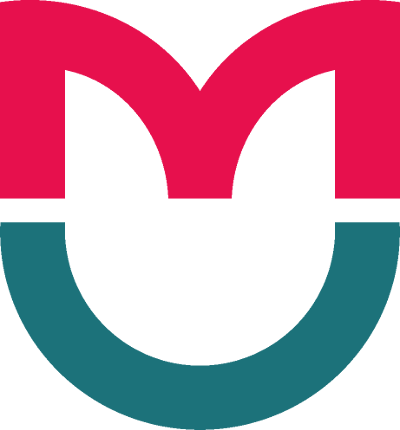
This article is an open access article distributed under the terms and conditions of the Creative Commons Attribution license (CC BY).
ORIGINAL RESEARCH
Clinical significance of cytokine counting in patients with multiple sclerosis and its relationship with herpes infection
1 Yaroslavl State Medical University, Yaroslavl, Russia
2 Pirogov Russian National Research Medical University, Moscow, Russia
3 Nasonova Research Institute of Rheumatology, Moscow, Russia
4 Sechenov First Moscow State Medical University (Sechenov University), Moscow, Russia
Correspondence should be addressed: Natalia S. Baranova
Revolutsionnaya, 5, Yaroslavl, 150000, Russia; ur.liam@sn_avonarab
Funding: the work was supported by the Innovations Assistance Fund within the framework of the UMNIK program: Participant of the youth scientific and innovative competition (contracts № 3560GU1/2014 of 23.09.2014, № 8815GU2/2015 of 17.12.2015).
Author contribution: NS Baranova, MC Gris — study planning and design, data analysis, manuscript authoring; MC Gris, AS Artyukhov, KM Shakirova — data collection and research; AA Baranov — data analysis; all autors — manuscript editing.
Compliance with ethical standards: the study was approved by the Ethics Committee of the Yaroslavl State Medical University (Minutes № 1 of October 1, 2013). All patients signed a voluntary informed consent.
Multiple sclerosis (MS) is a chronic disease of the central nervous system with autoimmune inflammatory and neurodegenerative mechanisms of development [1]. The processes important for immunopathogenesis of the disease are penetration of activated T cells (type 1 T helper cells (Th1), Th17 cells) and macrophages through the blood-brain barrier into the brain tissue, local activation of astrocytes and microglia, production of anti-inflammatory cytokines by them [2, 3] and subsequent demyelination and neuron degeneration. The etiology of MS remains unknown. It is believed that, in addition to genetic factors, various persistent infections contribute to its development [4]. Herpes simplex virus type 1 and 2 (HSV-1 and HSV-2) [5], varicella zoster virus (VZV) [6], human herpesvirus 6 (HHV6) [7] and Epstein–Barr virus (EBV) play a major role in the occurrence of MS, development of exacerbations and progression of the pathological process [8–11].
Production of cytokines as part of the innate immune response is crucial for arrest of spread of herpes infection in a body [11, 12]. In MS cases, lack of balance between production of pro- and anti-inflammatory cytokines is also considered the key factor in the development of the disease's exacerbations and progression of the inflammatory immune response. Interleukins (IL) IL1-β, IL2, IL4, IL6, IL10, IL17, IL23, tumor necrosis factor — (TNF-α) and interferon-γ (INF-γ) are the best studied response agents [2, 3, 13–15]. The role played by other cytokines, IL31 and IL33 in particular, in the pathogenesis of MS has not been investigated sufficiently; the data available originate from foreign sources exclusively [16–21]. Moreover, neither in Russia nor abroad have researchers sought to determine cytokines in blood serum during reactivation of herpes infection in MS patients, and subsequently assess their clinical significance. This gap in data presented in the published works substantiated this study, which aimed at determining the level of cytokines in the blood serum of MS patients, assessing their clinical significance and how they affect reactivation of herpes infection.
METHODS
The work was conducted at the premises of the Research and Educational Center for Demyelinating Diseases of the Yaroslavl State Medical University and Center for Multiple Sclerosis of Yaroslavl Clinical Hospital № 2 (Russia).
The study included 36 patients, 12 male and 24 female, with MS confirmed under McDonald criteria [22] (tab. 1). The mean age of the patients at the time of the study was 38.5 (28.0; 48.5) years, the age of onset of the disease — 27.00 (21.5; 38.0) years, and duration of the disease — 9.50 (3.5; 12.5) years. Multiple sclerosis was relapsing-remitting (RRMS) in all patients, with 29 (80.6%) of them in remission and 7 (19.4%) suffering exacerbation of the disease.
