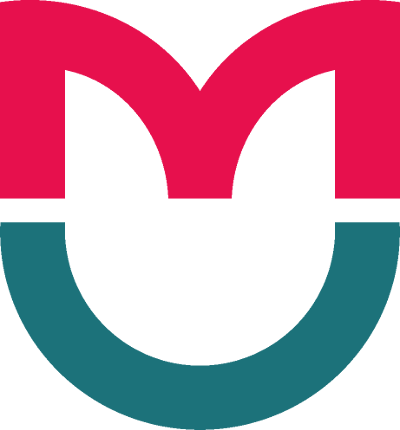
ORIGINAL RESEARCH
Selective changes in expression of integrin α-subunits in the intestinal epithelial Caco-2 cells under conditions of hypoxia and microcirculation
1 National Research University Higher School of Economics, Moscow, Russia
2 Art photonics GmbH, Berlin, Germany
Correspondence should be addressed: Diana V. Maltseva
Vavilova, 7, Moscow, 117321; moc.liamg@avestlamd
Funding: the study was supported by the Ministry of Science and Higher Education of the Russian Federation, project ID RFMEFI61719X0056.
Acknowledgement: the authors express their appreciation to the Center for Collective Use "Human Proteome" (IBMC) for the opportunity to use the equipment for proteome analysis, and to the Center for Precision Genome Editing and Genetic Technologies for Biomedicine of the Pirogov Russian National Research Medical University (Moscow, Russia) for the opportunity to use the molecular biology technologies.
Author contribution: Maltseva DV — working with cultured cells, molecular biology research, data analysis, manuscript writing; Poloznikov AA — proteomic and transcriptomic analysis data processing, bioinformatics analysis, functional analysis of genes, statistical analysis, manuscript writing, study management; Artyushenko VG — interpreting the study results, manuscript reviewing.
Compliance with ethical standards: the study was carried out in accordance with the World Medical Association Declaration of Helsinki.
The intestinal epithelium normally exists in a state of physiological hypoxia due to the commensal bacteria metabolism [1]. However, pathological processes, including inflammatory diseases and tumors, result in additional tissue hypoxia [2]. Yet the dramatic alterations in transcriptome and structure of the intestinal epithelial cell surface could be observed [3], which may result in the most important intestinal epithelium functions impairment, including barrier function and differential absorption, as well as in impaired interaction with microbiota. Thus, the interest to studying the effect of hypoxia on the intestine function have rised constantly in recent years.
There are several approaches to experimental models of hypoxia in vitro: the use of gas mixtures with low oxygen concentration [4], or the use of hypoxia mimetic agents, which increase the intracellular concentration of HIF-1α, the hypoxia-inducible transcription factor. Such hypoxia mimetic agents include oxyquinoline derivatives [5], Fe2+ chelators and dimethyloxalylglycine (DMOG) [4]. However, the most widely used hypoxia mimetic agent is cobalt chloride (CoCl2) [6]. It is also used in the experimental models of intestines in vitro [7]. Cobalt chloride induces stabilization of factors HIF-1α and HIF-2α, which are normally rapidly degraded.
MicroRNA (miRNA) is a class of small (of about 22 nucleotides in length) non-coding RNAs responsible for post-transcriptional gene expression regulation [8]. About one half of mammalian miRNA genes are encoded in introns of other genes. Together with the Argonaute family proteins, miRNAs interact with complementary sites in the target mRNAs, usually by partial binding [8]. Generally, the effective interaction with mRNA requires complementarity to a minimal seed region (matching nucleotides 2–7/8 of the miRNA), although often there are some other matching nucleotides. The Argonaute proteins attract other protein complexes which promote translational repression and the target mRNA degradation. About 60% of all protein-coding genes are regulated by miRNAs. A good deal of evidence suggests the key role of miRNA in the broad range of pathophysiological processes, including the intracellular and intercellular interactions [9], cancer, bacterial and viral infections [10]. Thus, the human miRNA families hsa-let-7e / hsa-mir-125a и hsa-mir-141 / hsa-miR-200 have recently been reported, responsible for regulation of genes ACE2 and TMPRSS2 being a gateway for coronavirus infection [10].
