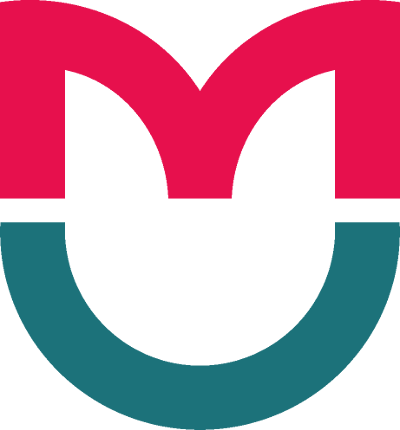
ORIGINAL RESEARCH
Preliminary results of a controlled study of BCI-exoskeleton technology efficacy in patients with poststroke arm paresis
1 Institute of Higher Nervous Activity and Neurophysiology, RAS, Moscow, Russia
2 Pirogov Russian National Research Medical University, Moscow, Russia
3 Research Center of Neurology, Moscow, Russia
4 Vladimirsky Moscow Regional Research Clinical Institute, Moscow, Russia
5 Municipal Clinical Hospital no. 31, Moscow, Russia
Correspondence should be addressed: Olesya Mokienko
Volokolamskoye shosse, d. 80, kab. 133, Moscow, Russia, 125367; ur.xednay@dm.aysel
Funding: the study was supported by the Ministry of Education and Science of the Russian Federation (Grant Agreement no. 14.607.21.0128 dated October 27, 2015), Russian Foundation Basic Research grants no. 16-04-01506а and 16-04-00962а.
Assessment of approaches to upper limb function restoration in poststroke patients with hemiparesis is a highpriority task in neurorehabilitation [1, 2]. However, none of the existing methods of motor rehabilitation has been assigned the highest level of evidence and high grades of recommendation strength for arm function restoration. The moderate level of evidence for arm function restoration in poststroke patients is demonstrated by virtual reality technology, robotic tools (due to abundant repetitive task practice) and mental training, including motor imagery [1, 2]. It is important to note that in contrast to motor imagery methods based on active motor paradigms, such as robotic technologies and constraint-induced movement therapy, can be applied to patients with mild or moderate paresis. In case of plegia or severe paresis, robotic therapy often plays a role of passive mechanotherapy.
The impact of motor imagery on motor nervous system activity and neuroplasticity has been demonstrated in multiple neurophysiological studies. It has been shown that during motor imagery, primary motor cortex and brain structures that participate in voluntary movement planning and control are activated [3, 4, 5, 6]. In the study that utilized navigated transcranial magnetic stimulation of the brain, the subjects who had been trained in motor imagery exhibited a decreased motor threshold and larger evoked motor responses of the muscles involved in fist clenching [4].
Thus, motor imagery remains the only active paradigm for modulating neroplasticity in motor areas of the brain in patients with plegia and severe paresis [3, 4, 7, 8]. Motor imagery can also be used for the rehabilitation of patients with mild motor dysfunctions as a training tool for more effective motor planning and accurate motor performance [9]. Motor imagery can be controlled by kinesthetic feedback provided by braincomputer-exoskeleton interfaces. Brain-computer interfaces (BCIs) allow for translating brain activity signals into commands for the external device [10, 11]. With motor imagery, such signals are represented by sensorimotor rhythm modulation [12]. If a limb exoskeleton is used as an external device, the BCI operator receives kinesthetic feedback (the operator needs to imagine the movement that the exoskeleton is able to perform).
A number of controlled trials have been carried out to study the efficacy of non-invasive BCIs with external assistive devices that implement kinesthetic feedback. Those studies enrolled up to 32 patients with poststroke arm paresis. Haptic Knob [13] and MIT-Manus [14] robots and orthoses [15], which are not exoskeletons by design, were used as external devices.
Clinical trials of the efficacy of a BCI-based system where kinesthetic feedback is implemented by a hand exoskeleton have been conducted in Russia [16, 17, 18]. Biryukova et al. [19] studied one clinical case. However, none of those works compared the obtained results with the controls. Besides, clinical effectiveness of training in using a BCI technology to control the external assistive device has not been studied in patients at different rehabilitation stages and with different paresis severity; the effect of repetitive training using a BCIexternal assistive device technology has not been investigated.
