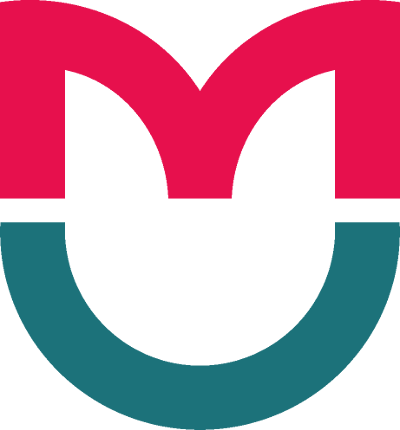
This article is an open access article distributed under the terms and conditions of the Creative Commons Attribution license (CC BY).
METHOD
Foveal microsurgical reconstruction technique for macular hole
Pirogov Russian National Research Medical University, Moscow, Russia
Correspondence should be addressed: Khristo Periklovich Takhchidi
Volokolamskoe shosse, 30, korp. 2, Moscow, 123182, Russia; moc.liamg@1031tph
Compliance with ethical standards: the study was approved by the Ethics Committee of the Pirogov Russian National Research Medical University (protocol № 224 dated 19 December 2022). All patients submitted the informed consent to surgical treatment and personal data processing.
Idiopathic macular hole is a foveal defect affecting all neuroretinal layers and characterized by decreased visual acuity, central scotoma and metamorphopsia [1–4]. According to the literature data, the macular hole incidence is about 3–5 cases per 1000 population aged over 55, it is more prevalent among women aged 60–70. The disorder is unilateral in 80% of cases [5].
In 2013, the researchers of the International Vitreomacular Traction Study Group (IVTSG) proposed an optical coherence tomography (OCT)-based anatomic classification system for assessment of the central retina condition in individuals with macular holes. According to the classification, macular holes are classified as small (≤ 250 µm), medium (250–400 µm), and large (>400 µm) based on minimal diameter [6, 7].
The internal limiting membrane (ILM) peeling with subsequent gas-air mixture tamponade had been considered the gold standard treatment for full-thickness macular hole [8]. To further improve the efficacy, modified surgical approaches to treatment of macular holes were proposed: mechanical approximation of the hole edges, ILM peeling followed by the hole sealing with inverted ILM flap, amniotic membrane plug, anterior lens capsule transplantation, ILM preservation [9–12].
In recent years, the use of platelet-rich autoplasma is one of the promising directions of the macular hole surgery. Two methods to obtain plasma are currently being actively used in treatment of retinal disorders: PRP (platеlet-rich plasma with the platelet content 3–4 times higher compared to baseline blood levels) and ACP (autologous conditioned plasma almost deprived of white blood cells and having an increased platelet concentration that is 2–3 times higher compared to baseline blood levels). The local use of autologous factors in macular surgery makes it possible to achieve good anatomic and functional outcomes and minimize retinal tissue injury during surgery [6, 13–15]. However, despite high rate of macular hole closure (78–96%) [16, 17], the quest for restoration of foveal microarchitectonics and, therefore, for improvement of visual functions, currently remains a pressing issue.
The study was aimed to develop a new technique for foveal reconstruction in individuals with macular holes based on the use of autologous conditioned plasma.
METHODS
The study involved 9 patients (10 eyes) aged 62–76 (average age 66.1 ± 7.41 years), who underwent surgery due to idiopathic full-thickness macular hole at the Ophthalmology Research Center, Pirogov Russian National Research Medical University.
All patients underwent comprehensive eye examination involving the use of standard (visometry to determine uncorrected visual acuity (UCVA) and best-corrected visual acuity (BCVA), pneumatonometry, biomicroophthalmoscopy with a MaxField indirect lens (Ocular Inc.; USA)) and specific assessment methods, such as spectral-domain optical coherence tomography (SD-OCT) performed with the Spectralis HRA+OCT system (Spectralis HRA+OCT, Heidelberg Engineering, Module, OCT-2 85,000 Hz, Inc.; Germany), microperimetry (MAIA, CenterVue; Italy).
