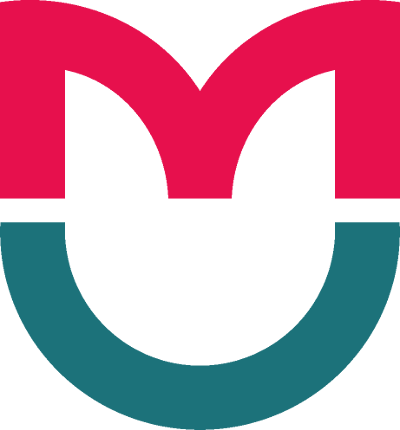
OPINION
Interferon signature in the development of SLE: molecular mechanisms, approaches to diagnosis and treatment
1 Shemyakin and Ovchinnikov Institute of Bioorganic Chemistry Russian Academy of Sciences, Moscow, Russia
2 Pirogov Russian National Research Medical University, Moscow, Russia
3 LLC MiLaboratory, Moscow, Russia
4 Department of Rheumatology, City Clinical Hospital No. 52 of the Department of Health, Moscow, Russia
Correspondence should be addressed: Olga V. Britanova
Miklouho-Maklaya, s. 16/10, 117997, Moscow, Russia; moc.liamg@natirblo
Financing: the study was supported by a grant from the Moscow Government (NIP No. 2412-63/22-1 dated May 13, 2022), sponsored by the Moscow Center for Innovative Technologies in Healthcare.
Author contribution: Myshkin MYu — literature analysis; Mutovina ZYu, Shagina IA — data collection in the field of rheumatology and medicine; Chudakov DM — concept, Turchaninova MA — analysis and interpretation of scientific data; Ryazantseva EV, Kazhdan MA — manuscript proofreading, Britanova OV, Nakonechnaya TO — literature analysis and manuscript preparation.
Epidemiological significance
The incidence of SLE varies from 4 to 250 cases per 100,000 population, the incidence rate depends on the region, ethnic composition of the population, gender and age [1, 23].
The risk of SLE in women is 8–10 times higher than in men; women have a higher risk during their reproductive years of 16–25 years, while SLE activity is expected during pregnancy and the puerperium [1–3].
Mortality in SLE is 4–5 times higher than in the the population at all; Possible causes of death in patients with SLE include infection (30%), neuropsychiatric disorders (15%), renal failure (14%) and cardiopulmonary damage (8%) [1].
Brief overview of the disease
Systemic lupus erythematosus (SLE) is an autoimmune disease in which the human immune system perceives host connective tissue cells as foreign [4]. The central mechanism of the immunopathology of SLE is a violation of immunological tolerance, leading to uncontrolled activation of B-cells response, the development of which is determined by a combination of genetic and epigenetic predisposition, environmental factors (ultraviolet radiation, viral infections, etc.) and intestinal dysbiosis [5].
Dendritic cells play a central role in the production of type I interferon and influence the clearance and sensitivity of nucleic acids (NAs) and immune complexes, known autoantigens in lupus (fig. 1). In fact, endogenous and extrinsic nucleic acids are the major antigenic stimulus in SLE. Autoantibodies targeting nucleic acid-bound antigens are one of the hallmarks of the disease. Apoptosis and NETosis (NET) may be the main source of such antigens. Excessive and impaired NET degradation is associated with lupus severity, lupus nephritis, anti-dsDNA antibodies, and complement consumption.
The strategic goal of SLE treatment is to achieve a state of remission or low activity [6–8].
Despite the increase in life expectancy of patients with SLE, associated primarily with improved tactics of using glucocorticoids (GCs) and immunosuppressive drugs, the incidence of deaths remains high, and adequate control of inflammatory diseases is observed in no more than half of patients [9]. Progress in basic research contributes to a better understanding of the pathogenesis of SLE and provides a conceptual basis for the development of new approaches to the pharmacotherapy of SLE [8, 10–11].
Diagnostics
In clinical practice, clinical complaints and manifestations in combination with hematological and immunological disorders are assessed to diagnose SLE [12]
In 2012, the SLICC/ACR diagnostic criteria for SLE were developed: the diagnosis is considered established if 4 criteria are present, of which one criterion must be clinical and the other immunological.
The EULAR/ACR (2019) [13] criteria are used to classify SLE, with sensitivity and specificity ranging from 96.1% to 93.4% [14].