In this work we present preliminary results of a multicenter blind randomized controlled study of the efficacy of the hand exoskeleton controlled by non-invasive braincomputer interface for the rehabilitation of patients with poststroke paresis. The study will be open for participant recruitment until the number of participants reaches 120.
METHODS
The study was approved by the Ethics Committee of the Research Center of Neurology (protocol no. 12/14 dated December 10, 2014). All patients gave written informed consent. The protocol of iMove study is listed in the international registry of clinical trials of the U.S. National Institutes of Health (ClinicalTrials.gov; study indentifier is NCT02325947).
This blind randomized controlled study has been carried out at three clinical centers since December, 2014. Among site selection criteria were the presence of a neurorehabilitation unit or a motor rehabilitation service and a pool of patients with a history of stroke at various time points in the past or hemiparesis of various degrees.
The study included male and female patients aged 18–80 years with a prior stroke (1 month to 2 years before screening); with a poststroke hand paresis (from mild to plegia on Medical Research Council Weakness Scale sums score, MRC-SS [20]); with a supratentorial focal ischemic or hemorrhagic stroke confirmed by MRI or CT scan; all patients gave written informed consent. Study participants were either admitted to the clinical centers or received outpatient therapy.
The following exclusion criteria were applied: lefthandedness according to Edinburgh Handedness Inventory [21]; severe cognitive impairment (Montreal Cognitive Assessment Score >10) [22]; sensory aphasia; severe motor aphasia; severe vision impairment that would not allow the patient to follow visual instructions on the computer screen; arm muscle contracture (Modified Ashworth Scale score of 4) [23].
Withdrawal criteria were as follows: patient’s refusal to participate in the study; development of acute disease or decompensation of chronic disease that could possibly affect the study outcome, including recurrent cerebrovascular events, acute myocardial infarction, decompensated diabetes, etc.; therapy with systemic muscle relaxants that started after the participant had been enrolled (or medication dosage change); injections of botulinum toxins in paretic arm muscles after the patient had been enrolled.
Patients who gave informed consent to participate in the study and met inclusion/exclusion criteria were screened; their data were submitted to the automated system of information support for clinical trials (ImagerySoft, Russia); each participant was given an identification number. Then participants were randomly allocated to the experimental or control group (3 : 1).
Patients from the experimental group were trained to use the BCI-exoskeleton technology; patients from the control group were trained to use the BCI-imitating system. Each group attended up to 12 training sessions (each 40 min long) every day except weekends (the acceptable idle interval was up to 3 days). Patients from both groups also underwent standard rehabilitation procedures, such as therapeutic exercises with the instructor and massage.
In this study we used a BCI based on EEG pattern analysis and recognition of synchronization/desynchronization of sensorimotor rhythms during arm movement imagery. EEG signals were band-pass filtered between 5–30 Hz. We used the EEG pattern classification based on Bayesian method [24, 25]. To assess classification accuracy, we used Cohen's kappa coefficient (κ = 1 represented perfect recognition, κ = 0 represented due-to-chance recognition [26] ) and the percentage of right responses suggested by the classifier (>33 % value represented more than chance recognition, because patients performed three mental tasks). The components of the BCI-exoskeleton system are presented in fig. 1.
During the session, the patient was wearing an electrode cap for EEG recording. Electrode gel was applied underneath each electrode. The exoskeleton was fixed to the paretic arm. The exoskeleton used in this study is a polymer carcass for the hand and fingers with robotic pneumatic drive, intended for finger extension that does not exceed the physiological norm. During the training session, the patient was sitting in front of the computer screen; his arms were on the armrest or on the desk in a comfortable position.
In the middle of the dark screen there was a circle for gaze fixation with 3 arrows around it; the arrows changed colors to indicate a new instruction. The patient followed one of three instructions: to relax and, to imagine a slow extension of the left hand fingers or the right hand fingers kinesthetically. The instructions to imagine the extension of the right or left hand fingers (right or left arrow changed its color respectively) were presented on the screen in random order for 10 min. Following the instruction to relax, the patient had to sit still and watch the center of the screen.
