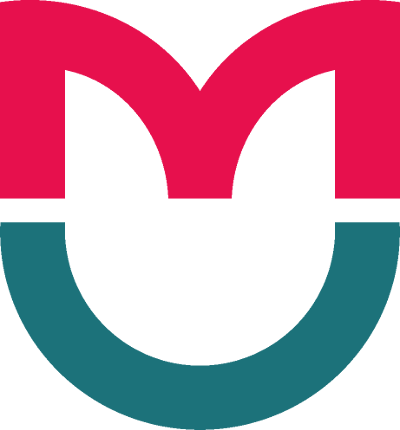
ORIGINAL RESEARCH
Prothrombogenic polymorphic variants of hemostatic and folate metabolism genes In patients with aseptic cerebral venous thrombosis
Research Center of Neurology, Moscow, Russia
Correspondence should be addressed: Marina Yu. Maksimova
Volokolamskoye Shosse, 80, Moscow, 125367; ur.liam@avomixamncn
Funding: the study was performed as a part of the public assignment of the Research Center of Neurology.
Author contribution: Maksimova MYu — concept development and study arrangement, clinical and laboratory data analysis, statistical analysis, manuscript writing; Dubovitskaya YuI — clinical examination of patients, data aquisition and primary analysis of the results; Krotenkova MV — brain MRI, MR venography and neuroimaging diagnosis of cerebral venous sinus thrombosis; Shabalina AA — laboratory analysis of prothrombogenic polymorphic variants of hemostatic and folate metabolism genes.
Cerebral venous sinus thrombosis (CVT) becomes the cause of stroke in less than 1% of patients [1, 2]. In 20–30% of patients, the cause of thrombosis remains unclear, and thrombosis is considered idiopathic [3]. Inherited hypercoagulable conditions significantly increase the risk of CVT [4, 5].
The incidence of cerebral venous sinus thrombosis (CVT) is 1.3 per 100,000 adults per year. Onset may occur at any age, but the highest incidence is noted in people aged 31–50 [6].
Among patients, the women prevail [7]. Despite the improvement of diagnostic and treatment methods, the CVT patients’ mortality remains high and reaches 30% [8].
CVT can be either septic (as a result of complications of purulent otitis media, mastoiditis, sinusitis) or aseptic (occurs as a complication of numerous diseases that increase the tendency to form a blood clot).
Many factors are involved in the development of aseptic CVT: severe dehydration, cardiac disorders (congenital heart defects, heart failure, artificial cardiac pacemaker), cancer, pregnancy, diabetes mellitus, hormonal drugs usage (contraceptive pills, hormone replacement therapy), nephrotic syndrome, polycythemia, essential thrombocytosis, antiphospholipid syndrome, connective tissue disorders and vasculitis (systemic lupus erythematosus, granulomatosis with polyangiitis, temporal arteritis, Behcet's disease), inflammatory bowel disease (Crohn's disease, ulcerative colitis), brain injury, congenital or acquired hemostasis alterations [9–12].
Hereditary thrombophilia is a significant risk factor for aseptic CVT. The most studied are polymorphic variants of factor V (Leiden) and prothrombin genes [13]. Thus, correlation was observed between the risk of CVT and the clotting factor V (OR 4.3; 95% CI — 1.5–12.3) and prothrombin genes (OR 3,6; 95% CI — 1,0–13,1) polymorphisms [14]. In another study, prothrombin gene polymorphism (A replaced with G at position 20210) was found in 19% of patients with CVT [15]. Correlation between the presence of the Leiden mutation and a 5-fold increase in the risk of CVT was confirmed [13]. Analysis of 26 case-control type studies demonstrated that the occurrence of the clotting factor V gene polymorphism (Leiden/G1691A) leaded to 2.4 fold increase of the risk of CVT (95% CI — 1.75– 3.30; р < 0.00001), and the occurrence of the prothrombin gene polymorphism (G20210A) leaded to 5.48 fold increase of the CVT risk (95% CI — 3.88–7.74; р < 0.00001) [16].