Thirty (83.3%) patients were undergoing a course of MS disease modifying drugs (DMDs), with 14 taking glatiramer acetate (GA), 16 — high-dose interferons (INF); the mean duration of the course was 30.0 (9.0; 67.0) months. Six (16.7%) patients were out of DMDs therapy at the time of examination.
For clinical assessment of the patients' neurological status, we relied on the J.F Kurtzke's assessment system [23] that includes the Functional Status Scale (FSS) and the Expanded Disability Status Scale (EDSS). The average score on the EDSS scale was 3.25 (2.00; 4.50), and the sum of neurological impairment — 6.50 (3.00; 9.00) points. Using the F. Lublin's classification [24], we divided the RRMS patients into active MS (n = 17, 47.2%) and inactive MS (n = 19, 52.8%) groups. In the active MS group, we also marked patients with highly active MS (n = 6, 35.3%), i.e., two or more exacerbations a year.
The key indicators of the course of the disease taken into account were first symptoms, duration of the first remission, patient's age at the time of onset, total neurological impairment score on Kurtzke's scale (TNIS), number and severity of exacerbations. We distinguished between mild MS exacerbations (TNIS growing by 0.5–1 point on EDSS scale), moderate exacerbations (growth by 1–2 points) and severe exacerbations (growth by more than 2 points) [25]. The mean annual frequency of exacerbations was taken as a ratio of the number of exacerbations to the duration of the acute period in years. The rate of disease progression was taken as a ratio of the level of disability (as per EDSS, in points) to the duration of the disease in years (point/year).
The progression index, which reflects the rate of neurological decline (RND), was calculated as the ratio of FSS score (TNIS) to the duration of the disease in years. Prognosis of the MS course was based on the calculated period of time before the patient became persistently disabled (3 EDSS points), duration of the first remission, and time before onset of secondary progression.
In the anamnesis, we paid special attention to herpesvirus diseases. In order to refine the MS development and aggravation risk factors, we designed a special questionnaire with which the patients reported if they suffered manifestations of labial and genital herpes often, had virus-associated exacerbations of MS, lived with chronic stress, subfebrility, chronic foci of infection, frequently contracted respiratory viral diseases and saw links between them and exacerbations of MS. We also examined patients for herpes rashes.
Based on the data obtained, the patients were divided into two groups. The first group included 18 patients (50%) with confirmed MS and reactivation of peripheral herpes virus infection (PHVI). The inclusion criteria for this group were confirmed MS combined with signs of PHVI, i.e., presence of both clinical (typical vesicular rashes, subfebrility, lymphadenopathy, arthralgia, myalgia, etc.) and serological signs of an active herpes virus infection; presence of only clinical signs of HSV at the time of exacerbation or within two weeks before and after it. Serological sign: low type-specific IgG antibodies avidity index (less than 50%) and IgG positivity coefficient (PC) three or more times greater than the reference range or specific IgM antibodies in the blood. The second group comprised 18 patients (50%) with confirmed MS with no signs of reactivation of PHVI registered by clinical and serological studies and/or recorded in the medical history. Table 2 (tab. 2) presents clinical characteristics of these groups.
In cases of PHVI, the onset occurred at an earlier age (p < 0.05, significant difference), the disease affected mainly women and had a shorter duration. The flu-like response to MS DMDs was more apparent, and exacerbations happened virtually twice as often (27.8%) as in the control group (11.1%). No differences were noted in other considered characteristics of patients between the compared groups, including age at the time of examination, disease severity, level of disability, MS DMDs therapy status. In both groups, patients had mixed HSV infection in most cases, but only those with PHVI exhibited serological markers of two infections (HSV-1, HSV2/VZV and EBV).
The control group included 18 practically healthy donors without clinical, historical and serological signs of PHVI. These participants had no chronic neurological diseases and somatic pathology in the acute stage. Seeking to detect diseases that could affect results of the study, we examined everyone (standard neurological examination) and collected medical histories thoroughly. This group was comparable to the MS group in terms of gender and age: 7 (38.9%) male and 11 (61.1%) female participants, mean age of 39.10 (29.00; 49.60).
Using the EIA method and standard kits (Vector-Best; Russia), we examined blood serum of all participants (both MS group and control group) with the aim to establish the levels of type-specific IgM and IgG antibodies to HSV-1 and HSV2, IgM and IgG to VZV, IgM and IgG to capsid antigen VCA EBV, IgG to early EA antigens and nuclear antigen NA EBV, IgM and IgG to CMV. The examination followed manufacturer's instructions and was carried out in the clinical and diagnostic laboratory of Set LLC (Yaroslavl). The PI taken as reference for the determined level of antibodies to HSV-1 and HSV-2 was >1 u/ml, that for the level of IgG to CMV — 0.25 RU/ml. The result was considered positive if the level of immunoglobulins G (IgG) exceeded the PI by 3 or more times, the IgG avidity index was low (less than 50%), or the blood contained immunoglobulins M (IgM) and we clinically registered activation of a latent herpes infection.