Results of mental task recognition were presented to the patient via visual and kinesthetic feedback. If the classifier successfully recognized the task the patient had been instructed with, the circle in the middle of the screen turned green and the exoskeleton extended fingers. When other tasks were recognized, the circle did not change its color and the exoskeleton did not perform any action.
One training procedure consisted of up to three sessions described above; each session lasted for 10 s. The patient rested for 5 s between the sessions.
With the controls, the same components of the BCI system were used and the same conditions were applied. The patients in the control group also followed the instruction to relax and watch the arrow color. The color changed at random, each change lasted for 10 s, and the exoskeleton opened the fingers of the paretic hand when the corresponding arrow appeared on the screen.
Thus, the patient in the control group did not imagine the movement and did not try to control the exoskeleton, but received passive mechanotherapy for the paretic hand. EEG signals were recorded for monitoring.
The researcher who performed clinical assessment of the patients did not know what group the patient was included into. This information was only available to the researchers who conducted rehabilitation sessions using BCI-exoskeleton system or its dummy.
Before and after the training course, the patients underwent a procedure for arm movement and arm force assessment based on Fugl–Meyer Assessment scale (FM) and Action Research Arm Test (ARAT) [27, 28]. Besides, dynamics across different scale sections were analyzed. The degree of spasticity was assessed using MAS scale.
We also estimated the percentage of patients with improvements by 5 points or more on ARAT scale and by 7 points or more in the motor function of upper extremities on FM scale (A–H sections).
Statistical analysis was done using Mann–Whitney test (for independent samples). Wilcoxon test (for dependent samples), Spearman correlation coefficient, RM-ANOVA analysis of variance, and a maximum likelihood χ² test on the PC with installed Statsoft Statistica 6.0 software.
The data are presented as median and quartiles (25 %; 75 %). Differences were considered statistically significant with р < 0.05.
RESULTS
232 patients were screened for eligibility. Out of 58 patients who met the inclusion criteria 11 patients refused to participate after the first or second training procedure. Thus, the study included 47 patients (33 male and 14 female) with a mean age of 56 years (48 and 64 years respectively), median time elapsed after stroke was 8 months (4 and 13 months respectively). There were 35 patients with ischemic stroke and 12 patients with hemorrhagic stroke. All enrolled patients were righthanded and Caucasian. The experimental group consisted of 36 patients; they attended BCI-exoskeleton training sessions. The control group included 11 patients who had training sessions with a dummy. The groups were comparable in terms of age, time elapsed after stroke, and the degree of neurological deficit. Patients’ demographics and the initial data are presented in tab. 1. No statistical differences were found between the groups with respect to age, time elapsed after stroke, lesion localization and lateralization and the degree of neurological deficit. No statistical differences were found between the patients from three clinical centers with respect to time elapsed after stroke, type, localization and severity of neurological deficit.
Mean number of training sessions was 9.5 (8.0; 10.0) in the experimental group and 10.0 (6.0; 10.0) in the control group, with p > 0.05.
In both groups, improvement of arm motor activity assessed by ARAT and FM scales (arm function sections: A–D, H, I) was observed. The following improvements on ARAT scale were observed in the experimental group only: grasp scores increased from 0.5 (0.0; 13.0) to 3.0 (0.0; 15.5) points, with р = 0.003; pinch scores increased from 0.5 (0.0; 7.5) to 1.0 (0.0; 12.0) points, with р = 0.005; gross arm movement scores increased from 2.0 (0.0; 4.5) to 3.0 (1.0; 6.5) points, with р < 0.001 (tab. 2). No statistically significant differences were found between the groups in motor function improvement using RM-ANOVA analysis.
In the experimental group, a clinically significant improvement in the arm motor function on ARAT scale (by 5 points or more) and on FM scale (by 7 points or more, sections A-D) was found in 33.3 % patient and 30.5 % patients, respectively. A clinically significant improvement of arm motor function on both scales was found in 16.7 % patients of the experimental group. The observed improvement was associated with the restoration of wrist motor function. In the control group, the percentage of patients with clinically significant improvement of arm motor function was lower: 9.1 and 18.2 % on ARAT and FM scales, respectively (tab. 2).