The Caco-2 cell line is widely used in the experimental models of intestinal epithelial barrier in vitro [11]. This cell line was first derived from patient with colon adenocarcinoma. However, when cultured, the Caco-2 cells become differentiated and form the monolayer of polarized cells with prismatic phenotype, expressing a number of intestinal brush border enzymes and membrane transporters specific to small intestine epithelial cells [12]. The proteome analysis data indicate that the differentiated Caco-2 cells resemble the native intestinal epithelium [13]. Our study is focused on the effect of hypoxia on the intestinal epithelial cells’ adhesive properties. Integrins play a vital part in adhesion. Integrins are a superfamily of transmembrane receptors responsible for interaction with the extracellular matrix components and the other cell’s surface proteins (including those of bacterial cells). All integrins are heterodymers consisting of α- and β-subunits. The 18α- and 8β-subunits have been identified in mammals. In the Caco-2 cells the major subunits include α-2, 5, V and β-1, 3, 4 subunits [14], which is consistent with the integrin subunit expression profile of the human primary enterocytes [15]. The study was aimed to assess the Caco-2 cells’ transcriptome and proteome alterations under the normal and hypoxic conditions, as well as to compare the results with alterations in the transcriptome of Caco-2 cells cultured in the microfluidic chip.
METHODS
Caco-2 cell culture
The immortalized human colorectal adenocarcinoma Caсo-2 cells (Institute of Cytology RAS; St. Petersburg) were grown in the MEM culture medium (Gibco; USA) with the following supplements: 20% fetal bovine serum (FBS, Gibco; USA), 1% (v/v) non-essential amino acids solution (Gibco; USA), penicillin (100 U/mL) and streptomycin (100 μg/mL) (Gibco; USA). The Caco-2 cells were cultured in the 6-well plates (Corning; USA) for 23 days up to the state of fully differentiated enterocytes. The culture medium was changed every 2–3 days. In order to simulate hypoxia, CoCl2 (Sigma-Aldrich; USA) was added to the culture media (Sigma-Aldrich; USA) to concentration of 300 μM and incubated for 24 h in accordance with the previously reported algorithm [7]. After incubation, the cells were washed with the 1× DPBS (Gibco; USA), and lysed for transcriptome and proteome analysis using the previously reported method [16].
The the effect of hypoxia on the cell monolayer was assessed by impedance spectroscopy. Prior to plating of Caco-2 cells on the 96-well plates with membrane inserts (Corning; USA), all the wells were filled with culture medium (50 μl into the upper chamber, 235 μl into the lower chamber) and incubated for 1 h at a temperature of 37 °С in the 5% CO2 atmosphere. Then the Caсo-2 cells were plated, approximately 5,600 cells per membrane insert, in the volume of 50 μL, and cultured for 23 days up to the state of fully differentiated enterocytes. The culture medium was changed every 2–3 days. CoCl2 was added to the culture medium in order to induce hypoxia as described above.
In order to assess the influence of perfusion on the transcriptome, the Caco-2 cells were cultured in the microfluidic chip, which was designed during execution of the project “Study of Bacterial Adhesion in a Microfluidic Model of the Human Intestinal Barrier” supported by the Ministry of Science and Higher Education of the Russian Federation. The microfluidic chip is the flow system containing two cell culture wells, each of the wells is separated by membrane with the 0.4 μm pore size.
