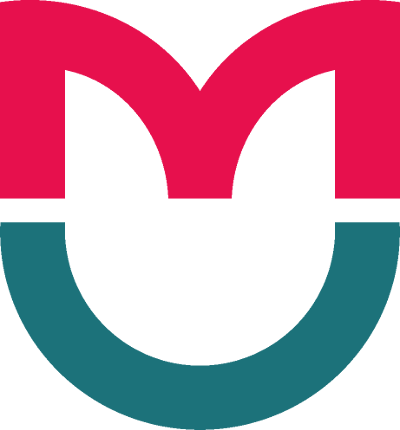
This article is an open access article distributed under the terms and conditions of the Creative Commons Attribution license (CC BY).
ORIGINAL RESEARCH
Alterations in tanycytes and related cell populations of arcuate nucleus in streptozotocin-induced Alzheimer disease model
Research Center of Neurology, Moscow, Russia
Correspondence should be addressed: Dmitry N. Voronkov
per. Obukha, 5, Moscow, 105064; ur.ygoloruen@voknorov
Author contribution: Voronkov DN — immunohistochemical study, morphometric study, data analysis and interpretation, manuscript writing; Stavrovskaya AV — study planning, stereotactic surgery, data analysis and interpretation, manuscript writing and editing; Gushchina AS, Olshanskiy AS — stereotactic surgery, specimens preparation for morphological study.
Compliance with ethical standards: the study was approved by the local Ethics Committee (protocol № 2–5/19 dated February 20, 2019). The animals were manipulated in accordance with the requirements of the European Convention for the Protection of Vertebral Animals Used for Experimental and Other Scientific Purposes (CETS № 170) and the Council of the European Communities Directive 2010/63/EU, order of the Ministry of Health of the Russian Federation № 119Н “On Approval of Rules of Good Laboratory Practice” dated April 1, and GOST 33216-2014 “Rules for Working with Laboratory Rodents and Rabbits”.
Streptozotocin (STZ), the glucosamine-nitrosourea derivative, is an alkylating agent, which causes DNA damage resulting in reduced proliferative activity and cell death. By virtue of chemical similarity with glucose, STZ has a high affinity for glucose transporter (GLUT2). It is selectively captured by pancreatic islet β-cells, which is used for pancreatic cancer therapy and, in case of systemic administration, to model diabetes mellitus in experimental animals [1]. The study, involving the use of pancreatic islet cells, showed that streptozocin caused DNA breaks and activation of poly(ADPribose) polymerases (PARP), which in turn resulted in decreased levels of NAD (being the substrate for PARP) and cell death [2].
Single intracerebral injection of STZ causes neurodegeneration. It is assumed that STZ reduces local glucose metabolism and brain energy metabolism [3–5]. Intracerebroventricular injection of STZ results in progressive death of neurons in hippocampus and neocortex, white matter damage, and cholinergic system dysfunction, causing cognitive impairment in animals [3]. STZ-induced neurochemical changes are as follows: β-amyloid accumulation, tau hyperphosphorylation, and dysfunction of insulin receptors [6–8]. These effects of intracerebral STZ injection reproduce the features of sporadic Alzheimer disease (AD) and are in line with the “type 3 diabetes” hypothesis, which links neurodegeneration in AD and local insulin resistance [9–11]. However, the precise mechanisms of STZ effects on the brain have not yet been well explored, and the hypothesis of STZ-induced direct irreversible insulin receptor desensitization is criticized by some researchers [5].
It is assumed that dysregulation of hypothalamic systems, involved in maintaining energy homeostasis, is one of the causes of AD. Neuroimaging studies have revealed changes in hypothalamic nuclei of patients with AD. According to some sources, insulin resistance and diabetes increase the risk of AD [12]. However, pathologic changes in hypothalamic cell populations in the streptozotocin-induced AD model have not been thoroughly explored. In particular, alterations in tanycytes of the wall of the third ventricle are of interest. Tanycytes are chemosensitive glial ependymal cells. There are several tanycyte subtypes, α1, α2, β1, β2 [13], varying in neurochemical profiles, localization in the wall of the third ventricle, and connections with hypothalamic nuclei. Tanycytes are involved in homeostatic regulation, and serve as sensors of glucose, fatty acids and other nutrients, as well as of the hormones leptin, ghrelin and insulin, involved in regulation of metabolic processes [14, 15]. The effects of targeted tanycyte injury on obesity have been described, the role of tanycytes in the control of eating behavior and association of those with orexigenic neurons of the arcuate nucleus have been demonstrated [16, 17]. Tanycyte involvement in systemic regulation of energy metabolism suggests that dysfunction of tanycytes could be one of the components of pathogenesis of both AD and type 2 diabetes mellitus [15, 18]. There is no detailed description of the tanycyte response to intracerebroventricular injection of STZ. Specification of changes in hypothalamic structures in this popular AD model would help us to move towards understanding the contribution of damage to hypothalamic cell populations to the pathogenetic processes in AD and type 2 diabetes mellitus.