Significant polymorphism of clinical manifestations, the absence of pathognomonic symptoms, as well as a variety of options for the onset, course, and localization of thrombosis make the clinical recognition of CVT difficult. Possibilities of CVT early diagnosis significantly expanded due to the neuroimaging methods (CT and MRI) [2, 17, 18]. Currently, MR venosinusography is one of the most reliable methods for the CVT diagnosis [19]. In up to 63% of patients, CVT is complicated by cerebral edema and the formation of necrosis foci with the addition of hemorrhagic component (37.57% of patients) [20]. In such a situation, these foci of necrosis are not infarcts, but arise as a result of the venous outflow slowdown, edema, hypoxia of brain tissue followed by diapedesis of red blood cells and leukocytes through necrotic walls of capillaries and microvessels. The factors predisposing to development of necrosis foci and hematomas in patients with CVT include the female sex, epileptic seizure, impaired consciousness, rapidly spreading thrombosis involving two or more venous sinuses [2, 8, 20].
Often, CVT is suppressed, it is detected unexpectedly by physician during CT or MRI of the brain. For most patients with CVT, a mismatch between the general condition of the patient and the neuroimaging manifestations of the disease is typical [17, 21].
Early diagnosis and well-organized treatment of aseptic CVT in the vast majority of patients leads to clinical recovery. Later, a decrease in the frequency of recurrent thrombosis depends on the timely identification of the thrombosis causes. Almost all researchers agree that recurrent CVT is more severe than the first diagnosed, and its prognosis is much worse.
The aim of the study was to evaluate the frequency of the prothrombogenic polymorphic variants of hemostatic and methionine-homocysteine metabolism genes alleles and genotypes in patients with aseptic CVT.
METHODS
In 2016–2019, 51 patients with aseptic CVT were examined in the 2nd Department of Neurology of the Research Center of Neurology (20 men and 31 women, the average age of the patients was 42.2 ± 13.1). Twenty eight patients were hospitalized in the acute phase of the disease. In 23 patients, the duration of thrombosis was 1–10 months. Control group included 36 healthy volunteers (14 men and 22 women, their average age was 44.7 ± 10.4). The groups under study were comparable by gender and age. Inclusion criteria: 1) acute aseptic CVT confirmed by neuroimaging data; 2) pre-existing aseptic CVT confirmed by neuroimaging data; 3) patients aged 18–75. Exclusion criteria: 1) septic CVT; 2) lower-extremity deep vein thrombosis (DVT) and pulmonary embolism (PE); 3) atherothrombosis involving cerebral arteries, lower limb arteries; 4) other (nonvascular) nervous system diseases; 5) decompensated comorbidities.
In all CVT patients, a detailed study of complaints, general and family history data, the clinical picture of the disease and medical documentation was performed, somatic and neurological statuses were evaluated.
For CVT diagnosis, each patient underwent brain MRI using the Magnetom Verio (Siemens; Germany) and Magnetom Symphony (Siemens; Germany) systems with 3T (Tesla) and 1.5T magnetic field strength respectively. MRI of the brain was performed in the sagittal, axial and coronal planes in T1, T2, T2 d-f (FLAIR), DWI modes with a slice thickness of 1, 3 and 5 mm. Later the images of the cerebral veins and venous sinuses were obtained using MRI in the venosinusography mode. Only after a comprehensive evaluation and a detailed study of the neuroimaging results obtained using standard modes and venosinusography, the CVT diagnosis was considered confirmed. Standard modes, Т2, Т2-FLAIR, were used for assessment of brain tissue focal lesions and for exclusion of other possible pathologies. Image evaluation was performed using the eFilm Workstation software (Merge Healthcare; USA).
Measurement of the hemostasis parameters (fibrinogen level, fibrinolytic activity level, partial thromboplastin time (PTT)) was performed using the ACL-9000 Coagulation Analyzer (Instrumentation Laboratory; USA).
Quantitative determination of D-dimer was carried out by the specific antigen–antibody reaction based immunochemical method using the immunoturbidimetric latex-agglutination assay (Instrumentation Laboratory; USA).
The level of homocysteine in the blood was determined by enzyme immunoassay using the diagnostic kits (AXIS; Norway) and the Immulite 2000 Immunoassay System (Siemens; USA).
DNA diagnostics of prothrombotic polymorphic gene variants was performed using polymerase chain reaction (PCR).
Detection of mutations (polymorphisms) in the human genome was carried out using the DNA-Technology kit (Russia). The patient’s genomic DNA was isolated from whole blood (EDTA tube) using NC sample, the DNA extraction reagent kit (DNA-Technology; Russia).
Two amplification reactions were simultaneously carried out with a sample of isolated DNA using two pairs of allele-specific primers. Real-time PCR was used for simultaneous amplification and for measuring of the studied DNA molecule amount (DTlite Real-Time PCR System; DNA-Technology; Russia). The DNA-Technology (Russia) commercial kits were used. The analysis results were represented by three types of decisions: а) allele 1 homozygote; b) heterozygote; c) allele 2 homozygote.