First, the wells of microfluidic chip were coated with laminin 332 (Biolamina; Sweden). For that, 57 μL of laminin 332 solution in DPBS (0.01 mg/mL) was added to the well and incubated for 24 h in the fridge at a temperature of 4 °С. Then the Caco-2 cells were detached from the substrate using the 0.25% trypsin-EDTA and the Hank's Balanced Salt Solution. After that the cells were resuspended in the culture medium and counted with the Countess Automated Cell Counter (Invitrogen; Germany). The cell suspension containing 20,000 Caco-2 cells in the volume of 50 μL was added to each well of the chip, then the microfluidic chips were incubated in the cell incubator (5% СО2, 37 °С) for 2 h. After the incubation the chip was connected to peristaltic pump to ensure perfusion in the 50 μL/h mode. The cells were cultured for 2 days in the cell incubator (5% СО2, 37 °С) with regular (at least twice a day) measurement of transepithelial electrical resistance in order to obtain the value of 350 Ohm·cm2. The cells being in state of fully differentiated enterocytes were washed with the 1× DPBS (Gibco; USA) and lysed for transcriptome analysis using the previously reported method [16].
Study of the collagen IV and laminin 332 membrane coating impact on the Caco-2 cell proliferation
The surface of the Transwell membrane inserts of the Corning 96-well plate was coated with collagen IV and laminin 332. For that, 50 μL of collagen IV solution in DPBS (0.1 mg/mL) or 57 μL of laminin 332 solution in DPBS (0.01 mg/mL) was added to the appropriate inserts and incubated for 24 h at a temperature of 4 °С. Then the inserts were washed to remove the unbound extracellular matrix components, and the plate wells were filled with culture medium (50 μl into the upper chamber, 235 μl into the lower chamber). The plate was put in the cell incubator (5% СО2, 37 °С) and incubated for 1 h. After incubation, the culture medium was removed from membrane inserts; the cell suspension containing 5,000, 10,000 or 20,000 cells was added to the membrane inserts and incubated in the cell incubator (5% СО2, 37 °С) for 96 h. After cultivation, MTS reagent with a concentration of 0.5 mg/mL was added to the culture medium and incubated at a temperature of 37 °C for 4 h. Then the optical density was measured at a wavelength of 590 nm.
Impedance spectra measurement and calculation of electrical parameters
The impedance spectra were measured in the frequency range of 40–20,000 Hz using the impedance spectroscopy measurement system (Bioclinicum; Russia) and the STX100C96 electrode (World Precision Instruments; USA) at room temperature. In order to obtain the average values of the electrical parameters, three distinct membrane inserts with cells were used. Calculation of the cell monolayer electrical parameters (transepithelial electrical resistance (TEER), capacity C, and monolayer resistance RM) was performed using the CEISA impedance fitting software (Bioclinicum; Russia) and the cell monolayer equivalent circuit (fig. 1). The further statistical analysis was carried out using the R 3.5 programming language and the RStudio graphical user interface. The statistical significance of the observed differences in the TEER values was evaluated by the two-way analysis of variance (ANOVA). The P values of less than 0.05 were regarded as statistically significant.
Transcriptome analysis
Transcriptome analysis was carried out using the Gene Chip Human Transcriptome Array 2.0 microarray for mRNA and the Gene Chip miRNA 4.0 microarray for miRNA (TermoFisher Scientific-Affymetrix; USA). RNA isolation, quality control and quantification were performed in accordance with the previously reported method [16]. The RNA integrity number (RIN) values exceeded 9.5 in all studied samples. The cDNAs were synthesized from 500 ng of isolated total RNA. Sample preparation, hybridization, microarray washing, staining and scanning were carried out in accordance with the manufacturer’s instructions. The CEL files obtained by the microarray scanning were processed using the Transcriptome Analysis Console 2.0 software package (TermoFisher Scientific-Affymetrix; USA). Microarrays contained multiple probes designed to hybridize to different gene regions for each gene, which comprised the multiple probe set (probe set). The probe sets not corresponding to any of the known genes (un-annotated probe sets) were excluded from analysis. When assessing the gene expression, the threshold signal intensity was set at 6.0 on the Affymetrix logarithmic scale.
Real-time PCR (qPCR)
The qPCR was performed in accordance with the previously reported method [16]. The real-time PCR product accumulation detection was based on SYBR-Green I fluorescence. The ACTB and GAPDH transcripts were used as reference genes (average threshold cycle values: 25.3 and 23.4 respecively). The nucleotide sequences of primers (Syntol; Russia) are presented in tab. 1.