Inclusion criteria: idiopathic full-thickness macular hole. Exclusion criteria: concomitant eye disorder (age-related macular degeneration, glaucoma, partial optic atrophy, vascular disorders, diabetic retinopathy, intraocular mass, retinal detachment, corneal scars or opacities), traumatic macular hole, macular hole duration exceeding 6 months.
The macular hole duration before surgery was between 3 weeks and 5 months. The follow-up period was 3–16 months. The study results were processed using standard descriptive statistical tools of the Microsoft Office Excel. The data were provided as М ± σ, where М was the mean, σ was the standard deviation.
Assessment showed that UCVA was 0.18 ± 0.13 and BCVA was 0.3 ± 0.17. OCT revealed macular holes with intraretinal cysts in all cases. The maximum macular hole diameter was 759.2 ± 174.7 µm, the minimum diameter was 343.4 ± 96.6 µm, the average central retinal thickness (CRT) was 416 ± 65.3 µm. According to the IVTSG classification, the patients were divided into groups as follows: group with ≤ 250 µm — 0 eyes; group with 251–400 µm — 7 eyes; group with ≥ 401 µm — 3 eyes. Microperimetry showed that the average retinal sensitivity (RS) in the studied group was 22.7 ± 1.52 dB.
All the patients underwent treatment involving the use of the developed technique: in the first phase phacoemulsification and intraocular lens implantation was performed in patients with lens opacities. Then three-port 27G vitrectomy with isolation and removal of the posterior hyaloid membrane was performed. After that the ILM was stained and removed. After the ILM removal, at least 1/3 of the vitreous cavity volume was substituted with the perfluorocarbon liquid (PFCL) that filled the posterior pole of the eye and hermetically “sealed” the macular hole. As a result, the hermetically sealed intraocular fluid pressurized by the irrigation fluid and the pressure head of PFCL stayed in the macular hole intrawound area. Then the fluid was passively evacuated from the macular hole area with an extrusion needle, the irrigation fluid pressure was 15 mm Hg. The vertical needle bevel was brought close to the hole area, not touching the retina. Owing to the differences in viscosity between PFCL and intraocular fluid, intraocular fluid, that was a priority when performing evacuation from the hole area, turned out to be more motile in terms of competitive aspiration (fig. 1). As the wound fluid was evacuated, the negative pressure (“mild micro-vacuum”) occurred in the hole area, which ensured even and atraumatic approximation of the ragged macular hole walls around the perimeter of the wound. To ensure visual control of the process of bringing together the hole walls, we lifted the needle tip above the hole area. When the hole walls fail to fuse completely, the manipulation involving the wound fluid aspiration can be repeated many times to achieve the necessary outcome (fusion). In case of unintended aspiration (“adhesion”) of the inner retinal surface of the macular hole edges, it is necessary to stop passive aspiration by closing the extrusion needle hole with the finger and slowly lifting the needle tip over the retina perpendicularly to the surface. As a result of this action, the retinal surface uniformly pressurized by PFCL gravity easily peels off the needle bevel without damaging the retinal tissue. This procedure is associated with no risk.
When exposed to negative pressure in the hole area, the aggregate foveal tissue of the fused macular hole walls was held in this position due to the PFCL gravity for at least 10 min (hole retention time) (fig. 2). During this time we intraoperatively prepared ACP using the Arthrex ACP double-syringe system. A total of 15 mL of the patient’s venous blood were collected in the Arthrex ACP syringe. After blood collection, the syringe was installed in the ROTOFIX 32A centrifuge (Hettich; Germany) for further centrifugation for 5 min at 1700 rpm. A total of 0.2–0.4 mL of ACP was taken up in the micro-syringe for application to the macular hole area. After the required time of the macular hole retention in the closed state (at least 10 min) was over, PFCL was substituted with the irrigation fluid. At this point the fused macular hole walls temporarily stayed closed due to weakening of the centrifugal force of the stretched retinal tissue elasticity. This time was enough to apply the prepared autoplasma forming a hermetic plaque sealing the hole area on the surface of the macular area of the retina. The autoplasma layers were applied to the macular area until a faint translucent plaque emerged (fig. 3А). After that the irrigation fluid was substituted with air.