In both group, restoration of arm function did not depend on the time elapsed after stroke and patient’s age (on both ARAT and FM scales and subscales). In each group, a moderate or medium correlation between the restoration degree of arm function (wrist in particular) assessed by ARAT scale and the initial severity of neurological deficit (r = 0.4, p < 0.05) was found; however, in the experimental group, statistically significant improvement of wrist function was observed in the subgroup of patients with initially severe paresis, as well as in the subgroup of patients with mild or moderate paresis (tab. 3).
Three patients of the experimental group from the second study site took a second BCI-exoskeleton training course during another planned hospitalization. The time interval between the courses was 6 to 9 months. Every course consisted of 8–10 training sessions. As shown in fig. 2, by the time of the second hospitalization, arm motor function assessed by ARAT scale had not deteriorated in any patient. The score of patient 1 on FM scale (C–D) was lower at the time of the second hospitalization, but still considerably higher than the initial score. During the second rehabilitation therapy course with BCI-exoskeleton training sessions included, all three patients displayed improvement of arm motor function parameters.
None of the patients displayed deterioration of arm functions on ARAT or FM scale during the study.
During the training sessions, 3 patients had mild headache, namely, 2 patients from the experimental group (one of them observed headache during two training sessions out of ten, the other had headache over the course of all ten sessions) and 1 patient from the control group (during 3 sessions out of 10).
The majority of patients reported attention fatigue 20 to 30 min after the training session. Fatigue was more conspicuous if a patient had been insomniac the night before the training (2 patients in the experimental group), was prone to depression (2 patients in the experimental group), had other tiring therapeutic procedures before the session (1 patient in the experimental group), or was initially weak. The majority of patients thought that fatigue was the evidence of training effectiveness and felt good about it.
If there were complaints about headache or fatigue, the training session was discontinued for that day. For one patient, the time between the sessions within one training course was extended to 2–3 min (in agreement with his doctor and following the patient’s wish). Due to fatigue and bad general condition, the time between the sessions was increased up to 2–3 days for one patient from the experimental group.
One patient from the experimental group had an episode of high blood pressure (200/100 mmHg) after the third training session during the second therapy course, but was able to respond to medication.
On the whole, none of the patients withdrew from the study on account of adverse effects.
DISCUSSION
Preliminary results of iMove multicenter blind controlled study conducted in Russia have shown that a 2–3 week rehabilitation therapy using a BCI-exoskeleton technology increases the number of patients with clinically significant improvement in arm motor function. This improvement is associated with the recovery of hand function, the motor imagery of which was practiced by the patients. It was also shown that only in the BCI-exoskeleton group, grasp and pinch movements were improved. It is important to note that to grasp a big object (for example, a special object for ARAT-based assessment), the intact ability for hand opening movement is necessary. During BCI-exoskeleton training sessions, the patients imagined hand opening and feedback was provided kinesthetically by the exoskeleton that implemented the movement. No statistically significant difference in the degree of motor function restoration was found between the control and the experimental groups, which can be explained by the insufficient training duration and the length of observation period [14].
The results of our study are consistent with the data provided by other controlled studies in a given area of research. In Ramos–Murguialday study, 16 patients with poststroke hemiparesis were trained to use a BCI-orthesis system and 16 other patients were included in the control group. In the control group, the orthesis was not connected to the BCI and opened at random. Both groups had training sessions for 4 weeks, except weekends (in average, the patients had about 18 training sessions). As a result, the BCI group showed improvement in motor function on FM scale and scored 3.41 points more than the control group (p = 0.018) [15].
With 26 patients enrolled in the study, Ang investigated the efficacy of treatment in the group that received BCI-Manus training sessions compared to the group that received only MIT-Manus robotic therapy. In the second group, training intensity was considerably higher than in the first group (1040 movements against 136 during one session). After the 4-week course, the efficacy of treatment was comparable in both groups; however, 12 weeks after the observation had started, further motor function improvements were observed in 63.6 % patients from the BCI-Manus group and in 35.7 % controls [14].