Studied prothrombogenic polymorphic variants of hemostatic and folate metabolism genes list:
- prothrombin gene, FII (с.G20210A);
- coagulation factor V gene, FV (c.G1691A);
- coagulation factor VII gene, FVII (c.G10976A);
- activated factor XIII (fibrinase) gene, FXIIIA1 (c.G103T);
- fibrinogen beta gene, FGB (c.G455A);
- plasminogen activator inhibitor gene, PAI-1 (c.5G6754G);
- Integrin alpha (Gp1a glycoprotein) gene, ITGA2 (c.C807T);
- Platelet fibrinogen receptor (Gp3a glycoprotein) gene, ITGB3 (c.T1565C);
- methylenetetrahydrofolate reductase gene, MTHFR (c.C677T);
- methylenetetrahydrofolate reductase gene, MTHFR (c.A1298C);
- methionine synthase gene, MTR (c.A2756G);
- methionine synthase reductase gene, MTRR (c.A66G).
Statistical analysis was performed using the IBM SPSS Statistics software, v.23 (IBM Corporation; Russia). Nominal data were described with absolute values and percentages. For categorical data analysis the contingency tables were used. The significance level was taken equal to 0.05 in all comparisons.
Frequency of allele and genotype variants (f) was calculated by the following form. 1 :
where n was the variant (allele or genotype) occurrence, N was the sample size.
The significance of differences in the allele and genotype frequencies between the studied groups was evaluated using the χ2 criterion.
To assess the relative risk, the odds ratio (OR) and its confidence interval (CI) calculations were used at a confidence level of 95% form. 2
.
where a was the frequency of the studied allele (genotype) in the treatment group; b was the frequency of the allele in the control group; c was the sum of other alleles (genotypes) frequencies in the treatment group; d was the sum of other alleles (genotypes) frequencies in the control group.
RESULTS
Isolated CVT was observed in 14 patients (27.5%). In other 37 patients (72.5%) multiple thrombosis was detected involving two or more venous sinuses.
Left transverse sinus thrombosis was detected in 28 patients (54.9%), left sigmoid sinus thrombosis in 26 patients (51%), right transverse sinus thrombosis in 21 patient (41.2%), right sigmoid sinus thrombosis in 14 patients (27.5%), superior sagittal sinus thrombosis in 11 patients (21.6%), inferior sagittal sinus thrombosis in 4 patients (7.8%), tentorial sinus in 3 patients (5.9%), and left cavernous sinus thrombosis in 1 patient.
Among patients with thrombosis of individual venous sinuses, 5 patients (35.7%) were diagnosed with thrombosis of the left transverse sinus, 5 patients (35.7%) were diagnosed with thrombosis of the left sigmoid sinus, 3 patients (21.4%) were diagnosed with thrombosis of the right transverse sinus, and 1 patient was diagnosed with thrombosis of the superior sagittal sinus.
In 4 women, CVT occurred after abortion, in 1 woman onset took place 9 days after delivery, in 7 women (22.6%) CVT developed while taking hormonal drugs (contraceptives). A history of inflammatory diseases of the paranasal sinuses was observed in 3 women and 1 man. Aseptic CVT was confirmed by the absence of systemic inflammatory reactions and inflammatory changes of the laboratory blood parameters.
Among all CVT patients, the focal necrosis in brain tissue caused by thrombosis was observed in 14 patients (27.5%).
Diffuse headache was the chief complaint, which was observed in 45 patients (88.2%). A typical feature of the headache was its worsening after being in a horizontal position. Unsystematic dizziness was noted in 27.5% of patients; it was constant, independent of body position. Nausea occurred in 23.5% of patients. In 7 patients (13.7%), thrombosis began with impaired consciousness. Convulsive seizures were observed in 5 patients (9.8%): focal motor seizures in 2 patients and generalized tonic-clonic seizures in 3 patients. In 9.8% of patients, the meningitis symptoms were noted. Impaired motor function was detected in 3 patients (5.9%): hemiplegia in 1 patient, mild or moderate decrease in muscle strength in the limbs in 2 patients. Speech problems were represented by mild motor aphasia in 4 patients (7.8%).
