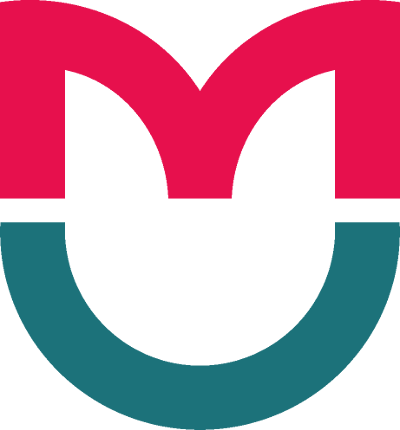
This article is an open access article distributed under the terms and conditions of the Creative Commons Attribution license (CC BY).
ORIGINAL RESEARCH
Prospects of gene therapy with hematopoietic stem cells
1 Moscow Institute of Physics and Technology (National Research University), Moscow, Russia
2 Kulakov National Medical Research Center for Obstetrics, Gynecology and Perinatology, Moscow, Russia
Correspondence should be addressed: Konstantin V. Popov
Akademika Oparina, 4, Moscow, 117997, Russia; ur.liam@tsnok_vopop
Funding: the paper was executed within the framework of the State Assignment of the Ministry of Health of the Russian Federation No. 123020800103-6.
Author contribution: Valieva YM — literature review, data acquisition, manuscript writing; Popov KV — manuscript concept, editing.
According to the definition, the therapeutic effect of a gene therapy medicinal product is determined by the sequence of recombinant nucleic acid included in its composition or by the product of the expression of such a sequence [1]. Depending on the molecular mechanism underlying the disease, therapy may aim to correct genetic defects or give cells new properties. The strategy for dealing with a damaged gene function may include adding a working copy, suppressing expression or correcting the sequence. Adding a working copy of the gene is used to treat autosomal recessive disorders. Suppressing the expression of the damaged gene is used for autosomal dominant diseases [2]. With the development of genome editing techniques, correcting the sequence of a damaged gene is being considered a priority approach for the development of treatment for both autosomal recessive and autosomal dominant inherited diseases. CAR-T therapy is the most widely used gene therapy drug in clinical practice, is based on giving cells with new properties. In this case, a chimeric receptor is used to enable them to recognize and destroy cancer cells [3].
Current gene therapy methods
Regardless of the mechanism of action of the nucleic acid contained in the gene therapy drug, a key factor in the development of the manufacturing technology for the drug is the choice between in vivo and ex vivo delivery of the therapeutic construct to the target cells. In the in vivo application, the drug containing the vector is administered directly into the patient's body. For ex vivo application, specific cells are isolated from the patient's body, genetically modified, and then returned to the patient.
Currently, the use of viral vectors is the most studied method for delivering genes into cells. Viruses are used for targeted delivery of necessary genetic material to cells both in vivo and ex vivo. Leaders among modified gene carriers are adeno-associated viral (AAV) vectors and lentiviral vectors. AAV vectors are predominantly used in vivo, while lentiviral vectors are utilized for ex vivo therapy [4, 5].
RNA methods in gene therapy represent a rapidly evolving field with significant potential for treating various diseases. These methods include therapy using antisense oligonucleotides, RNA interference and messenger RNA (mRNA). The development of non-viral RNA delivery systems, particularly those based on encapsulation in lipid nanoparticles, is expected to allow for the avoidance of viral delivery systems in the future. This advancement will help to mitigate risks associated with the immune system’s reactions to viral proteins and the uncontrolled integration of transgenes into the patient's genome [6].
The development of a new method of genome editing, CRISPR/Cas9, has become a groundbreaking technology. CRISPR/Cas9 can be used to correct genetic mutations by precisely editing the defective gene. This approach is particularly relevant for monogenic diseases, where a specific gene mutation is responsible for the condition [7].
Gene therapy in vivo has a competitive advantage in most cases due to the ability to create ready-made drug products tailored to the main cohort of patients. This leads to ease of scaling, a reduction in cost per unit of production and readiness for on-demand use.
Gene therapy ex vivo is primarily used in the treatment of oncological diseases (in this case, immune cells are modified) and in the treatment of hereditary diseases using genetically modified hematopoietic stem cells (HSCs). HSCs are optimal target cells for therapeutic genome editing due to their ability to self-renew and differentiate. Thus, a prolonged expression level of the therapeutic construct is ensured, along with the delivery of its expression products to differentiated cells throughout most of the organism. Moreover, HSCs do not carry the risk of immunogenicity associated with the direct use of high doses of viral vectors in vivo [8].