The role of T and B lymphocytes in the pathogenesis of SLE
The causes of SLE in adults can vary, including genetics, hormonal imbalances, past infection, and environmental factors. Often in SLE there is an increased circulation of apoptotic bodies formed after cell death. The engulfment of cell bodies by dendritic or B cells can result in the presentation of self-antigens on their surface in the MHC class II complex, leading to T cell destruction and increased inflammation.
A study of 41 patients with active SLE and 101 healthy donors showed that the proportion of both CD4+CD28– and CD8+CD38+HLADR+ T cells with effector activity was significantly increased in SLE [15]. There is a shift toward a Th1716 inflammatory response with a decrease in the proportion of Tregs [16, 17].
Increased levels of expression of interferon-induced genes or type I interferon “signature” are found in the mononuclear fraction of blood, which confirms the key role of the innate immune system in the pathogenesis of SLE [18, 19]. An increased type I interferon (IFN) signature has been reported to be found in approximately 75% of adult patients and 90% of pediatric patients [20].
The role of interferons in the development of the disease
Recently, special attention has been paid to the regulation of interferon production in SLE [12, 21, 22].
Interferon is a cytokine produced under normal conditions in response to viral infection and has effects such as regulation of immunity, antiviral and antitumor activity. Depending on the sequence of the first protein, the cognate receptor, the gene locus and the cell type responsible for its production, IFN is mainly classified into three types.
– Type I IFN (IFN-α and IFN-β, -ϵ, -κ and -ω);
– IFN type II (IFN-γ);
– IFN type III (IFN-λ).
Many studies have shown the phenomenon of IFN-α dominance in SLE, but there is evidence that the IFN-γ signature may occur in the early stages of SLE and play an important role in the development of lupus nephritis [23] and, in general, IFN-γ levels are higher in the serum of patients with SLE than in healthy people [24, 25], and the pattern is that there is an abnormal accumulation of IFN-γ long before the diagnosis of SLE and before the appearance of autoantibodies and IFN-α.
IFN-γ levels and their gene boundaries have also been shown to increase with type I IFN activation in SLE patients [26, 27].
IFN-γ is a pleiotropic type II IFN that is primarily produced by effector Th1 CD4+ T cells, cytotoxic CD8+ T cells and NK cells and to a lesser extent by other cell types such as dendritic cells (DCs), macrophages and B cells [28]. IFN-γ binds to IFN-γ receptors (IFNG-R), which is expressed in most cells and activates Janus kinase 1 (JAK1) and JAK2 in canonical pathway, that lead to phosphorylation of STAT1 homodimers and binding to the IFN-γ activation site (GAS) for gene transcription [29]. Moreover, IFN-γ may also play a role in signal transduction through non-canonical pathways. There is overlap (crosstalk) between type I and type II inducible genes, and signaling pathways may be shared between them. Each type of interferon induces the production of the other, which ultimately leads to stimulation from the other side and a mixed signature [29].
IFN-α is a pleiotropic cytokine related to type I IFN that is widely used in patients with certain risk factors and viral diseases. IFN-α can influence tumor cell functions through several principles. In addition, these cytokines can mediate the differentiation and activity of host immune cells.
Type I IFN is critical. At least 10% of the genes of the human body take part for rergulation IFN type I, the expression of which depends on the cell type, cellular distribution of receptors and the nature of activation stimuli [30]. At the same time, against a viral background, the controlled synthesis of type I IFN is important in maintaining immune homeostasis by inducing the differentiation of B cells into plasma cells, synthesizing antiviral antibodies and generating B regulatory cells.
Plasmacytoid dendritic cells (pDCs) are the focus of attention in SLE [31]. Although almost all cells containing a nucleus are capable of synthesizing and activating type I IFN, its main source is pDCs, which generate it 1000 times more powerfully than other cells. Each pDC can produce up to 109 IFN-α molecules in 12 hours. This fact, as well as the unevenness of IFN-α compared to IFN-β in the blood in SLE, confirms that pDCs are the main cellular source of IFN-α in SLE. Accordingly, pDC deficiency has been shown to ameliorate disease in mouse models [32, 33]. Although other cell types, including macrophages and fibroblasts, are also known types of IFN I, these cells exclusively synthesize IFN-β. However, isolation of IFN-α-producing PDCs from the blood and tissues of SLE patients remains stringent.
The leading mechanism of activation of type I IFN synthesis in SLE is associated with impaired nucleic acid (NA) clearance.