When calculating the correlation coefficients, a relationship was found between brain tissue focal lesions and the development of convulsive seizures (correlation coefficient r = 0.4; p < 0.01), motor function impairment (correlation coefficient r = 0.5; p < 0.01), speech problems (correlation coefficient r = 0.5; p < 0.01), and depression of consciousness (correlation coefficient r = 0.5; p < 0.01).
Statistical analysis revealed correlation of superior sagittal sinus thrombosis with the development of a convulsive seizure (correlation coefficient r = 0.4; p < 0.01), as well as impaired venous outflow (correlation coefficient r = 0.5; p < 0.01). It also revealed correlation of tentorial sinus thrombosis with the depression of consciousness (correlation coefficient r = 0.3; p < 0.01).
Hemostasis parameters
In the group of patients with CVT the fibrinogen level was 3.7 g/l [2.2; 6.8] (in the control group it was 3.8 g/l [2.9; 5.0]); activated partial thromboplastin time was 29.5 s [22.5; 36.2] (in the control group it was 28.7 s [26.5; 29.9]); antithrombin III level was 112 [98; 119] (in the control group it was 115 [107; 126]); C protein level was 134% [125; 148] (in the control group it was 143% [125; 165]); D-dimer level did not exceed 0.5 μg/ ml. The fibrinogen level increase over 5.5 mmol/l was detected in one patient. Hemostasis parameters of the CVT patients did not differ significantly from the hemostasis parameters of the control group patients.
Homocysteine level in the blood plasma of CVT patients was 16.2 μmol/l [14.6; 17.7] (in the control group it was 7.7 [5.6; 10.9]; p < 0.05).
Prothrombogenic polymorphic variants of hemostasis and folate metabolism genes
Molecular genetic testing for detection of prothrombogenic polymorphic variants of hemostatic genes (tab. 1) and methionine-homocysteine metabolism genes (tab. 2) was carried out in 51 patients with aseptic CVT.
Homozygous (4G/4G) allele 4G at the –675 position of the PAI-1 gene was detected in 11 patients (22.4%). Homozygous allele 103T of the FXIIIA1 gene was detected in 3 patients, homozygous allele 807T of the ITGA2 gene was present in 3 patients. Two patients were the 455A allele of the FGB gene homozygous carriers, 1 patient was the 10976A allele of the FVII gene carrier, and 1 patient was the 1565C allele of the ITGB3 gene carrier. Combination of two alleles in a homozygous state was detected in 2 patients. In the first of them the combination of homozygous 10976A allele of the FVII gene and 6754G allele of plasminogen activator inhibitor gene PAI-1 was observed. In the other of them the combination of homozygous 103T allele of the FXIIIA1 gene and 6754G allele of the plasminogen activator inhibitor gene PAI-1 was present. No patients with homozygous 1691A allele of the FV Leiden and 20210A allele of the FII gene were revealed.
Heterozygous allele 4G at the –675 position of the PAI-1 gene was detected in 28 patients (57.1%). Isolated polymorphic variant of the PAI-1 gene (с. 5G6754G) in the heterozygous state was present in 9 patients (19%). Seventeen patients (34.7%) were heterozygous carriers of 455A of the FGB gene. Thirteen patients (26.5%) were carriers of 10976A allele of the FVII gene, 103T allele of the FXIIIA1 gene, and 1565C allele of the ITGB3 gene. Polymorhic variant 807T of the ITGA2 gene in the heterozygous state was observed in 9 patients (18.4%). Polymorhic variant 1691A of the FV Leiden gene in the heterozygous state was present in 3 patients (5.9%). Two patients (4%) were heterozygous carriers of 20210A allele of the FII gene.
The frequency distribution of hemostasis genes prothrombogenic polymorphic variants alleles and genotypes could be found in tab. 1.
Alleles 5G and 4G of the PAI-1 gene frequency was 48 (49%) and 50 (51%) in the treatment group, in the control group it was 59 (82%) and 13 (18%). According to the calculated odds ratio, 4G allele increases the risk of CVT by more than 4 times (χ2 = 19.337; р < 0.001; OR = 4.728; 95% CI — 2.303– 9.706). Homozygous 4G/4G polymorphism increases the risk of CVT by 10.1 times (χ2 = 6.623; р = 0.011; OR = 10.132 + 1.070; 95% CI — 1.243–82.573), and heterozygous 5G/4G polymorphism increases the risk of the disease by 3.03 times (χ2 = 5.908; р = 0.016; OR = 3.030 + 0.463; 95% CI — 1.223– 7.507).