Caco-2 cells proteome analysis
The Caco-2 cells sample preparation, total protein extraction, hydrolysis and the subsequent procedures were carried out in accordance with the previously reported method [16]. After trypsinolysis, the supernatant fraction was analysed using the Q Exactive HF mass spectrometer with nano-electrospray ionization (nESI) source operated in the positive ionization mode (Thermo Fisher Scientific; USA), with the emitter voltage of 2.1 kV and the capillary temperature of 240 °C. The protein level quantification was performed using the Progenesis IQ software (Waters; USA) with default settings. The proteins were identified using the SearchGUI v.3.3.1 software and the HumanDB database (UniProt Release 2018_05) with the following search parameters: digestive enzyme trypsin, monoisotopic mass accuracy ±5 ppm, mass measurement accuracy on tandem MS/MS data (tandem mass spectrometry) ±25 ppm, and the chance to skip one cleavage site. In order to assess the differentially expressed proteins, the raw data were analyzed with the MaxQuant 1.6 software (Max-Planck-Institute of Biochemistry; Germany) (iBAQ algorithm). The further data processing was carried out using the Perseus software and the R 3.5 programming language with the integrated development environment RStudio 1.1 (R-Tools Technology; USA). Student's t-test was used to determine the statistical significance of the differences observed.
Statistical data processing
The raw microarray data were normalized using the oligo package for the R programming language [17]. The data obtained were log2-transformed. Student's t-test was used for analysis of differential expression of genes and miRNA. The false discovery rate (FDR) was controlled using the Benjamini– Hochberg method developed for the multiple hypotheses testing [18]. The miRNA false positive rate was evaluated using the previously reported algorithm [19].
Functional gene annotation was performed using the DAVID version 6.8 databases and algorithms [20]. The experimentally supported miRNA-target interactions were exported from the DIANA-TarBase version 8 database [21]. The miRNA-binding sites in the 3'-untranslated regions (3'-UTR) of miRNA targets were predicted with the miRWalk tool [22]. The miRIAD database was used to explore the intragenic miRNA and their host genes [23].
RESULTS
Exposure to CoCl2 mimics hypoxia in Caco-2 cells and upregulates the integrin α-subunits
According to literary sources, coating of the membrane used for Caco-2 cell culture with extracellular matrix proteins, such as collagens and laminins [24], can greatly affect the rate of monolayer formation and cell differentiation. Therefore, when optimizing the microfluidic intestinal barrier model culture conditions, we assessed the effect of the collagen IV and laminin 332 membrane coating on the Caco-2 cell proliferation. The study was carried out using the Transwell Corning membrane inserts. Coating of membrane with laminin 332 more than doubled the Caco-2 cell proliferation (fig. 2). Therefore, cell culture on the laminin 332-coated membrane was selected for further investigation.
In order to mimic hypoxia, the Caco-2 cells were exposed to CoCl2 (see “Methods”). The impact of hypoxia on the cell monolayer was assessed by impedance spectroscopy. Impedance spectroscopy allows one to measure the electrical impedance (total resistance to sinusoidal alternating current) as a function of the frequency of applied electrical current (see “Methods”) [25]. The efficiency of impedance spectroscopy for such kind of research has been previously reported [25]. The average TEER values, both for hypoxia and normoxia, are above 3,000 Ohm (corresponds to resistivity of 429 Ohm∙cm2). The TEER values depend both on the intracellular resistance resulting from the state of transmembrane channels responsible for transport of ions, and the state of tight junctions responsible for paracellular resistance. For example, it is known that the TEER values rapidly decrease with decreasing concentration of calcium in the medium, since the calcium ions are essential for maintenance of the tight junctions’ normal struсture. On the other hand, cell death results in the broken integrity of the membrane and the significantly decreased intracellular resistance. High TEER values observed throughout the experiment are the indication of intact tight junctions and lack of severe cytotoxicity resulting in cell death (fig. 3).