The study was aimed to assess the dynamic changes in the of hypothalamic tanycytes in the streptozotocin-induced Alzheimer disease model.
METHODS
Animals
The study involved 20 Wistar rats (males, 320–350 g, age 3 months by the beginning of the experiment) kept in the vivarium with constant access to food and water. The animals (n = 16) received intracerebroventricular (ICV) injections of STZ (Abcam; UK), and the control group (n = 4) received 0.9% NaCl solution. After administration of the toxin, the animals, which received STZ, were randomly divided into four groups (four animals per group) and withdrawn from the experiment by decapitation using the guillotine (OpenScience; Russia) 2 weeks, 4 weeks, 3 months and 6 months after injection.
Stereotactic surgery
STZ was dissolved in 0.9% NaCl in a dose of 3 mg/kg, 5 μL of the solution were injected into each lateral ventricle using the stereotaxic manipulator (Stoelting; USA). The following coordinates were used for injection: AP = –0.8; L = 1.5; V = 3.5 (in accordance with Paxinos G, Watson C, “The Rat Brain in Stereotaxic Coordinates”). The combination of tiletamine/ zolazepam in a dose of 3 mg/100 g, and intramuscular injection of the 3 mg/kg xylazine dose were used for anesthesia; the animals were premedicated with 0.04 mg/kg dose atropine administered by subcutaneous injection 10–15 min before xylazine hydrochloride administration.
Immunohistochemical analysis
For immunohistochemical study, brain specimens were fixed for 24 h in 4% formalin, and impregnated with 30% sucrose. Then the freezed frontal sections of the hypothalamus, 10 μm thick, were prepared. Antigen unmasking was performed by heating the sections with citrate buffer (antigen retrieval buffer, pH = 6.0; Sigma; Germany) in the steamer for 20 min. The study involved the use of mouse monoclonal antibodies (Abcam; UK) against tanycyte markers, nestin (Nes) and vimentin (Vim), and rabbit polyclonal antibodies (Sigma; Germany) against astroglial proteins, glial fibrillary acidic protein (GFAP) and glutamine synthetase (GS). Neurons were identified using mouse antibodies (Invitrogen; USA) against the RNA-binding protein HuC/D. Mitochondrial alterations were assessed using the rabbit antibodies (Invitrogen; USA) against PGC1α (transcriptional coactivator, regulator of mitochondrial biogenesis) and MT-CO1 (cytochrome C oxidase subunit I, mitochondrial respiratory chain complex IV). Cell proliferation was evaluated using the rabbit anti-Ki67 antibody (Dako; Denmark), and β-amyloid deposits were identified using the rabbit anti-β-amyloid 1–42 antibody (Sigma; Germany). The sections were incubated with primary antibodies for 24 h at room temperature; antibodies were diluted in accordance with the manufacturer's guidelines. Binding was visualized with the use of appropriate pairs of anti-mouse or anti-rabbit IgG secondary antibodies, conjugated with Alexa 488 or Alexa 555 fluorochromes (Invitrogen; USA). The slides were mounted with FluoroShield mounting medium (Abcam; UK), containing diamidino phenylindole (DAPI) for cell nuclei labeling.
Morphometric study and statistical data processing
The slides were examined using the Nikon Eclipse NiU fluorescence microscope (Nikon; Japan) with installed Nikon DS-Qi camera (Nikon; Japan) and NIS Elements software
Д. Н. Воронков с соавт. | DOI: 10.24075/vrgmu.2021.050 2 из 9
(Nikon; Japan). The measurements were performed on the 12bit images acquired using the same settings of the microscope illumination system with the 20x or 40x lens magnification. Areas of interest (lumen of the ventricle, cell nucleus or soma) were manually selected on the images using the graphic tablet (Wacom; Japan). Cell density and immunofluorescent stain intensity in the areas of interest were assessed in at least 25 fields of view in a series of 6–8 frontal slices acquired at various levels of hypothalamus (the results were presented as % of maximum brightness level — 4096 shades of gray). A total of 150–300 cells per animal were selected in the images in order to assess the intensity of MT-CO1 and PGC1α immunofluorescent staining. The data acquired for one animal were averaged. The data acquired for the group were presented as median and interquartile range (Me [LQ; HQ]). Statistical analysis was carried out using the Statistica 12.0 (StatSoft; USA) and GraphPad Prism (GraphPad Software; USA) software. The differences between groups were evaluated with the nonparametric Kruskal Wallis test and subsequent Dunn's test for paired intergroup comparisons. The differences were considered significant when p < 0.05.
RESULTS
Intracerebroventricular injection of STZ resulted in the increasing damage to the wall of the third ventricle and hypothalamic structures. Macroanatomical study revealed the dilated third ventricle (fig. 1A, D): in the second week after STZ administration the area of the third ventricle on the slice was significantly (p < 0.001) larger compared to controls (more than twice larger), and continued to further increase. By month 6 after administration, the area of the third ventricle on the slice increased by more than 20 times, and necrosis was revealed in the adjacent hypothalamic nuclei. Simulation of pathomorphological findings, characteristic of Alzheimer disease, upon STZ administration was confirmed by accumilation of β-amyloid peptide 1–42 in hypothalamic structures (fig. 1B).
