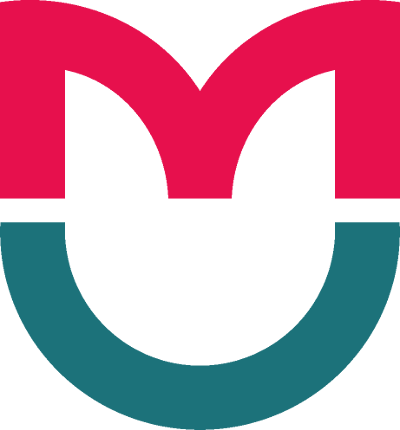
This article is an open access article distributed under the terms and conditions of the Creative Commons Attribution license (CC BY).
ORIGINAL RESEARCH
Strains of Mycobacterium tuberculosis with mutations in gyrA differ in their level of competitive fitness
1 Central Tuberculosis Research Institute, Moscow, Russia
2 Yevdokimov Moscow State University of Medicine and Dentistry, Moscow, Russia
Correspondence should be addressed: Sophia Nikolayevna Andreevskaya
Yauzskaya Alley, 2 p. 1A, Moscow, 107564, Russia; ur.liam@aifosdna
Funding: the study was conducted under the State assignment of FGBNU ‘CNIIT’ No. NIOCTR 122041100246-3 ‘Interspecies and intraspecies polymorphism of mycobacteria in patients with tuberculosis and mycobacteriosis in the presence of specific therapy’.
Author contribution: Chernousova LN, Ergeshov A — development of the research design; Ustinova VV, Kiseleva EA — conducting the experiment; Smirnova TG — conducting the experiment, analyzing the obtained data; Larionova EE — analyzing the obtained data; Sevastyanova EV — review of publications on the topic of the research; Andreevskaya SN — analyzing the obtained data, writing the manuscript.
Mycobacteria of a tuberculosis complex form a genetically homogeneous group of bacteria with a low mutagenesis rate compared to other bacteria [1]. Although the frequency of mutations in Mycobacterium tuberculosis (MTB) is low, it must be considered that tuberculosis disease lasts from several months to several years, and the size of the bacterial population in the host during this time can reach more than 1 billion CFU. Over the course of the disease, there is an increased likelihood of spontaneous mutations, including mutations in genes associated with drug resistance, which may result in the emergence of clones with new genotypes [2, 3].
The coexistence of drug-sensitive and drug-resistant MTB pools in the same organism is called bacterial heteroresistance [4, 5]. Heteroresistance can occur not only in the case of microevolution of a single clone, as described above (monoclonal heteroresistance), but also as a result of mixed infection, when a person is infected with several MTB strains with different drug resistance (polyclonal heteroresistance) [6–8]. Monoclonal heteroresistance is considered one of the major steps in the development of drug resistance in bacterial isolates [5, 9]. Several studies have linked the presence of heteroresistance to poor outcome of TB treatment [10, 11].
Multidrug-resistant tuberculosis (MDR TB) is currently on the rise worldwide, when the pathogen is resistant to the two main first-line drugs, Rifampicin and Isoniazid, at the same time [12]. Fluoroquinolones are the most effective drugs for MDR TB therapy [13–15]. According to different authors, the frequency of MTB heteroresistance to fluoroquinolones varies from 1 to 35% depending on the region studied [16–20].
The already mentioned formation of drug resistance through heteroresistance, failure of TB therapy in the presence of pathogen heteroresistance, and fluoroquinolones becoming the most effective drugs for MDR TB — all this provides relevance to the studies aimed at understanding the mechanisms of MTB heteroresistance to fluoroquinolones, also helping to understand the evolutionary perspectives of MTBs with different genetic determinants of resistance to drugs of this group.
The goal of this research was to run an in vitro investigation of growth characteristics of M. tuberculosis strains which have different genetic determinants of resistance to fluoroquinolones, in the setting of competition for nutrients.
METHODS
The subject of the study
The study was conducted on five clinical strains of MTBs that were maximally similar in phenotypic resistance, had an identical MDR genotype (rpoB_S531L + katG_S315T) and belonged to the spoligygous SIT1 of Beijing sublineage, but differed in genetic determinants of resistance to fluoroquinolones (four strains with different mutations in the gyrA gene and one strain with wild-type gyrA; tab. 1).
Culturing MTB strains
MTB strains were cultured in pairs and individually on Middlebrook 7H9 liquid nutrient medium and under multistress conditions (50% Middlebrook 7H9 medium, 2 mM KNO2, 0.02% H2O2). The time of the experiment was 21 days.