The concentration of 15 cytokines in blood serum (IL1ß, IL4, IL6, IL10, IL17A, IL17F, IL21, IL22, IL23, IL25, IL31, IL33, INF-γ, TNF-α, sCD40L) was determined with the help of a Bio-PlexTM 200 System analyzer (Bio-Rad; USA) utilizing xMAP multiplexing technology. The tests were carried out with manufacturer's reagents in the laboratory of the Translational Medicine Research Institute of Pirogov Russian National Research Medical University. We analyzed both the mean level of each cytokine and the frequency of its increase (peaks beyond the upper limit of normal M+3 σ in the control group).
For statistical processing, we used Statistica 10.0 software package (StatSoft; USA) and generally accepted methods of parametric and nonparametric analysis. Mann–Whitney test enabled comparison of two groups for parameters with abnormal distribution, and for 3 or more groups in such cases, we used the Kruskal–Wallis test. The results are presented as a median (Me) with interquartile range [25th, 75th percentile]. To compare samples by qualitative attribute and to assess the proportion of occurrence of a characteristic/sign, we used the Fisher's exact test. Spearman's rank correlation coefficient was used for the correlation analysis. The differences were considered statistically significant at p < 0.05.
RESULTS
Cytokine study of the MS group patients (acute stage and remission)
No gender-related differences were registered in the average cytokine levels among participants from the MS group. We detected significant positive associations between age of the patients at the time of examination and concentration of IL6 (r= 0.36; p < 0.05), TNF-α (r = 0.35; p < 0.05) and sCD40L (r = 0.42, p < 0.05); duration of the disease did not correlate with cytokine level.
Compared to the control group, MS patients exhibited significantly increased mean levels of IL10 and IL33 (p < 0.001) upward trend of IL4 (p > 0.05) (tab. 3). Concentrations of IL1-β, IL17F, IL22, IL25 and TNF-α against the background of MS were significantly lower (p < 0.001) than in participants from the control group, and the level of IL23 was lower, but the difference did not reach significance (p > 0.05). The levels of IL6, IL17A, IL21, IL31, INF-γ and sCD40L did not differ in the compared groups.
The most common phenomenon registered was hyperproduction of IL33 (n = 20, 52.8%). Significantly less frequently, we registered peaking levels of IL17A, IL17F, IL21, IL31 (in 2.8, 5.6, 5.6 and 13.8% of cases, respectively). No other cytokine level exceeded the upper limit of the reference range. The level of IL17A, IL17F, IL21 has always been combined with an increase of that of IL33. Hyperproduction of IL31 was isolated only in one patient; in other participants, it was coupled with hyperproduction of IL33. Isolated growth of concentration
of IL33, on the contrary, was detected in most (14 (70%) of 20) patients, and in 6 it grew together with other cytokines, most often — four cases — with IL31. High levels of IL33 were significantly associated with increased concentration of IL17A (r = 0.38; p < 0.05), IL17F (r = 0.38; p < 0.05), IL21 (r = 0.54; p < 0.001) and IL31 (r = 0.68; p < 0.001).
During exacerbation of MS, the average level of IL10 was significantly higher than outside of this period (p < 0.01), and, the levels of IL4, IL23, IL31, IL33 and sCD40L tended to grow (p > 0.05). The mean values of IL1ß, IL6, IL17A, IL17F, IL21, IL22, IL25, TNF-α and INF-γ did not differ between the compared groups.
High levels of IL31 were registered significantly more often during exacerbations (in 42.8% and 6.9% of cases, respectively; p = 0.04), with IL33 production simultaneously on the rise (71.4 and 51.7%; p > 0.05). The level of IL33 was predominantly growing with other cytokines (IL17A, IL17F, IL21, IL31) (in 57.1 and 6.9% of cases, respectively; p = 0.008). We have also identified positive associations between MS exacerbations and high values of IL17A (r = 0.34; p < 0,05), IL17F (r = 0.34; p < 0.05) and IL31 (r = 0.41; p < 0,01). The combined hyperproduction of IL33 correlated significantly exacerbations of the disease (r = 0.53; p = 0.001).
Cytokine levels in MS patients depending on the clinical manifestations of reactivation of PHVI
In MS patients with clinical manifestations of PHVI, the average level of IL1ß, IL23 and IL33 was significantly higher than in those who did no have the infection reactivated (p < 0.05 and p < 0.01, respectively); we have also noted the upward trend for mean values of IL4 (p > 0.05; tab. 4). Concentrations of IL6, IL10, IL17A, IL17F, IL21, IL22, IL25, IL31, INF-γ, TNFa and sCD40L did not differ between the compared groups.