In another study that enrolled 21 patients and was conducted by the same research team [13], three approaches were compared: a BCI with Haptic Knob robotic device for hand opening (the BCI-HK group), a Haptic Knob without BCI control, and a standard rehabilitation therapy. Compared to the standard therapy, a considerable hand function improvement was found only in the BCI-HK group during the 3rd, 12th and 24th weeks of the observation (by 2.14, 1.82 and 2.28 points on FM scale (C–D), respectively, with p < 0.05).
It is important to note that in contrast to our study, in the experiments mentioned above, patients were tested for the ability to control a BCI by motor imagery. Another important difference is higher training intensity: 18 h in total [13, 14], compared to 5 h in our study. However, in our case it is impossible to increase training intensity due to the specifics of the centers where the study is conducted and limited hospital admission periods.
Our study is characterized by the use of several scales for the assessment of arm motor function restoration. FM scale is more universal and detailed [13, 27], while ARAT scale is more functional and allows for the assessment of various hand movements needed for daily tasks [28].
Unlike other studies in this area of research, our study utilizes the exoskeleton as an assistive device. Although there are no data indicating the higher efficacy of the use of this particular device during BCI training sessions, the movement it implements is kinematically closer to the hand and finger physiological movement. It becomes possible due to the use of flexible pneumatic muscles, exo-joints and finger fixators designed with regard to human hand anatomy, which improves ergonomic parameters, helps to avoid rapid fatigue onset during the session, and also excludes traumas if all safety measures are taken. On the other hand, it should be emphasized that it is still impossible to implement a complex functional movement. The exoskeleton contributes to human finger extension; flexion of the fingers is passive, as they are brought back to the initial position by the spring. Such exoskeleton can be only used to stimulate surface and proprioceptive afferentation coming from fingers and hands and as a passive mechanotherapeutic complex with one degree of freedom for the distal arm. The second disadvantage of the exoskeleton is noise from the pneumatic pump that can distract the BCI operator from motor imagery.
Our study demonstrated that arm restoration assessed by ARAT and FM tests did not depend on the time elapsed after stroke and patient’s age in both the experimental and the control groups; thus, this method can be used at various rehabilitation stages and can contribute to better health restoration, which is consistent with the results obtained by other authors [13, 14, 29].
In spite of moderate or medium correlation between the degree of hand function recovery on ARAT scale and the initial severity of neurological deficit, improvement of hand function was observed in the subgroups of patients with initially severe paresis and in the subgroup of patients with initially mild or moderate paresis. Earlier, improvement of hand motor function in patients with severe hand paresis was demonstrated associated with BCI-exoskeleton training [19]. Thus, the severity of motor deficiency cannot be seen as the criteria of exclusion from a BCI-exoskeleton training course. Moreover, for patients with plegia or severe paresis, such training courses are the only available method among those based on active motor paradigm.
The distinctive feature of this study is participation of patients from three clinical centers with statistically negligible differences in sex, time elapsed after stroke, type, localization and lateralization of stroke. Patient screening performed by different experts from different clinical centers and the blind design of the study reduced the influence of subjective factors [27] on the assessment of clinical test results.
Patients who had 2 training courses spaced 6 to 9 months apart observed further improvement of motor activity during the second training course. It is important to study the specifics of motor function restoration throughout several training courses separated by rest periods. Within the framework of this study, the observation will be continued for patients who are scheduled for further hospital admissions.
The most common adverse effect was fatigue; however, none of the patients withdrew from the study because of a serious adverse effect, and on the whole the technology is safe. Because conspicuous fatigue was preceded by insomnia, considerable exercise load during therapeutic procedures before the training session, predisposition to depression and generalized weakness, the probability of this adverse effect can be reduced by selecting the optimal sequence of rehabilitation procedures and by questioning the patient about his condition and sleep problems before each training session.
CONCLUSIONS
Although there is no screening for the ability of a patient to control the brain–computer interface and training sessions based on this technology are not so intensive, preliminary results of this study demonstrate its efficacy with respect to the percentage of patients with clinically significant improvement on ARAT and FM scales.