Determining the number of MTB cells in a sample
To determine the number of cells of each strain in the sample, we followed an approach using multiplex PCR using the Amplitub-FQ-PV kit (Syntol, Russia), which detects key mutations associated with fluoroquinolone resistance. For this purpose, serial dilutions of each of the MTB strains from 102 to 108 CFU/ml were prepared and calibration curves were plotted, showing the dependence of the threshold cycle of amplification — respective to the channel targeted by the mutation — on the concentration of MTB cells in the sample. According to the calibration curves, the concentration of each of the co-cultured mutants was determined by the value of the threshold cycle. All studies were performed in triplicate.
Competitive fitness
Competitive fitness (W) of MTB strains, reflecting the total culture growth of each strain during the experiment in paired cultivation, was determined according to the formula [21]:
where W — Competitive fitness level;
S1(i) — initial concentration of strain 1;
S1(f) — final concentration of strain 1;
S2(i) — initial concentration of strain 2; S2(f) — final concentration of strain 2.
When W > 1, the competitive fitness of strain 1 is higher than that of strain 2;
W < 1, the competitive fitness of strain 1 is lower than that of strain 2;
W = 1, strains 1 and 2 have similar competitive fitness.
To compare the growth of MTB strains cultured in pairs with individual growth on the medium, we determined the specific growth rate of the culture (µ), i.e. the accumulation of bacterial mass per unit time during the exponential phase of growth, according to the formula [22]:
where g — generation time, defined as:
where t1 and t2 — parameter estimation time during the exponential growth phase; n — number of generations, defined as:
where N1 and N2 — number of CFU at time points t1 and t2, respectively.
RESULTS
The level of competitive fitness was determined for 10 pairs of MTB strains with different determinants of resistance to fluoroquinolones (tab. 2). It was shown that under competition for nutrients during growth under optimal conditions, MTB strains with wild-type gyrA accumulated biomass more intensively compared to strains 90V, 94A and 94G. Strain 94Y, when cultured under optimal conditions, had the highest competitive fitness of all strains examined, including strain WT. MTB strains 94A and 94G had the lowest competitive fitness under optimal culture conditions.
Under multistress conditions on depleted Middlebrook 7H9 medium in the presence of active oxygen and nitrogen, the ability of the studied MTB strains to compete for nutrients differed from optimal culture conditions. Under multistress, the wild-type gyrA strain was more competitive compared to MTB strains with mutations in gyrA. The competitive fitness of strain 94Y decreased dramatically under multistress conditions, while in contrast, strain 94A improved compared to optimal culturing conditions. The competitive fitness of strain 94G under multistress conditions, as well as under optimal conditions, was the lowest among all strains studied.
The specific growth rate of MTB culture with different genetic determinants of resistance to fluoroquinolones also depended on the culture conditions (tab. 1). When individually cultured under optimal conditions, the wild-type gyrA strain and the 94Y / 90V mutants had similar specific growth rates. The specific growth rate of the 94G and 94A mutants was lower.
When paired culturing was performed under optimal conditions, the specific growth rate of MTB strains was generally the same as that of individual culturing. A lower value of specific growth rate in paired culturing, compared to individual culturing, was observed only for strain WT when cocultured with strain 94A and for strain 94G when co-cultured with strain WT and with strain 94A.
Under multistress conditions of individual culturing, the specific growth rate of MTB strains WT and 90V was at the same level as under optimal conditions, while that of strain 94A increased. The specific growth rate of strains 94G and 94Y was lower under multistress conditions when individually cultured than under optimal conditions.
When strains were co-cultured under multistress conditions, the specific growth rate of strains WT, 90V, 94A, and 94Y did not differ significantly from that of strains cultured under optimal conditions, or, in some combinations, was higher. The specific growth rate of strain 94G when cultured with other strains either did not change compared to the optimal conditions, remaining at the same low level, or decreased even more compared to the optimal conditions.
The specific growth rate of strains when cultured individually and when cultured under multistress conditions tended to differ. Strain 94G when co-cultured with strains WT and 94A and strain 94A when co-cultured with strain WT had a specific rate more than 10% lower than that of individual cultivation. But more often the specific rate was higher when strains were co-cultured under multistress conditions than when they were cultured individually (strain WT co-cultured with 94A; strain 90V co-cultured with WT, 94G and 94A, strain 94A co-cultured with 94Y and 90V; strain 94G co-cultured with 90V and 94Y, strain 94Y co-cultured with 90V and WT).
It was also found that MTB strains with mutations in gyrA differed in their ability to replicate when co-cultured, and this parameter for most strains varied depending on the culture conditions.
Thus, under optimal conditions, MTB strain 94Y multiplied more intensively than strains 94A and 94G, while under multistress conditions, the best growth rates in pairs were demonstrated by strain 94A, and the fission rate of strains 94Y and 94G in paired cultivation was identical. Similarly, cultivation conditions affected the level of competitive fitness in pairs 94G + 90V, 94G + 94A and 94A + 90V. The only exception was the pair of strains 94Y + 90V, in which both under optimal and multistress conditions the strain 94Y had a higher culture growth rate.