Hematopoietic stem cell gene therapy
The methodology of ex vivo gene therapy using hematopoietic stem cells (HSC GT) is a multi-step process. First, HSCs are collected from the patient, then they are cultured, subjected to genetic modification, and the modified cells are reintroduced into the patient. The injected cells populate the HSC niche in the bone marrow [9]. HSC GT ex vivo appears to be a promising approach for treating monogenic genetic disorders, including primary immunodeficiencies, hemoglobinopathies and lysosomal storage diseases.
The most successful example of HSC GT is the gene therapy product registered in 2020 for the treatment of metachromatic leukodystrophy (MLD) — Libmeldy. The drug consists of autologous HSCs that have been transduced with a lentiviral vector carrying a functional copy of the ARSA gene. The treatment results showed a significant therapeutic effect and a long duration of action for the drug [10]. The use of lentivirus-based vectors ensures stable integration of genetic constructs into the genome, resulting in high and prolonged gene expression. However, utilizing viral vectors for modifying HSCs poses a risk of insertional mutagenesis. Treatment of X-linked severe combined immunodeficiency (X-SCID) with a similar HSC GT drug was found to be associated with an increased risk of T-cell acute lymphoblastic leukemia. Despite efforts to modify the design of lentiviral vectors to reduce the risk of cancer development due to transgene integration, similar complications were observed when using the therapy for treating adrenoleukodystrophy [11]. Increasing the safety of modified HSCs can be achieved by using non-viral delivery systems based on transposons and CRISPR/Cas genome editing systems.
The advantage of ex vivo HSCs gene therapy compared to in vivo therapy using viral vectors lies in the absence of the need to use high doses of the vector, thereby reducing the patient's risk of developing immune reactions. In particular, when using AAV vectors, a significant portion of particles remains in the liver due to the first-pass effect. In other words, if the target organ differs from the liver, AAV vectors do not reach it at the required therapeutic dose, and using higher doses leads to hepatotoxic and immunogenic effects [12]. Since autologous cells of the patient are used in HSC GT, the immunogenicity of these products is minimal. Transplantation of HSCs with stable integration of gene therapy constructs provides a longlasting, theoretically lifelong therapeutic effect. In the case of hematopoietic diseases, such as Wiskott-Aldrich syndrome and other primary immunodeficiencies, HSCs themselves are the target cells. HSCs are capable of crossing the blood-brain barrier, differentiating into macrophages to occupy the microglial niche, and expressing necessary enzymes in brain tissues [13]. This explains the effect of allogeneic hematopoietic stem cell transplantation from a healthy donor for the treatment of a group of hereditary diseases caused by damage to brain nerve cells, including mucopolysaccharidoses, adrenoleukodystrophy, and MLD. The same effect is achieved when using autologous HSCs with corrected gene defects.
The improvement of ex vivo cultivation methods for HSCs allows for the production of significantly more HSCs for modification than what is available from directly using cells collected from the patient. High doses of HSCs used for transplantation raise hopes for the introduction of modified HSCs transplantation protocols that do not require genotoxic conditioning regimens. Currently, achieving a clinical effect from HSCs transplantation requires prior myeloablative conditioning through chemotherapy aimed at suppressing the patient's bone marrow. Such conditioning carries both short-term risks associated with the development of infectious diseases and long-term risks of growth impairment, intellectual development issues, loss of fertility, and the development of other diseases [14]. Currently, protocols are being developed for the formation of HSCs niches using antibody conjugates specific to HSCs combined with toxins.
Today, the most expensive drug in the world is Lenmeldy, an HSC-based medicine for MLD [15]. The use of patient-derived cells for the production of the drug means that there is no possibility of reducing the cost per dose by increasing the volume of the produced batch. In addition, ex vivo application also requires complex logistics and carries additional risks related to ensuring the quality and safety of the donor material. The possibility of reducing the production costs of these drugs is linked to optimizing the logistics of delivering donor material and the finished product, as well as automating the manufacturing process. A significant reduction in the cost of drugs can be expected from replacing the currently used viral vectors with non-viral systems for delivering gene therapy constructs. This approach will help reduce the time and costs associated with each unit of the drug. The creation of scientific and technological complexes for the development of personalized drugs based on HSC GT will help automate, accelerate, and scale the production process.
CONCLUSION
The development of ex vivo HSC GT is currently relevant and promising. The development of such therapy will provide opportunities for etiological treatment of many incurable genetic diseases. Further exploration of this direction in gene therapy, improvement of existing methods, and the development of new ones will expand the range of diseases for which treatment with HSC GT is effective from both clinical and economic perspectives.