The frequency of growth of IL31 was similar in both groups of patients (16.7 and 11.1%; p > 0.05). As for IL33, its level was significantly more often (77.7%) higher against the background of reactivated PHVI than outside of this condition (33.3%; p = 0.008). Clinical signs of PHVI were detected somewhat more often when IL33 was growing together with other cytokines than alone (in 83.3 and 64.3% of cases, respectively); with IL 33 concentration at the normal level, PHVI manifested itself only in 25% of cases (p = 0.02). Hyperproduction of IL33 was significantly associated with reactivation of PHVI (r = 0.45; p = 0.006); we established no similar pattern for IL17A, IL17F, IL21 and IL31 (r = 0.17, r = 0.17, r = 0.24 and r = 0.08, respectively; p > 0.05 in all cases).
Isolated or combined increase of level of IL33 was significantly associated with frequent (more than once a year) manifestations of Herpes labialis (r = 0.42 and r = 0.38, p < 0.01 in both cases), and simultaneous growth of IL33 and other cytokines pointed to repeated episodes of herpes zoster in adulthood (r = 0.55; p < 0.001).
The amount of antibodies to the EBV IgG capsid protein significantly positively correlated only with concentration of IL1ß (r = 0.34; p < 0.05). We identified no correlations between the level of other cytokines and laboratory markers of herpetic infection.
DISCUSSION
The clinical significance of cytokine counting in MS patients is ambiguous. Some authors point to gender as a factor affecting their levels: IL31 and sCD40L was found increased in male MS patients [26], IL33 — in female MS patients [18]. Other researchers state that gender and age of the patients have no effect on serum concentration of IL33 [27], or report its increase mainly in elderly patients [28]. IL31 and sCD40L typically grow during the early (up to 5 years) period of the disease [19], which was established by other authors, too [21]: they discovered significant negative correlations between duration of the disease and levels of IL1ß, IL17, IL21, IL23, IL31 and IL33. In our study, we identified no differences in cytokine concentrations between men and women, there was no relationship with the duration of the disease found, and age of the patients positively correlated only with the values of IL6, TNFa and sCD40L.
Compared to the control group, MS patients had significantly higher concentrations of IL10, IL33, IL4 tending to grow, and simultaneously falling levels of IL1β, IL17F, IL22, IL25 and TNF-α. These results are somewhat consistent with data reported by other authors. In a controlled study that analyzed 15 cytokines (multiplexing), MS patients had increased blood plasma concentrations of IL4, IL33, sCD40L and decreased level of TNF-α, as well as high counts of IL1ß, IL10, IL33 and low level of sCD40L in the cerebrospinal fluid [17].
Low level of proinflammatory cytokines in MS patients is associated with the effect of MS DMDs [26, 29]. The majority of participants of our study (83.3%) were also taking MS DMDs. However, there is a study that involved RRMS patients that did not take such drugs [17]. In addition, another study reports high concentration of IL33 in 32 RRMS patients registered before they started therapy with glucocorticoids or MS DMDs [16]. A multiplex analysis of concentration of 41 cytokines in 56 naive MS patients revealed that the levels of IL2, IL4, IL7, IL8, IL17A, TNF-α and sCD40L drop compared to healthy donors [15].
During exacerbation of MS, levels of IL1ß, IL2, IL6, IL17, IL23, TNF-α, INF-γ typically grow and those of IL4 and IL10 drop [2, 3, 30]. However, some papers report that during this phase of the disease, the level of IL17 goes down while counts of TNF-α and IL10 remain on par with values registered in the control group [31], while RRMS patients in remission enjoy a significant decrease in the concentration of IL10 [32]. Participants of our study had the level of IL10 significantly higher during exacerbations, which also triggered hyperproduction of IL31 and growth of IL33 and other cytokines (IL17A, IL17F, IL21, IL31).
One of the main objectives of this study was to assess the effect of herpetic infection on cytokine levels in MS patients. In the literature available to us, we found no studies investigating the the relationship between production of cytokines and reactivation of herpes infection. The best researched connection is that between γ-herpesviruses, EBV [10, 33] and HHV-6 [7] in particular, and chronic latent infection in B cells and T cells of MS patients [12]. In adolescent patients predisposed to MS, these viruses cause development of the disease [11, 12, 33]. Relapses of MS may be associated with faults in control of EBV reactivation by CD8+T cells [34]. β-herpes CMV infection, on the contrary, can protect against MS and reduce the risk of its occurrence [35].
