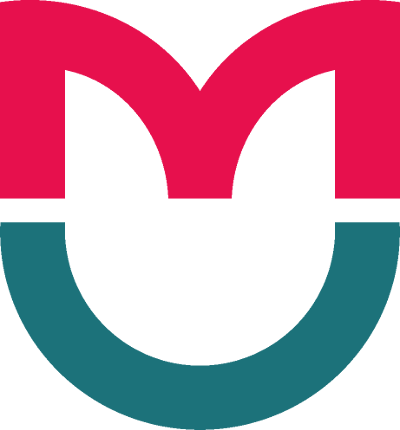
ORIGINAL RESEARCH
A method for rapid generation of model intestinal barriers in vitro
1 National Research University Higher School of Economics, Moscow, Russia
2 SRC Bioclinicum, Moscow, Russia
Correspondence should be addressed: Sergey V. Nikulin
Vavilova, 7, Moscow, 117321; moc.liamg@b.c.nilukin
Funding: the study was supported by the Russian Science Foundation, project № 16-19-10597.
Author contribution: Nikulin SV — cell culture work, preparation of samples for transcriptome analysis, data analysis, manuscript preparation; Poloznikov AA — analysis of transcriptome data, manuscript preparation; Sakharov DA — supervision, manuscript preparation.
Compliance with ethical standards: the study complies with the 1964 Helsinki Declaration and its later amendments.
Intestine is an important organ where food is digested, nutrients and drugs are absorbed into the blood, and interaction of microorganisms with host cells occurs. One of the main intestine functions is the barrier function. Intestinal barrier dysfunction has been implicated in numerous health conditions including inflammatory and autoimmune diseases [1]. Cancer chemotherapies often lead to gut barrier dysfunction [2].
The pathways for transporting molecules through the intestinal barrier include active transport, passive diffusion through the cell membrane, and passive diffusion through the intercellular spaces in between epithelial cells. Epithelial tight junctions are the key structures regulating paracellular trafficking of molecules [3]. Tight junctions are multi-protein complexes located close to the apical surfaces of the epithelial cells and consisting of different cytoplasmic and transmembrane proteins such as occludin and claudins. Due to dynamic changes of tight junctions, intestinal permeability can change rapidly [4]. The tight junction protein complexes are dynamically modulated by various signaling molecules such as kinases (c-Src, c-Yes, etc.) and cytokines (PHOα, interferron γ, etc.) [5]. Since tight junctions play an important role in the functioning of the intestine both in normal and in pathology, it is crucial to develop appropriate in vitro models to evaluate various effects on tight junctions [6, 7].
There are a few different techniques for measuring the paracellular permeability reflecting the integrity of tight junctions. In particular, measurements of molecular marker concentrations on both sides of the barrier are widely used [8, 9]. However, this method is time-consuming and not easy to use. Alternatively, transepithelial electrical resistance (TEER) can be used to monitor changes in epithelial cell culture integrity [10]. Using this quantitative technique takes researchers less time and is well suitable for the high-throughput screening; TEER measurement accuracy can be increased by applying impedance spectroscopy [11, 12].
Currently, Caco-2 cell line is widely used to model the intestinal barrier in vitro [13]. The Caco-2 cells were originally derived from a colon adenocarcinoma — the cells turned out to be able to spontaneously differentiate into a monolayer of cells with many properties typical of the small intestine epithelium [14, 15]. Caco-2 cells are known to form tight junctions as they grow and differentiate, and the cell density of their tight junctions is higher than in normal colon [14, 15]. This makes Caco-2 cell line a valuable transport model system for studying tight junction processes. However, for a wider application of this cell model, it is necessary to improve experiment performance. This can be partially achieved by cell culture automation, e.g. using microfluidic chips [16–18] however, it would be very helpful to optimize cultivation conditions to make it possible to have ready for use cell models more rapidly.
Our study was aimed at determining the optimal cultivation conditions for Caco-2 cells that would allow obtaining functional cell monolayers with tight junctions as quickly as possible.
METHODS
Caco-2 cells were obtained from the Institute of Cytology of the Russian Academy of Sciences (Russia). The cells were cultured in MEM (Gibco; USA) supplemented with 20% fetal bovine serum (Gibco; USA) and 1% penicillin-streptomycin (Gibco; USA). Cells were maintained at 37 °C in an incubator with 5% CO2. Subcultivation was performed every 2 or 3 days according to the standard procedure using a trypsin EDTA solution (PanEco; Russia). Cell counts were performed using Automated Countess Cell Counter (Gibco; USA) according to the manufacturer's recommendations.
Cells were seeded onto 1.0 μm pore-size polyester inserts (PET) HTS Transwell-96 (Corning; US). Before seeding, a part of membranes was coated with laminin-332 (BioLamina; Sweden) and another part was coated with type IV collagen (Imtek; Russia): 30 μl of proten in DPBS solution with 10 μg/ml concentration was added to each membrane insert. Then, 96- well plates with membrane inserts were incubated at 4 °C for 24 h. After incubation, protein solutions were removed from all wells, and each well was washed 3 times with 100 μl of DPBS solution.
