of gyrA mutations was usually associated with phenotypic resistance to levofloxacin, except for mutation D94A that was associated with phenotypic resistance to levofloxacin in only 67% of cases. The gyrA mutations were found mostly in MDR MTB: 93.52% of the strains carrying gyrA mutation were also resistant to rifampicin and isoniazid. The findings proved that resistance to fluoroquinolones was developed during treatment of MDR-TB. Furthermore, in 2.31% of cases heteroresistant MTB populations were found that included 2–4 MTB pools with different structure of the gyrA QRDR. Fluoroquinolone heteroresistance indicates active development of resistance to this group of medications in the current conditions.