However, α-herpes viruses (HSV-1, HSV-2 and VZV) are partake in pathogenesis of MS. The viruses of this group can persists in neurons for a long time, periodically reactivate and replicate (lytic pattern), which triggers relapses with a short reproductive cycle and rapid destruction of the infected host cells [36]. In a case-control study, HSV-1 DNA was detected in peripheral blood monocytes of 45.1% of RRMS patients, while in healthy people this indicator was at 3.4% [37]. HSV-1 is more often found in the brain tissue of MS patients than in that of individuals without this disease [38].
In MS patients, monoinfection of herpesvirus is less common than the mixed variety. The most frequently diagnosed combination includes four herpesviruses: HSV-1 and 2 + VZV + EBV + CMV [39]. Development of clinical exacerbations of RRMS was established to be accompanied by reactivation of HSV-1 in peripheral blood monocytes [40]. HSV-1 seropositivity is associated with an increased risk of MS in individuals who have no DRB1*15 allele [41]. These data confirm the possible involvement of HSV-1 and HSV-2 and VZV in the development of MS and its exacerbations in patients specifically predisposed genetically.
Analyzing the clinical signs peculiar to the reactivated PHVI group, we identified a number of characteristic features that sum up to an earlier disease onset age and frequent exacerbations. This is consistent with the data reported by other researchers [42] and the results of our earlier work [39]. We detected significantly higher mean levels of IL1ß, IL23, IL33, and prevailing production of IL4 in the RRMS patients with clinical manifestations of PHVI that participated in this study. At the same time, IL1ß and IL23 did not rise in other groups. High values of IL33 were significantly more common in PHVI patients; the level of this protein was growing both alone and in combinations with IL17A, IL17F, IL21 or IL31. There was also a significant association between high levels of IL33 and clinical manifestations of recurrent infection that are typical for α-herpes viruses HSV-1 and HSV-2, and VZV.
Our results allow discussing the special character of the role played by cytokines in the pathogenesis of MS associated with herpes viruses, which is obviously linked to the realization of their biological functions. For example, IL10 has a powerful antiinflammatory effect and affects the innate and acquired immune response. It curtails production of IL1ß, IL6, IL8, IL12, IL23 and TNFa, which also possesses neuroprotective action effect [43]. Traditionally, increased level of IL10 in MS patients is linked to the early stages of remission of the disease, with production of IL17, IL23 and IL25 suppressed in the background [2]. Our data indicate that RRMS exacerbations may be accompanied by simultaneous growth of IL10 and proinflammatory cytokines.
The encountered differences may stem from our use of multiplexing technology to count the cytokines. Unlike uniplexing analysis technology, common earlier, multiplexing enables simultaneous assessment of a complex of molecules and not just individual indicators. For example, multiplexing allowed detecting growth of IL10, IL17 and IL23 in RRMS in remission [21].
At the same time, viral infections can trigger excessive synthesis of IL10, which is of particular importance if the patient has MS. In the active phase of inflammation, this protein is produced to limit the undesirable consequences of hyperactive innate immunity response to the pathogen [44]. However, evolving, viruses have learned to use the immunoregulatory function of IL10 to evade the host's immune system and thus up their chances of survival. With inflammation in the active phase, antiviral CD4 and CD8 T cells become the main sources of IL10 [45], but the protein, which suppresses the function of Th1 cells, reduces their ability to present the antigen. Increased synthesis of IL10 with prolonged activity of the antigen can deplete the stock of antiviral T cells, switch their phenotype and thus make them cells predominantly producing IL10 that are unable to activate upon repeated presentation of the antigen [44].
In addition, EBV encodes synthesis of the protein that is a homologue of human IL10 (viral homologue IL10 (EBV IL10), as well as synthesis of conventional IL10 (cIL10) [46]. EBV IL10, a late lytic phase protein encoded by the BCRF-1 gene, is approximately 80% homologous to human IL10. IL10 homologues allow the virus to escape immune response of the host or limit its action. Compared to IL10, EBV IL10 induces a much weaker STAT3 phosphorylation in the peripheral blood monocytes; it is less effective in suppressing inflammatory genes [47]. EBV IL10 degrades expression of CD163 on monocytes, which translates in inhibition of their polarization in M2 cells possessing antiinflammatory properties. Moreover, the process disrupts participation of monocytes in clearance of apoptotic cells, which, accumulated, promote secondary necrosis. It is believed that, with EBV infection in the background, IL10 synthesized by cells and EBV IL10 act simultaneously and in a functionally coordinated manner, helping the virus to stay in B lymphocytes for a long time and neutralize the antiviral potential of T cells [46]. EBV IL10 can activate В cells [48]. Both of these cytokines are found in CNS; they support the pool of B-cells with latent EBV infection, which locally stimulate pathogenic T cells. Given the presence of serological markers of EBV infection in all RRMS patients involved in this study, it is possible that these mechanisms shaped production of IL10 in our case, too.