Right before cell seeding, HTS membrane inserts were filled with culture medium (50 μl into the upper chamber and 235 μl in the lower chamber) and incubated in a cell incubator for one hour. Then different quantities of cells were added to each membrane insert (6,250, 12,500 or 25,000 cells per well) to 50 μl of the culture medium to achieve initial cell density of 43,700, 87,400 and 174,800 cells per cm2, respectively. Each experiment was carried out in three repeats. 96-well plates with membrane inserts were incubated in a cell incubator throughout the experiment.
To determine TEER values in 24 h and 48 h from the onset of the experiment, impedance spectra measurements were made using an impedance spectrometry system (BioClinicum; Russia) and original electrodes (BioClinicum; Russia). TEER values were calculated following the previously described equivalent electrical circuit [19] using CEISA Impedance fitting (BioClinicum; Russia). Statistical analysis of obtained data was carried out using the programming language R 4.0 with the integrated development environment RStudio 1.1 (RStudio PBC; USA). To assess the statistical significance of the observed TEER differences, we used a three-factor (substrate type, initial cell density, and time from the onset of the experiment) Analysis of Variance (ANOVA) Tukey-adjusted for multiple comparisons. Differences were considered significant at p < 0.05.
To obtain microscopic images of Caco-2 cells on various substrates, some of the wells in 96-well plates (Corning; US) was coated with laminin-332 (BioLamina; Sweden) and type IV collagen (Imtek; Russia) using the protocol similar to membrane inserts coating. 50 μl of protein solution per well was used for coating. Each well was then loaded with 100 μL of cell suspension in a complete culture medium with concentration of 100,000 and 200,000 cells per 1 ml (corresponding to the initial cell densities 31,300 and 62,600 per cm2). The plates were further incubated in a cell incubator. Microscopic images were obtained using PrimoVert inverted microscope (Carl Zeiss; Germany).
To obtain fully differentiated Caco-2 cells, culturing was carried out according to the procedure described in [11, 19]. Analysis of gene expression levels in differentiated and undifferentiated Caco-2 cells was carried out using microchips GeneChip Human Genome 1.0 ST (Affymetrix; USA) [20]. Cells were lysed with QIAzol lysis buffer (Qiagen; Germany). Total RNA was isolated using miRNeasy Mini Kit (Qiagen; Germany) according to the manufacturer's protocol. Concentration of isolated total RNA was measured using NanoDrop 1000 spectrophotometer (Thermo Fisher Scientific; USA). RNA quality was assessed using the Experion system (Bio-Rad; USA). For hybridization on microchips, 500 ng of each RNA sample was used. The experiment was carried out in three repeats.
The results were processed using TAC 4.0 software (Thermo Fisher Scientific; USA). The statistical significance of differences in expression levels between differentiated and undifferentiated Caco-2 cells was assessed by single factor variance analysis (ANOVA) adjusted with Benjamini–Hochberg. The significance threshold was 0.05. Genes with an expression level less than 6.0 on the Affymetrix logarithmic scale were considered not expressed.
RESULTS
Based on TEER measurements (fig. 1) for membrane inserts made 24 h after the cell seeding, TEER was shown to increase as initial cell density increased. E.g., for uncoated control membrane inserts, TEER values were found to be about 118 Ω.cm2 higher for the maximum initial cell density compared to the minimum initial cell density (p < 0.001). No significant differences were found between intermediate and minimum initial cell densities (p = 1). Similar results were observed for the laminin-332 coated wells. In case of laminin-332 coating, no significant TEER differences were found as compared to uncoated control wells for all tested initial cell densities (p = 1).
In case of type IV collagen coating, the results differed significantly. Considerable differences were observed between the minimum tested initial cell density of 43,700 cells per cm2 and 87,400 cells per cm2 (an increase of 147 Ω.cm2; p < 0.001), as well as between the minimum tested initial cell density of 43,700 cells per cm2 and 174,800 cells per cm2 (an increase of 208 Ω.cm2; p < 0,001). Thus, in case of type IV collagen coating, the dependence of TEER values on initial cell densities was more significant. For the initial densities 87,400 cells per cm2 and 174,800 cells per cm2, a strong increase in TEER (188 Ω.cm2 and 142 Ω.cm2, respectively) was also found in type IV collagen coated wells as compared to control polyester wells (p < 0,001 in both cases).
After 48 h from the beginning of the experiment, TEER values in the uncoated control wells increased significantly for the 87,400 initial cell density and for the 174,800 initial cell density compared to the measurements taken 24 h after cell seeding (202 Ω.cm2 and 110 Ω.cm2, respectively; p < 0.001 and p = 0.002, respectively). At the same time, in case of minimal 43,700 initial cell density, there was no significant difference between TEER values for 48 h measurements and 24 h measurements (p = 1).