DISCUSSION
The study examined the culture growth characteristics of five MTB strains differing in genetic determinants of resistance to fluoroquinolones, in individual and paired cultivation. The gyrAmutant MTB variants studied are represented in the population as follows: strains with a mutation in gyrA D94G are the most common (40%), followed by strains with mutation A90V (20%), then strains with mutation D94A (15%) and D94Y (5%). [20]. There are other gyrA-mutant variants of MTB, but they are very rarely exuded by patients, which prevented us from including these strains in the study.
Heteroresistance of the MTB population in the host organism can be considered as a special case of intraspecies competition, as there is competition for a limited resource between representatives of the same species with insignificant differences (in our case, the different structure of the alpha subunits of DNA gyrase encoded by the gyrA gene). The result of such competition may be the disappearance of the least adapted MTB pool and the proliferation of the most adapted pool in the population. Since the competitiveness of mutant MTB variants was to be studied, one of the parameters we evaluated was the competitive fitness of MTB strains when cocultured. MTB competitive fitness is traditionally determined by culturing a resistant strain together with a susceptible strain, followed by reinoculation of the strain mixture on medium with an antituberculosis drug, thereby determining the number of CFU of the resistant strain in the mixture. [23]. However, this approach does not allow us to compare the competitive fitness of two resistant strains, so for the first time we used a multiplex PCR method to determine competitive fitness, which allowed us to quantify the number of CFU of each mutant variant in a sample. Since the study was performed in dynamics rather than by endpoint, the approach we used also allowed us to estimate the specific growth rate of each mutant when co-cultured.
In addition, we studied the growth of co-cultured strains not only under optimal conditions but also in a multistress model, being able to create in vitro — to some extent — the conditions that the pathogen encounters in the macroorganism: lack of nutrients and presence of active nitrogen and oxygen as bactericidal agents produced by macrophages [24].
We have demonstrated that the competitive fitness of MTB strains with mutations in gyrA is lower than that of strains with wild-type gyrA. The only exception when cultured under optimal conditions was strain 94Y with a rare mutation, however under conditions as close as possible to in vivo conditions in the multistress model, its fission rate decreased significantly.
The low fission rate of gyrA-mutant MTB strains was generally predictable, as the gene encodes the alpha-subunit of DNA gyrase [25]. Changes in the structure of this enzyme due to mutations in gyrA can inhibit DNA replication and transcription in the MTB cell and lead to a decrease in the growth rate of the pathogen.
The frequency of gyrA-mutant MTB variants occurrence in a population associates with the level of competitive fitness in a multistress culturing model as follows: the strain with the frequent mutation gyrA_A90V had high competitive fitness values, while the strain with the rare mutation gyrA_D94Y had low values. The fitness of the strain with the gyrA_D94A mutation in the multistress model was comparable to that of strain 90V, but was less frequent in the population, possibly because the gyrA_D94A mutation is not strictly associated with resistance to fluoroquinolones and, therefore, the pool of MTBs carrying this mutation could be eliminated during chemotherapy [20, 26, 27].
Surprisingly, the strain with the most common gyrA_D94G mutation had the lowest competitive fitness and lowest specific growth rate of all strains examined. However, a reduced rate of fission in vivo is not always a disadvantage, especially under selection pressure due to chemotherapy. Most TB drugs are effective against MTBs demonstrating active fission, while pools of slow fission pose a major challenge to the efficacy of chemotherapy [28, 29].
Consequently, it can be assumed that the high prevalence of fluoroquinolone-resistant MTBs with the gyrA_D94G mutation is related to the low fission rate of such strains, which allows them to avoid exposure to anti-TB drugs.
In summarising the results of this study, it appears that the evolutionary success of the most common gyrA-mutant variant of MTB lies in its tolerance to anti-TB drugs due to slow growth. MTBs with the gyrA_A90V mutation, which are twice as rare, have good growth properties and have been favoured by this feature. Strains with the gyrA_D94A mutation are even rarer because, having a high level of competitive fitness, they are not always resistant to fluoroquinolones. MTBs with the gyrA_D94Y mutation have the lowest fitness under multistress conditions and are rarely found in the population.
In conclusion, it should be noted that the article presents data from a pilot study on a limited group of strains; therefore, the findings can be regarded as preliminary and should not be generalised at this stage to the entire population of MTBs resistant to fluoroquinolones. A full-scale study on a large sample of strains is planned to confirm the findings.
CONCLUSIONS
This study found that MTB strains with mutations in gyrA differ in their ability to adapt to nutrient competition in vitro. Strain cultivation conditions influence the level of competitive fitness of MTB strains with different genetic determinants of resistance to fluoroquinolones. Most MTB strains demonstrate a direct association between the level of competitive fitness under multistress conditions and the frequency of occurrence in the population. Mutations in gyrA reduce the rate of MTB growth; maybe it helps the pathogen survive chemotherapy.