Type I IFN production primarily triggers the activation of NK-binding receptors, which are released from medical apoptotic and non-totic (NET) cells. The NK-binding receptor group includes endosomal toll-like receptors (TLRs) 3, 4, 7, and 9, cytosolic sensor cyclic GMP-AMP synthase (cGAS), and RNA sensor RIG-I-like receptors (RLRs)-MAVS[34]. Under normal conditions, these NK sensing pathways are tightly regulated and create the requirement for a normal antiviral response [34, 35], but many patients with SLE have chronic hyperactivity of these pathways. NCs themselves are capable of producing IFN, and can also be included in the so-called “interferonogenic” immune complexes (IC). Interferonogenic IC means complexes consisting of NK, NK-binding proteins and antinuclear antibodies.
Impaired clearance and the formation of complexes in the form of the NETs method is very typical of SLE, as well as a weakening of the function of extracellular DNase I. In turn, NK and IR, binding to TLR7 and TLR9, localized in the endosomes of the PDK and induce the synthesis of type I IFN (fig. 2). The role of TLR7 in SLE is well conserved, as its overexpression is associated with a hard form of lupus in mice, and inhibition of TLR7 is protective [36].
Additional stimuli for the synthesis of type I IFN are mitochondrial DNA, a complex consisting of the cationic antimicrobial peptide LL37 and DNA, and the HMGB1 protein (chromosomal high mobility group block protein 1).
Study of interferons and interferon signatures in the clinic
Highly sensitive methods for determining IFN-α itself in blood serum have been developed, the results of which generally correlate with the parameters of gene expression of IFN type I [34, 37].
In all studies, the effectiveness of type I IFN overproduction is based on the analysis of interferon signals [38] by the expression of various genes (IFI27, IFI44, IFI44L, RSAD2, etc.) using PCR recently. Other approaches to measure the interferon signature include microarray technologies and the highly sensitive NanoString system using probes, which allow analysis of modern genes [39].
Since hyperactivation of the type I IFN signaling system is a feature not only of SLE, routine development of assessments of IFN signaling is also being carried out in Russia [40–42]. A multiparameter diagnostic test system has been patented, which can be used to determine the levels of mRNA of the human RIG-1, IFIT-1, IFIH-1 genes in a biological sample [43].
The question of the necessity and sufficient set of genes remains unresolved; expression should be assessed, as well as a unified method for calculating the interferon index.
Should be emphasized that the results of methods for determining the interferon signature depends on material (whole blood, cell population) and quantitative type I IFN genes. In addition, patterns of gene expression induced by IFN types I, II and III are observed. There is evidence that the expression of some IFN-responsive genes may reflect SLE activity [44, 45], but this has not been confirmed in more recent studies [46]. It has been established that overproduction of IFN-α is associated with the detection of “lupus” autoantibodies, primarily to RNAcontaining antigens [47–51], but their level does not correlate with the activity of SLE and does not change during therapy.
It is noteworthy that in SLE, natural autoantibodies to type I IFN are present, as a rule, in patients with low disease activity [52], and in COVID-19, on the contrary, in patients with severe infection [53]. These data reflect the primary role of type I IFN in the development of an effective antiviral immune response in patients with COVID-19 and provide the basis for deciphering the relationship between viral infection and autoimmunity in general.
It is noteworthy that hyperproduction of type I IFN in SLE is associated with the development of a wide range of diseases, on the one hand, observed during viral infections, and on the other, characteristic of SLE. These include fever, weakness, myalgia, arthralgia, headaches, pleurisy, as well as hematological disorders (anemia, neutropenia, lymphopenia, thrombocytopenia), damage to the skin, joints, lower extremities and nervous system (CNS). For example, when study target organ biopsy obtained from patients with SLE, an increased IFN signature was found to correlate with skin lesion activity [54, 55], exist in synovial tissue from patients with arthritis [56], in kidney tissue from lupus nephritis [57], and in the cerebrospinal fluid in patients with central nervous system lesions [58].
The recent SPOCS study (SLE Prospective Observational Cohort Study) characterized patients with high disease activity and/ or elevated type I interferon levels. As shown, patients with high levels of IFN symptoms are, firstly, younger in age and diagnosed later.And secondly, in such patients there was a predominance of cutaneous, immunological and hematological manifestations compared with patients with low levels of type I IFN [59].