In the case of laminin-332 coating, similar dynamics was observed comparing 48 h vs. 24 h TEER values: for 43,700 cells/cm2 initial density, no significant difference was found (p = 0.1); for 87,400 cells/cm2 initial density, TEER values significantly increased by 165 Ω.cm2 (p < 0.001) however, for the 174,800 cells/cm2 initial density, the observed growth by 83 Ω.cm2 was not statistically significant (p = 1). On the other hand, in case of type IV collagen coated wells, a significant TEER increase (175 Ω.cm2) was reported in case of the minimum initial cell density only (p < 0.001) while no significant differences between related 48 h and 24 h measurements were found for 87,400 cells/cm2 and 174,800 cells/cm2 initial densities (p = 0.2 and p = 1, respectively).
Interestingly, for uncoated wells and for laminin-332 coated wells, 48 h TEER values were still dependent on the initial cell densities. In case of uncoated wells, 48 h TEER values were higher as compared to the 43,700 cells/cm2 initial density for both 87,400 initial density (172 Ω.cm2; p < 0.001) and 174,800 cells/cm2 initial density (188 Ω.cm2; p < 0,001). Similar results were obtained in case of laminin-332 coating. At the same time, 48 h TEER values in the wells coated with type IV collagen were not dependent significantly on the initial cell density (p = 1 in all cases) and were more than 200 Ω.cm2.
In order to evaluate the effect of type IV collagen/ laminin-332 substrate coating on cell morphology and growth rate, live-cell imaging of Caco-2 cells was performed in 24 hours after cell seeding (fig. 2). It turned out that for control uncoated wells and laminin-332 coated wells, 100% confluence was not achieved at the considered initial cell densities. However, in case of type IV collagen coating, about 80% of surface was covered in wells with 31,300 cells/cm2 initial density, and a monolayer was formed in wells with 62,600 cells/cm2 initial density. In type IV collagen coated wells, an increased amounts of elongated spindle shaped cells were observed compared with control wells and laminin-332 wells.
Transcriptomic analysis showed that in both differentiated and undifferentiated Caco-2 cells, expression of all type IV collagen chains was at a relatively low level (<7 according to Affymetrix logarithmic scale) (see table). Moreover, in the process of cell differentiation a slight decrease in expression of COL4A1 and COL4A6 genes was observed.
Integrins α1β1 and α2β1 are known to be the main receptors of type IV collagen [21]. Transcriptomic analysis showed that ITGB1 gene (β1-integrin chain) was expressed at a sufficiently high level in both differentiated and undifferentiated Caco-2 cells (10.0 and 10.1 in Affymetrix logarithmic scale respectively), and its expression did not significantly change during the process of differentiation (p = 0.4). ITGA1 gene (α1-chain integrin) was also expressed in both differentiated and undifferentiated Caco-2 cells (8.6 and 9.0 in Affymetrix logarithmic scale, respectively) however, its expression slightly decreased in differentiated cells (1.3 times lower; p = 0,002).
Similar results were obtained for ITGA2 gene (α2 integrin chain): the average expression values were 9.2 for differentiated cells and 9.5 for undifferentiated cells while a small 1.3 time decrease of expression in differentiated cells was statistically significant (p = 0,04).
DISCUSSION
The experiment showed that type IV collagen was the most effective substrate significantly accelerating the process of formation of functional epithelial intestinal barrier. Some chains of type IV collagen (α1, α2, α5 and α6) are known to be involved in formation and development of the intestine, and the proteins can be synthesized in both epithelial and mesenchymal cells [22]. Based on the results of transcriptomic analysis, Caco-2 cells cannot sufficiently synthesize type IV collagen while at all stages, the cells express receptors for type IV collagen which indicates that type IV collagen may have effect on the cell processes.
Based on the obtained cell images, it can be concluded that type IV collagen promotes both proliferation and migration of Caco-2 cells. To date, an extended data has been accumulated showing that type IV collagen stimulates both adhesion and migration of Caco-2 cells [23-25]. Type IV collagen is also known to stimulate proliferation of other epithelial cell types [26, 27]. Thus, the obtained results are well consistent with the data available from earlier studies.
Effect of type IV collagen on Caco-2 monolayer TEER values has already been studied, and it was found that a few days after cell seeding, TEER values were significantly higher in the wells coated with type IV collagen however, the dynamics of TEER changes during the first few days of cell culturing was not determined [28]. In the present study, it was shown that type IV collagen affects not only the TEER values but also the time periods when TEER values (about 200 Ω.cm2) [9] become sufficient for barrier model experiments — it can be really achieved within 24 h period. The obtained results can be easily applied both to other static in vitro models of barrier tissues and to dynamic microfluidic systems [29, 30].
CONCLUSIONS
In our experiments, we found that using the type IV collagen coated substrate for cell growth significantly increased the rate of proliferation and migration of Caco-2 cells. This made it possible to obtain functional monolayers of epithelial cells with tight junctions contacts within 24 hours. The optimal initial cell densities were also determined. For obtaining an intestinal barrier model in vitro during 24-hour period, the initial cell density should be 90,000 to 200,000 cells per cm2. Type IV collagen was found to be poorly expressed by Caco-2 cells while the expression levels of type IV collagen receptor proteins were relatively high in the cells. Another basement membrane component laminin-332 was shown to have no significant effect on the rate of formation of functional epithelial cell monolayers.
The findings can be used to further improve the performance of experiments involving in vitro intestinal barrier models both in static conditions and in microfluidic systems.