Comparative analysis of the alleles of FXIIIA1 gene G103T polymorphism frequencies in patients of the treatment group and control group revealed statistically significant differences. Alleles G and Т of the FXIIIA1 gene occurrence was 79 (80.6%) and 19 (19,4%) in the CVT patients group, and 67 (93%) and 5 (7%) in the control group. Thus, the frequency of 103T allele of the FXIIIA1 gene in patients with CVT significantly exceeds the frequency of the allele in the group of healthy people (χ2 = 5.3; р = 0.022). According to the calculated odds ratio, the presence of T allele in the FXIIIA1 gene increases the risk of CVT by more than 3 times (OR = 3.223; 95% CI — 1.142–9.095).
There were no differences in the allele and genotype frequencies distribution of the FII (с.G20210A), FV (c.G1691A), FVII (c.G10976A), FGB (c.G455A), ITGA2 (c.C807T), ITGB3 (c.T1565C), MTHFR (c.C677T), MTHFR (c.A1298C) genes between patients with CVT and healthy volunteers.
Among patients with polymorphic variants of methionine-homocysteine metabolism genes, 66G allele of the MTRR gene in a homozygous state was detected in 5 patients (10.9%). Three patients (6.8%) were homozygous carriers of 677T allele of the MTHFR gene, and 2 patients (4.2%) were carriers of 1298С allele of the MTHFR gene. In one patient, the combination of the homozygous 1298С allele of the MTHFR gene and 66G allele of the MTRR gene was observed. No patients were homozygous carriers of 2756G allele of the MTR gene.
Sixteen patients (36.4%) were identified as heterozygous carriers of 677T allele of the MTHFR gene, 19 patients (41.3%) as carriers of 66G allele of the MTRR gene, 18 patients (39.1%) as carriers of 2756G allele of the MTR gene, and 9 patients (18.8%) as carriers of 1298С allele of the MTHFR gene.
Isolated polymorphic variant of the MTRR gene (с.A66G) in heterozygous state was present in 7 patients (17%).
In 3 patients (7%), the isolated polymorphic variant of the MTR gene (с.A2756G) in heterozygous state was identified.
Among 19 patients who were identified as heterozygous carriers of 66G allele of the MTRR gene, in 9 patients (47%), the heterozygous variant of 1298С allele of the MTHFR gene was also detected.
The frequency values for individual polymorphic variants of methionine-homocysteine metabolism genes are presented in tab. 2.
According to data obtained, the frequency of alleles А and G of the MTRR gene (c.A66G) in the treatment group was 63 (68.5%) and 29 (31.5%). The frequency of these alleles in the control group was 64 (89%) and 8 (11%) respectively. The G allele frequency differences between the group of patients with CVT and the group of healthy people were significant (χ2 = 9.631; р = 0.002; OR = 3.683; 95% CI — 1.564–8.672). Allele G increased the risk of CVT by 3.68 times (χ2 = 9.631; р = 0.002; OR = 3.683 ± 0.437; 95% CI — 1.564–8.672). The presence of A/G polymorphism increased the risk of CVT by 3.5 times (χ2 = 5.784; р = 0.017; OR = 3.519 + 0.538; 95% CI — 1.225–10.104).
Allele G of A2756G polymorphism of the MTR gene frequency in the group of patients with CVT was significantly higher than in the control group (18 (19.6%) against 6 (8%); χ2 = 4.079; р = 0.044), and the frequency of allele A was significantly lower (74 (80,4%) against 66 (92%); χ2 = 4.079; р = 0.044). The odds ratio calculation demonstrated that allele G of A2756G polymorphism correlated with CVT (OR = 2.676 ± 0.501; 95% CI — 1.002–7.142). In patients with CVT, the frequency of A/G heterozygous genotypes was significantly higher than in the control group (χ2 = 4.923; р = 0.027; OR = 3.214 ± 0.540; 95% CI — 1.116–9.257).
When performing the clinical molecular analysis, no significant correlation between the CVT clinical features and the identified prothrombogenic polymorphic gene variants was revealed.
Isolated CVT without any PAI-1 gene polymorphisms (c.5G6754G) was diagnosed in 1 patient, and isolated CVT with PAI-1 polymorphisms in the homozygous or heterozygous state was diagnosed in 13 patients (33%). Multiple CVT without any PAI-1 gene polymorphisms was detected in 9 patients (90%), and multiple CVT with PAI-1 polymorphisms in the homozygous or heterozygous state was identified in 26 (67%) patients (р = 0.244).