IL17 is known to be one of the key cytokines in the pathogenesis of MS [2]. It also plays an important role in the immune response to viral infections [12]. Stimulated by HSV, Th17 cells produce IL17 [49]. The expression of KIR2DL2 receptor on NK cells in MS patients makes them more susceptible to HSV-1 infection [50]. The bulk of IL17A is released by KIR2DL2+NK cells [51]. In this study, we did not register differences in concentrations of IL17A and IL17F between RRMS and control groups; the levels did not differ during exacerbations and remissions and were not dependent on PHVI reaction or lack thereof.
IL23 also participates in pathogenesis of MS. Compared to healthy donors, RRMS patients have more serum IL23 [52]. Moreover, IL23 plays an important role in HSV infection cases. It induces proliferation of memory T cells [53] and can be detected as early as on the 3rd day of infection in the nerve ganglia of mice infected with HSV [54]. IL23 stimulates production of IL17 by NK cells, attracts neutrophils to the infection locus and supports local synthesis of antiinflammatory cytokines IL1β, IL6 and TNFα. We have registered a significant growth of IL23 in the reactivated HSV group, which was not the case for IL17.
IL31 belongs to the IL family; it is mainly synthesized by Th2 cells, and it is dependent on IL4 [55, 56]. IL31 acts through a heterodimeric receptor that comprises an IL31RA chain and a — chain of the oncostatin M receptor. The protein targets mast cells found in peripheral tissues innervated with minor nerve fibers, as well as in endoneural part of peripheral nerves, brain's tunic and blood vessels [57]. Various subpopulations of leukocytes, epithelial and stromal cells, spinal ganglia, keratinocytes and fibroblasts were found to express IL31RA [20, 58]. IL31 and its receptor play an important part in regulation of neuroinflammation. IL31 promotes tissue remodeling and inflammation through induction of IL6, chemokines and matrix metalloproteinases [59, 60]. Compared to healthy donors, RRMS patients had high level of IL31 in serum, which decreased during remissions [19, 52]. The patients involved in this study also had IL31 hyperproduced during exacerbations, but it was not related to PHVI reactivation.
IL33 is a member of the IL1 family, which includes IL1β and IL18. It plays an important role in pathogenesis of various diseases, including MS [55]. Compared to healthy donors, RRMS patients were found to have significantly higher level of blood serum IL33 [52]. The action of IL33 is realized through binding to its receptor, ST2, which can be soluble (pST2) and membrane-bound (ST2). The first form of the receptor is a decoy, it isolates free IL33 and thus locally restricts the activity of extracellular IL33, which allows avoiding undesirable consequences of inflammatory reactions [62]. IL33 affects various types of cells bearing ST2 receptors on their surfaces. The list includes eosinophiles and basophiles, mast cells, Th1 and Th2 lymphocytes, cytotoxic T lymphocytes, natural killers and Type 2 innate lymphocytes [63]. Membrane-bound ST2 activates the MyD88/NF-kB signal pathway reinforcing function of mast cells, Th2 cells, regulatory T cells and Type 2 innate lymphocytes [62].
It is believed that polymorphism of IL33 and ST2 play an important part in the development of MS. There are data suggesting connection between single nucleotide polymorphism rs1929992 in the gene of IL33 and various courses of MS [64]. Another controlled study reports no significant differences in the frequency of detection of three single nucleotide polymorphisms, IL4 (rs2070874), IL17A (rs2275913) and IL33 (rs7044343) [65]. Only the genetic polymorphism rs10204137 of IL33 receptor's gene was shown to have associations with MS against the background of high levels of this protein [66].
While IL33 is one of the best studied cytokines in the context of neural system pathologies and MS, its participation in neuroinflammation and neurodegeneration is ambiguous. It is expressed not only on astrocytes and oligodendrocytes but also on neurons and microglia cells [67]. ST2, the receptor of IL33, is mainly found on neurons and oligodendrocytes. The crossexpression of IL33 and ST2 on various CNS cells indicates the complexity of autocrine and paracrine mechanisms of IL33/ST2 signaling in CNS, in addition to the protein's immunomodulating action in cases of inflammation [67].
IL33 boosts invasion of CNS by immune cells from the blood flow and activation of the resident immune cells [55]. IL33 directly affects oligodendrocytes and activates astrocytes [67–69].