Analysis of transcriptome for the treated and reference cells was carried out using the Affymetrix microarrays (TermoFisher Scientific-Affymetrix; USA). The significantly increased expression of genes involved in response to hypoxia in accordance with [26] was detected in samples exposed to CoCl2, the alterations revealed were validated using qPCR (tab. 2). The significant alterations of expression (twofold or more; FDR < 0.05) were detected in 165 genes. The functional annotation of genes using the DAVID databases and algorithms [20] showed the significant enrichment of the HIF-1 signalling pathway (KEGG-pathway hsa04066), mediating the response to hypoxia (tab. 3). Functional annotation revealed no enrichment of antioxidant defence genes (GO: 0016209, antioxidant activity). Mass spectrometry of proteome for the treated and reference cells failed to detect the significant uncrease in the level of the following four proteins encoded by the HIF-1 signalling pathway genes: ENO2 by 19.6, HMOX1 by 29.1, PDK1 by 2.8, and SLC2A1 by 2.2 times respectively. Proteome analysis revealed the total of 120 proteins, the expression of which significantly changed under hypoxia-mimicking conditions.
Comparison of the CoCl2-treated and reference cells’ transcriptomes revealed the significantly increased expression of two genes encoding the integrin α-subunits: under hypoxia-mimicking conditions the expression of ITGA2 increased by 3.2 times (p = 0.02), and the expression of ITGA5 increased by 1.9 times (p = 0.0088). The alterations were validated using qPCR: the expression of ITGA2 and ITGA5 increased by 3.5 and 2.0 times respectively. No expression increase was observed for genes encoding β-subunits. During the proteome analysis, among all β-subunits only the β1- and β4-subunits were detected, and there were no alterations in expression of those under hypoxia-mimicking conditions. In Caco-2 cells, these two subunits made up more than 90% of the total β-subunits number [14]. No α-subunits were detected in the proteome, which could be due to insufficient analysis sensitivity. Furthermore, it had been shown before that the levels of α2- and α5-subunits in the Caco-2 cells were 10 and 100 times lower than the level of β1-subunits [14]. It's important that when growing the Caco-2 cells in the microfluidic chip, the expression of ITGA5, on the contrary, decreased by 2.1 times. The 1.6-fold decrease in the expression of gene LAMA1 encoding the normally abscent in the healthy gut laminin α1 chain was observed. This is evidence of the conditions closer to physiological established in the microfluidic chip. It should be noted that no significant alterations in genes involved in response to hypoxia and HIF-1 signalling pathway compared to static condition have been revealed.
Altered ITGA5 expression is associated with alterations in the regulatory miRNAs’ expression
To explore the possible cause of the ITGA2 and ITGA5 integrin expression alteration in response to hypoxia simulation, we analyzed miRNAs involved in regulation of these genes. Using the Affymetrix miRNA microarrays, the miRNA-transcriptomes of the CoCl2-treated and reference cells were assayed. Expression of nine miRNAs significantly changed under hypoxia-mimicking conditions. Of those two miRNAs, hsa-miR-23b-5p and hsa-miR-766-3p, turned out to be the experimentally validated ITGA5 gene expression regulators [27, 28]. In the hypoxic state the expression of those decreased by 2.2 and 2.1 times respectively (p = 0.046), which was opposite to the expression alteration of their target gene ITGA5.
Analysis of the discussed miRNAs binding to 3'-UTR of the ITGA5 mRNA showed that both miRNAs were perfectly complementary to the target mRNA in the seed region (nucleotides 2-7/8 of miRNA) (fig. 4). Perfect complementarity to the seed region is essential for efficient interaction between miRNA and mRNA. Moreover, both miRNAs are able to pair with the ITGA5 mRNA outside the seed region, which further increases the strength of binding (see fig. 4).
Genes of both miRNAs are located in introns: hsa-miR-23b- 5p is located in the intron of gene AOPEP, and hsa-miR-766-3p is located in the intron of gene SEPTIN6. It should be noted that under conditions of hypoxia simulation no significant alterations in the listed hosting genes’ expression have been detected. This may indicate that these miRNAs have independent transcription start sites, which have been previously found in many intronic miRNAs [29].