RESULTS
After a month of postoperative follow up (eight patients, 10 eyes) UCVA increased to 0.45 ± 0.21, BCVA was 0.54 ± 0.13. Microperimetry showed that the average RS was 24.86 ± 1.53 dB. According to the OCT data, the average CRT decreased from 416 ± 65.3 µm to 299.3 ± 49.2 µm.
On the follow-up examination three months later UCVA increased to 0.53 ± 0.22 and BCVA increased to 0.66 ± 0.12. The average RS was 25.27 ± 3.3 dB. The average CRT decreased to 290.3 ± 46.3 µm.
After six months of follow up (seven patients, eight eyes) UCVA was 0.53 ± 0.3, BCVA was 0.73 ± 0.15. The average CRT was 274.6 ± 37.6 µm. The average RS was 25.7 ± 2.11 dB.
In a year (five patients, six eyes), UCVA was 0.42 ± 0.33, BCVA was 0.75 ± 0.21. According to the OCT data, the average CRT was 285.2 ± 31.8 µm. The average RS was 25.4 ± 2.32 dB.
The longest follow-up period (up to 16 months) was reported for one patient (one eye) with preoperative UCVA of 0.05 and BCVA of 0.1. CRT was 450 µm. The average RS was 22.7 dB. On the follow-up examination 16 months after surgery UCVA and BCVA increased to 0.1 and 0.8, respectively. CRT decreased to 336 µm. The average RS was 27.8 dB.
As a result of surgical treatment, OCT showed complete macular hole closure and foveal structure restoration in all cases. According to the OCT findings, regression of the intraretinal cysts diagnosed before surgical intervention was observed in the early postoperative period (after 6 h and 3 days) (fig. 4, fig. 5).
No postoperative complications were reported in any case; there were no relapses throughout the postoperative follow-up period.
DISCUSSION
Part of the foveal tissue dies when the macular hole is formed. The remaining highly functional foveal tissue of the retina provides a morphofunctional basis for visual function restoration. However, after the macular rupture, the surviving part of the foveal tissue is shifted from its microanatomical/ microhistological site. Therefore, microarchitectonics of the remaining foveal tissue part is impaired and, consequently, visual functions are reduced. The situation of macular tissue disorganization is aggravated by edema and microcysts, the traumatic inflammation and degeneration sequelae. Furthermore, the presence of the circular tissue defect in the fovea creates the priority for centrifugal forces related to the surviving retinal tissue elasticity, which more and more actively stretch the remaining foveal tissue and shift it towards the macular area periphery as time passes.
Some authors have reported the methods of mechanical approximation of the macular hole edges by smoothing the retina surrounding the hole with the vitreous spatula from the periphery to the center [18] or by using the retinal “massage” with the silicone cannula [19] for repositioning of the macular hole edges. However, these manipulations dramatically increase the risk of reactive retinal edema, retinal pigment epithelial dislocation and spontaneous retinal detachment along the hole edge, which, in turn, can result in the foveal tissue loss and reduced functional potential of the retina after the anatomical fusion [20–22].
The proposed technique of fluid removal from the macular hole area by passive aspiration involving hermetic sealing of the area with the PFCL ensures manageable and maximally atraumatic fluid evacuation from the hole and the surrounding macular tissues of the retina. The fluid removed by this method creates the steadily increasing negative pressure, microvacuum that generates centripetal forces primarily pulling up and fusing the most functionally valuable parietal edge foveal tissue remains, in the intrawound space of the macular hole. At the same time, the fused macular hole walls are fixed in the foveal area by the PFCL gravity.
Thus, the “micro-vacuum” steadily increasing around the perimeter of the circular macular hole results in simultaneous minimally traumatic retraction, smoothing and fusion of the opposite macular hole walls behind the torn parts of the surviving foveal tissue. Along with this process, exposure to the PFCL gravity results in the fact that the fused remains of the macular hole wall tissue are fixed and retained in the foveal area. This micro-manipulation brings the surviving foveal tissue to the baseline microanatomical/microhistological position.