In MS patients, peripheral leukocytes and astrocytes are the important sources of IL33, which activates the microglia cells [16, 70]. IL33 released by the glia forces neighboring cells to produce inflammatory molecules that are detrimental to neurons [71, 72]. The glial maturation factor also makes astrocytes produce IL33 faster, and the protein acts synergistically with it and promotes synthesis of TNF-α by these cells [73]. Incubation of the mixes of astrocytes and neurons or only neurons with IL33 decreases their amount, rids neurons of their processes, destroys their network and shapes neurite-like changes of the cells' exterior [73].
IL33 also participates in damaging and disruption of reparation of myelin. Experiments on the co-cultures of myelinated cells of rat's CNS have shown that IL33, while not influencing the density of axons, can suppress their myelination significantly [67]. In MS patients, the levels of IL33 mRNA and IL33 itself are very high in the lesions [16]. Foci of inflammation were also found to host high local expression of ST2 in axons and damaged myelin, as opposed to its diffused distribution in the normal human brain cortex [67].
At the same time, there is an ongoing discussion about the neuroprotective effects of IL33. The plasma levels of IL33 were revealed to be increased in patients with mild MS, and blood concentration of this cytokine shown to correlate negatively with the amount of T2 hyperintensive lesions seen on MRI scans [21]. The growth of IL17A and IL33 in the blood serum of MS patients is not connected to EDSS [65]. Macrophages are known to actively participate in inflammatory processes with MS in the background [74]. IL33 modulates polarization of microglia into the M2 phenotype and promotes neuroprotection [75].
Administration of recombinant IL33 to mice with experimental autoimmune encephalomyelitis (EAE) after onset of the disease switches the immune response from proinflammatory, mediated by Th1 and Th17 cells, to antiinflammatory, dependent on Th2. M2 polarized macrophages produce less IL17 and INF-γ and more IL5 and IL13 [76]. In EAE cases, high levels of circulating IL33 are considered to constitute the mechanism self-limiting chronic inflammation. With low concentration, the effect produced by IL33 may be insufficient, which is compensated for by production of other cytokines, such as IL1. It seems like these mechanisms are universally important; they also play a part in prevention of generalized encephalitis with a herpes infection in the background, which is confirmed by the increased levels of IL1β and IL33 in the reactivated PHVI group.
Gender is also a factor. Experiments on SJL mice infected with EAE have shown that male subjects had the concentration of testosterone growing when they were immunized with myelin peptide (PLP139-151), which stimulates production of IL33 by mast cells with androgen receptor on their surface [77]. IL33 activates Type 2 innate lymphocytes producing IL13, which accumulate in lymph glands, brain tunics and CNS, and promote Th2 dependent protective response. In male specimen, ST2+ maste cells and basophils sensitive to IL33 boost polarization of Th2 cells through production of IL4 and IL13.
With testosterone level low, mast cells in female specimen express molecules of IL1β and TNF-α instead of IL33; moreover, its insufficient amount disallows activation of Type 2 innate lymphocytes. These lymphocytes, in turn, are known to help restore tissues, they actively express IL33's receptor on its surface and can produce IL4, IL5, IL9, IL13 [63, 78]. Without the suppressive effect of IL33, the encephalitogenic Th17 dependent immune response prevails in females. This response can be inhibited by administration of exogenous IL33.
Through activation of Type 2 innate lymphocytes, IL33 controls accumulation of regulatory T cells in the foci of inflammation, as well as their effector function and macrophage polarization [79]. IL33 also stimulates regulatory B cells that are important for maintaining peripheral tolerance and suppression of inflammatory autoimmune reactions. Administration of IL33 to mice increases the amount of B cells producing IL10 [80, 81].
IL33 participates in the innate immune response to tissue damage peculiar to infections. However, this cytokine appears in circulation and functions differently from IL1β and IL18 [79]. Typically, IL33 is released from cells as a biologically active full-sized molecule during necrosis or necroptosis, but not cell apoptosis [82, 83]. As opposed to IL1β and IL18, formation of a biologically active form of IL33 does not require preconditioning with caspase 1 and participation of inflammasome. On the contrary, its molecule can undergo apoptosis-associated scission by caspases 3 and 7, which makes half-life of IL33 shorter and decreases its biological activity [84]. Inactivation of IL33 via caspases suppresses the immune response, not enhances it. IL33 is also contained in the cell nucleus as a chromatin-associated factor, which is rapidly released during their necrosis [85, 86]. Interacting directly with NF-kB, nuclear IL33 isolates it and prevents signal transmission, acting as a nuclear transcription suppressor and thereby reducing the proinflammatory activity of cells [87].