Isolated CVT with polymorphic variants of the FXIIIA1 gene (c.G103T) in the homozygous or heterozygous state was observed in 5 patients (31%), and the isolated CVT without any FXIIIA1 polymorphisms was present in 9 patients (27%). Multiple CVT was diagnosed in 11 patients (69%) with polymorphic variants of the FXIIIA1 gene (c.G103T) in the homozygous or heterozygous state, and in 24 patients (73%) with no FXIIIA1 polymorphisms (р = 1.000).
Among patients with polymorphic variant of the MTR 2756 gene in heterozygous state, isolated CVT was observed in 10 patients (56%), and multiple thrombosis was observed in 8 patients (44%). In the absence of polymorphisms of the MTR gene (с.A2756G), isolated CVT was diagnosed in 7 patients (25%), and multiple CVT was diagnosed in 21 (75%) patients (р = 0.06).
In homozygous and heterozygous carriers of 677T allele of the MTRR gene, isolated CVT was detected in 5 patients (21%), multiple CVT was detected in 19 patients (79%). Among patients with no MTRR gene polymorphism isolated CVT was diagnosed in 8 patients (38%), and multiple CVT was diagnosed in 13 (62%) patients (р = 0.323).
DISCUSSION
During our study, CVT was diagnosed in 24 patients (47.1%) aged under 40. The ratio between males and females was 1 : 1.2. Slight prevalence of thrombosis among women of childbearing age could be explained by such risk factors as pregnancy, the use of contraceptives and hormone replacement therapy [22].
Prothrombogenic polymorphic variants of hemostatic genes were diagnosed in 94% of patients. Most often, polymorphic variants of the PAI-1 gene (с.5G6754G) were observed, the product of which was involved in the fibrinolysis regulation. The presence of a polymorphic variant of such gene leads to an increase in the plasminogen activator inhibitor protein functional activity, which in turn contributes to an increased risk of thrombosis [23]. The frequency of heterozygous 4G allele at the –675 position of the PAI-1 gene in the population is 50%, and the frequency of the homozygous allele is 26% [24]. In our study, 4G allele at the –675 position of the PAI-1 gene in heterozygous state (5G/4G) was detected in 57.1% of patients, and in the homozygous state (4G/4G) it was detected in 22.4% of patients.
For the PAI-1 gene, significant differences between the group of patients with CVT and the group of healthy people were observed in the distribution of both alleles and genotypes. In patients with the 4G/4G genotype, the risk of CVT increased by 10.1 times (OR = 10.132; 95% CI — 1.243–82.573), and in patients with the 5G/4G genotype it increased by 3.03 times (OR = 3.030; 95% CI — 1.223–7.507).
The effect of factor FXIIIA1 on CVT still remains unclear [25]. It is possible that G103T polymorphism of the FXIIIA1 gene is associated with the fibrin structure alterations. At a low concentration of fibrinogen, the blood clot density in patient with G103T polymorphism is high, while with an increase in the level of fibrinogen, the density of the blood clot, and therefore its stability, decrease. In the presence of 4G/4G polymorphism of the PAI-1 gene the protective effect of G103T polymorphism of the FXIIIA1 gene decreases drastically [26].
Some authors note correlation of С677Т polymorphism of the MTНFR gene and the risk of CVT [27, 28]. During our study, the prothrombogenic polymorphic variants of the methionine-homocysteine metabolism genes were detected in 86% of patients. Polimorphic variants of the MTRR (с.A66G) and MTR (A2756G) genes were most common. According to literature data, 66G allele of the MTRR gene frequency in the European population reaches 54%. In our study the frequency of the allele was 31.5%. Allelle G of A66G polymorphism of the MTRR gene increases the risk of CVT by 3.68 times (OR = 3.683; 95% CI — 1.564–8.672), allele G of A2756G polymorphism of the MTR gene increases the risk by 2.676 times (OR = 2.676; 95% CI — 1.002–7.142).
The presence of A/G polymorphism of the MTRR gene increases the risk of CVT by 3.5 times (OR = 3.519; 95% CI — 1.225–10.104), and the presence of A/G polymorphism of the MTR increases the risk by 3.2 times (OR = 3.214; 95% CI — 1.116–9.257).
CONCLUSION
Gene polymorphisms 5G6754G (PAI-1), G103T (FXIIIA1), A66G (MTRR) and A2756G (MTR) carriage increases the risk of aseptic CVT and do not affect the thrombosis clinical manifestations.