The time of the hole wall retention in the closed state by the PFCL gravity proposed by the technique (at least 10 min) makes it possible to significantly weaken the centrifugal forces associated with elasticity in the stretched retinal tissue. This important feature enables temporary retention of the fused macular hole walls in the closed state after the PFCL removal. Thus, there is enough time to cover the wound surface with ACP to achieve the hermetic plaque formation. The following takes place when applying ACP to the sealed wound along with the process of finalizing the plaque formation in the macular hole intrawound space.
Under the forming ACP plaque, after the holding force effect termination (fixing force, PFCL gravity), the remaining centrifugal forces of the retinal tissue elasticity are activated, which begin to stretch the closed walls of the macular hole from the center to the periphery. As a result, partial micro-separation of the macular wound walls occurs under the hermetic plaque. When micro-separation of the macular wound walls occurs, negative pressure is created in the resulting wound micro-lumen that increases with increasing micro-lumen size and forms a microvacuum effect ensuring suction of the liquid fractions of the entirely noncoagulated ACP. This process changes over time and continues at the level of microscopic alterations.
ACP soaked up into the micro-lumen (fig. 3B) ensures the best and fullest microscopic coverage of the glued uneven surfaces of the torn macular tissue. Furthermore, autoplasm leaks into irregularities, micro-cracks and micro-cavities. Thus, the maximum ACP (“biological glue”) coverage of the fused (glued) macular hole wound surfaces is achieved.
An adhesive biological insert (clot) of coagulated ACP is formed between the fused surfaces. The tomography scans (fig. 5), where we managed to obtain OCT images with air tamponade 6 h after surgery, clearly demonstrate that ACP fills the entire intrawound space, leaking into the wound wall irregularities. The ACP insert having a branched configuration duplicates the macular hole wall uneven curvature. This confirms the mechanism of micro-vacuum ACP suction from the plaque covering the macular hole. Moreover, already at this stage, the lack of parietal retinal cysts and cavities attracts attention, which demonstrates effective evacuation of both wound fluid and tissue fluid of patiental macular hole areas, including retinal cysts and hole wall cavities, from the macular hole.
On day three, all these signs become more prominent, which confirms the described foveal reconstruction mechanisms (fig. 4). In the majority of cases, the insert transforms into a macular tear junction (adhesion line), which is associated with the macular profile formation and morphological organization of the macular/foveolar area tissue structure. The macular/foveolar area architectonics is restored. The images make it possible to see the processes of retinal segmentation and restoration of the most important retinal structures: foveal profile, ellipsoid zone, external limiting membrane, outer plexiform layer, inner plexiform layer.
In the long-term period, the architectonics restoration is completed and a soft scar is formed in the fovea at the site of the tear, around which the macular/foveolar retinal area framework structures fuse. The surviving neuronal cell structures of the fovea tend to occupy their normal microanatomical/ microhistological positions, thereby ensuring restoration of preserved visual potential of the retina. Thus, the process of foveal architectonics micro-reconstruction is completed.
Along with the adhesive potential, the insert contains an equally significant range of natural biological substances ensuring the regeneration and reparation potential. In fact, the proposed technique is very close to micro-mechanisms underlying the natural tissue restoration process that ensures continuous physiological self-repair of the tissue microdamage occurring throughout the human body’s active life.
CONCLUSIONS
1. The use of mild micro-vacuum for atraumatic fusion of the remaining foveal tissue of the retina, as well as for the maximum coverage of uneven surfaces of the torn macular tissue with the adhesive compound, ensures optimal foveal microarchitectonics reconstruction. 2. Understanding of the micro-processes associated with the proposed technology of the macular hole wall fusion (adhesion) results in the possibility of improving the technique and achieving the better and more full-fledged restoration of visual functions. 3. Bringing the techniques closer to the natural processes of the living tissue self-repair enables the optimal use of body’s resources for restoration of damaged foveal microstructures and vital functions. 4. The more nuanced understanding of micro-processes associated with manipulations in the living tissue microworld opens up new opportunities for the development of clinical medicine dealing with microworld of the living human.