DISCUSSION
The increased expression of genes involved in the HIF-1 signalling pathway was detected under hypoxic conditions simulated by exposure of colon adenocarcinoma Caco-2 cells to cobalt (II) chloride. The results obtained were consistent with literary data on the transcriprome alterations due to treatment of Caco-2 [30] and Caki-1 [31] cell lines with cobalt (II) chloride.
Hypoxia modeling has revealed the significantly increased expression of genes ITGA2 and ITGA5. These genes encode the integrin receptor α2- and α5-subunits. Since no significant alterations of β-subunits have been detected, it can be assumed that the proportion of receptors containing α2- and α5-subunits have increased under hypoxic conditions. We discovered that in the static Caco-2 cell culture the expression of ITGA5 was 2.1 times higher compared to cell culture in the microfluidic chip. Microcirculation brings the cell culture conditions closer to physiological conditions and increases the nutrient supply. Thus, alterations in the integrin receptor expression profile (higher proportion of α2- and α5-subunits) may be the Caco-2 cells’ response to the growth conditions deterioration. During the study we have discovered the potential regulators of ITGA2 and ITGA5 expression, the hsa-miR-23b-5p and hsa-miR- 766-3p miRNAs. The expression of those decreased under hypoxic conditions. In should be noted that in addition to the listed miRNA activity there may be cofactors promoting the expression of ITGA2 and ITGA5, for example, transcription factors involved in regulation of the discussed genes, which are also found to have altered expression levels.
The existing literature reports little information on the two identified miRNAs. Thus, it is known that the hsa-miR-766-3p downregulation results in increased proliferative activity of renal cell carcinoma cells [32] and hepatocellular carcinoma aggression [33]. Downregulation of hsa-miR-23b-5p results in increased proliferation and migration of the lung adenocarcinoma cells [34].
In order to become a receptor, integrin chains must form an αβ-heterodymer. In the intestinal epithelial cells, one of the major partners for α2- and α5-chains is β1-chain. The main ligands of the α2β1 integrin receptor are laminin, collagen and epithelial cadherin, and the receptor α5β1 binds to fibronectin [35]. These proteins, in turn, function as ligands for bacterial adhesins. For example, the YadA adhesin found on the outer membrane of the gram-negative bacteria Yersinia pseudotuberculosis and Yersinia enterocolitica (zoonotic bacterial pathogens causing pseudotuberculosis and yersiniosis) binds to collagen, laminin and fibronectin [36]. Furthermore, invasin, the protein of the outer membrane of the Yersenia genus pathogenic bacteria, selectively binds the β1 family integrin receptors, which results in translocation of bacteria across the epithelial layer [37]. It should be noted that in one of the studies simulation of hypoxia using DMOG was associated with a lower sensitivity of Caco-2 cells grown on plastic to Yersinia enterocolitica [38], which, according to the authors, was due to lower expression of integrin β1-chain. In our study, no alterations in the integrin β1-1 chain expression in cells grown on the permeable membrane insert were observed. This seems to indicate the importance of cell culture conditions used in such experiments. However, the influence of the specific hypoxia mimetic agents cannot be excluded.
Thus, the intestinal epithelial cells hypoxia, insufficient nutrient supply and excess metabolic waste result in altered integrin receptor expression profile, which may contribute to increased susceptibility to a number of bacterial pathogens. Hsa-miR-23b-5p and hsa-miR-766-3p miRNAs may function as regulators of the discussed process.
CONCLUSION
The use of cobalt (II) chloride made it possible to simulate hypoxia in the Caco-2 cell line. Transcriptome analysis revealed activation of key HIF-1 signalling pathway components, as well as alterations of the integrin expression profile in the intestinal epithelial cells. The discussed process could be regulated by the hsa-miR-23b-5p and hsa-miR-766-3p miRNAs, the expression of which inder hypoxic conditions decreased by 2.2 and 2.1 times respectively. Microcirculation has the opposite effect on the expression of ITGA5 and has no effect on the HIF-1 signalling pathway genes’ expression.