Other enzymes, such as neutrophil serine proteases, cathepsin G and elastase, are capable of sciccioning IL33 and, unlike caspases, increase the biological activity of cut forms by 10–30 times compared to the full-size form [88, 89]. Mast cellspecific chymase and tryptase generate a form of IL33 with enhanced ability to activate Type 2 innate lymphocytes [90], which, unlike Type 1 and Type 3 innate lymphocytes, are not present in active lesions in MS patients [12] but participate in HSV-IL2-induced demyelination of the CNS, as shown in a mice model of MS [91].
Necroptosis was found to develop against the background of MS [92]. Cortical lesion samples from a patient with caspase 8 activation defect contained mediators RIPK1, RIPK3, MLKL characteristic of it. The mechanisms of necroptosis induced by TNF-a lead to degeneration of oligodendrocytes, and inhibition of receptor of protein kinase 1 (RIPK1) prevents their death [92].
Necrosis and necroptosis of cells are also peculiar to an HSV infection [93]. Such an infection makes various molecules, including the viral DNA genome, RNA types obtained by transcription, non-coding cellular RNAs, activate transmembrane (toll-like) and cytosolic pattern recognition receptors associated with pathogens, and they transmit signals through individual adapter proteins to initiate innate antiviral immunity. This leads to the production of cytokines, cell necroptosis through mechanisms similar to those in MS, which are linked with protein kinase-3 by its receptor (RIPK3), and activation of the NF-kB pathway [94].
Programmed necrotic cell death limits virus replication and virion propagation [95]. In such situations, IL33 appear in the circulation and acts as an alarm signal. It initiates biological effects aimed at elimination of threats to the body, including through the of production of pro-inflammatory cytokines [55]. Activation of NF-kB via toll-like receptor signaling pathways leads to secretion of TNF-α and IL1-β, which mediate the transcription of IL33 [96, 97]. IL33 itself also induces mRNA expression in TNF-α and IL1-β microglia.
In many cases, the combined increase of IL33 and IL31 correlates with the severity of signs of inflammation [55]. It is believed that IL33 secreted as a result of cell damage promotes IL4-dependent release of IL31 by Th2 cells [98]. These data may explain the association of IL33 and IL31 we have registered during MS exacerbations, as well as the joint production of IL33 and IL1β, IL4, IL23 in the reactivated herpes infection group.
There is a delicate balance between direct damage (necrosis) to cells in an HSV infection case and an immune response to it [99]. In infection recurrence, excessive cytokine production can not only limit the spread of the virus but also activate mechanisms of cell necroptosis with organ dysfunction, which is especially important for the CNS, where tissue regeneration capabilities are limited [12, 99, 100].
The reflection of these processes, apparently, is the increase in the concentration of IL33 detected by us mainly during reactivation of HSV. It is possible that α-herpes viruses, HSV-1 and HSV-2, as well as VZV, should not be considered an etiological cause of MS in most cases; rather, they may be viewed as a factor contributing to excessive activation of the immune system in genetically predisposed individuals, which is crucial for progression of demyelinating diseases. Processes such as molecular mimicry, regulation of endogenous retroviruses, or impaired remyelination can be mediated by this pathogen [101], and frequent relapses of this infection create conditions for the progression of neurodegeneration and transition of RRMS into SPMS.
Our data are consistent with the opinion of other researchers about the importance of the IL31/IL33/ST2 axis in the development of MS, which is possibly based on its participation in demyelination in the CNS, in contrast to the protective antiinflammatory function of the injected recombinant IL33. Moreover, they expand our understanding of the involvement of herpetic infections in immuno-inflammatory reactions and processes of demyelination or remyelination disorders characteristic of MS. Further investigation of functioning of IL10, IL31 and IL33/ST2 systems, which are an important link between immune cells, the nervous system and the epithelial tissues, under herpes viral load, will be crucial for the development of new approaches to treatment of MS [102].
CONCLUSION
The results of this work indicate the important involvement of IL10, IL31 and IL33 in the pathogenesis of MS, but their role is ambiguous. We believe that the increase of level of IL10 in MS patients, during exacerbations and otherwise, largely depends on the realization of its biological role in inflammation caused by an EBV infection. On the contrary, the combined growth of the levels of IL1ß, IL23 and especially IL33, registered during reactivation of PHVI, is apparently triggered by HSV and VZV.
Our study was exploratory in nature, we did not formally assess the sample size and adjusted for multiple comparisons, therefore, the results/trends learned should be confirmed in future works. Nevertheless, this study reflects the data of real clinical practice of managing patients with RRMS, which brings us closer to deciphering the mechanisms involved in exacerbation of MS and progression of the disease.