Interferons in the pharmacotherapy of SLE
A body of evidence obtained from basic and medical research provides grounds for the development of new pharmacotherapy options for SLE using monoclonal antibodies (mAbs) that block the activity of type I IFN or its receptors [60–62] (5-oligoadenylate synthesis, MX1 — IFN-induced GTP-binding protein 1, P — phosphate [66]" data-note="" >fig. 3).
Several biologics are currently used to block type I IFN in SLE. The main ones include:
- Anakinra: A recombinant interleukin-1 (IL1) receptor antagonist that may inhibit interferon-activated signaling pathways. Clinical data on its effectiveness in SLE are limited, but some studies show improvement in patients with refractory forms of the disease. The response to therapy may be variable.
- Anaxifumab (Anifrolumab): Monoclonal antibody that blocks type I interferon receptors (IFNAR1 (Interferon receptor alpha and beta subunit 1)). Anaxifumab is intended to reduce the activity of the type I interferon signaling pathway. In phase III studies (TULP-1 and TULP-2), anaxifumab demonstrated improvement in patients with SLE. In TULIP-2, improvement in SRI-4 (systemic lupus erythematosus responder index) was observed in 47.8% of patients receiving anaxifumab, compared with 31.5% in the placebo group.
- Belimumab: Although belimumab is primarily aimed at inhibiting B cells, it also affects signaling pathways associated with interferons and may reduce their activity. Belimumab has received widespread acceptance and approval for the treatment of SLE. In phase III clinical trials (BLISS-52 and BLISS-76), approximately 43–58% of patients experienced further improvement compared to 34–44% in the placebo group.
Among these drugs, anifrolumab (AFM) [63, 64] and belimumab [65] occupy a special place.
AFM induces the internalization of IFNAR1, thereby reducing its membrane expression, which is necessary for the creation of a multifunctional IFN receptor consisting of two subunits — IFNAR1 and IFNAR2. The APM molecule is specially designed with a triple mutation L234F/L235E/P331S in the immunoglobulin chain gene, which leads to a decrease in the connection of APM molecules with membrane cellular Fc receptors. As a result, when introduced into the human body, APM does not have the ability to induce antibodydependent and complement-dependent cellular cytotoxicity, which reduces the risk of developing infusion phenomena.
When studying the principle of action of APM, it was shown that blockade of IFNAR1-mediated signaling is associated with a wide range of molecular and cellular effects: suppression of the expression of IFN-induced genes; phosphorylation of STAT I (signal transducer and activator of transcription); synthesis of type I IFN and inflammatory cytokines; overexpression of costimulatory molecules on the pDC membrane; pDC and B cell differentiation [66]. There was a decrease in TRAIL (TNF-related apoptosis-inducing ligand) load, which was previously found to increase in SLE [67], as well as IP-10 (interferon gamma-induced protein 10) and progranulin (regulate the recruitment of immune cells in the zone), associated with activity SKV [68, 69].
Other consequences of AFM include normalization of the B cell cytokine chain, such as BAFF (B cell activating factor belonging to the TNF family), the synthesis of which is altered by IFN type I [70]. When treating AFM in patients with SLE, rapid normalization of the level of lymphocytes, neultrophy, monocytes and platelets, circulating CD4+ and CD8+ T cells and memory B cells is observed. Notably, the anti-BAFF monoclonal antibody (belimumab) used in SLE [65] causes a decrease in naïve and switched B cells but does not affect B cell memory [71]. There was a tendency towards normalization of the level of antibodies to double-stranded DNA (anti-dsDNA) and complement components (C3, C4, CH50).
CONCLUSIONS
In addition, in the vast majority of patients treated for SLE, AFM was associated with suppression of basal expression of interferon signatures. Thus, after 24 weeks, the average level of suppression index of 21 genes characteristic of the signature was 89.7% at a dose of APM 300 mg after 4 weeks and 91.7% with a dose of AFM 1000 mg in women for 4 weeks. However, suppression of type I IFN signatures in patients with initial overexpression of these genes was detected after 12 weeks and persisted for 52 weeks [72] In addition to standard individual therapy, AFM reduces the need for corticosteroids and reduces the activity of lupus, especially skin and musculoskeletal diseases, and has an acceptable safety profile [73].
The data obtained to date indicate the expediency of studying the level of expression of IFN-induced genes, for example, using PCR test systems, both in the case of SLE and in some other systemic inflammatory diseases. Such a study should improve the stratification of patients with SLE, prompt replacement of other therapeutic approaches with targeted blockade of IFN type I for patients with a high IFN signature, and expand the range of interventions for the use of such therapy.