When detecting vimentin, damage to glioependymal elements of the wall of the third ventricle was observed (fig. 1А). By week 4 after STZ administration, staining intensity in the zone of α1 and α2 tanycyte (forming connections with dorsomedial and ventromedial hypothalamic nuclei respectively) localization significantly decreased (p = 0.003) compared to controls. The decrease in vimentin staining intensity in the zone of β1 tanycyte (projections to the arcuate nucleus) localization wass less prominent, and significant changes (p < 0.001) were detected only three months after STZ administration. Ependymocytes of the dorsal region of the wall of the third ventricle remained intact until week 4 after administration of STZ. Distinct Vim+ tanycytes of the hypothalamic median eminence persisted after three months, while there were found no Vim+ ependymal cells in other regions of the wall of the third ventricle by month 6. Our study was focused mostly on assessing the changes in the wall of the third ventricle 2 and 4 weeks after STZ administration due to rapidly increasing damage to tanycytes.
Identification of both nestin and vimentin made it possible to demonstrate changes in the morphology of tanycytes. Visually, during week 2 after STZ administration, there were thickened Vim+ processes and deformation of the ventricular wall ependymal layer in the zone of β1 tanycyte localization and the tranzition zone (β1/α2 tanycytes) (fig. 2A). Similar changes were found when detecting nestin (fig. 2А). The nestin staining intensity decreased by week 4 after STZ administration compared to controls (p = 0.024), along with the Nes+ β1 tanycyte density (p < 0.001) (fig. 2C, D). However, the number of Ki-67-positive nuclei in tanycytes significantly decreased (p = 0.049; fig. 2D) by week 2, which confirmed the effects of STZ on the proliferative activity of cells.
The damage to tanycytes was preceded by altered expression of proteins, associated with mitochondrial functions. Reduced intensity of tanycyte cytoplasm staining with antiMTCO1 (mitochondrial respiratory chain complex IV) antibody compared to controls (p = 0.013) was observed starting from week 2 after STZ administration, earlier than the reduction of tanycyte density and the decrease in nestin expression (fig. 3A, B). Furthermore, MT-CO1 staining in cell bodies of the adjacent arcuate nucleus persisted, and counting neurons of the arcuate nucleus during weeks 2 and 4 after STZ administration revealed no significant decrease in the neuronal density compared to controls. Detection of transcriptional coactivator PGC1a (involved in regulation of a number of mitichondrial functions) revealed the significantly (p = 0.048) increased intensity of tanycyte nuclei staining by week 2 (fig. 3B, C), while during week 4 after STZ administration, PGC1a staining intensity dropped to control values.
Immunohistochemical identification of GFAP and glutamine synthetase (GS) was used to assess the astroglial changes. In intact animals, GFAP was detected in the substantial proportion of ependymal cells, however, GS expression levels in tanycytes appeared to be negligible compared to astroglia. Changes in the morphology of the GFAP-positive astrocytes in the arquate nucleus had been already indicative of the STZ-induced reactive gliosis by week 2: thickening, deformation of processes and increased staining for glial cell markers were observed (fig. 4А, B). The GS staining intensity increased by week 4 (p = 0.03), which was probably due to the increased area of astrocytes on the slice (fig. 4B, D). However, the number (density) of astrocytes did not increase early after STZ administration and significantly decreased compared to controls by month 3 (p = 0.019; fig. 4C). In general, changes in astroglia were indicative of the increasing neuroinflammatory response in hypothalamic structures adjacent to the third ventricle. Although STZ caused damage to astrocytes, these cells appeared to be more resistant to the toxin compared to tanycytes.
To summarize the findings, it can be noted that STZ administration resulted in β-amyloid accumulation in hypothalamic structures, including neurons of arcuate nucleus, and in ventricular enlargement, which was probably due to the damage to glial ependymal elements. Tanycytes of various types were susceptible to STZ, however, both α1/α2 tanycytes and β1 tanycytes were damaged early after STZ administration, as manifested in reduced proliferative activity and altered expression of proteins, associated with mitochondria. These alterations occurred before the detection of the prominent tanycyte morphology impairment. The β1 tanycyte density in the third ventricle decreased by week 4 after STZ administration. Moreover, STZ caused reactive changes in astrocytes and damage to astroglia, however, there were no changes in the density of both astrocytes and neurons of arcuate nucleus within four weeks after administration of the toxin, which was indicative of higher tanycyte vulnerability to STZ compared to other hypothalamic cell populations.
DISCUSSION
The majority of papers on studying morphological and neurochemical changes in streptozotocin-induced models are focused on hippocampal research due to the role of hippocampus in cognitive impairment, associated with AD. These data are in line with alterations, revealed during our study.
Thus, experiments, involving intraventricular or intracisternal STZ administration, demonstrate β-amyloid accumulation in hippocampus three months after administration of the toxin, altered expression of synaptic proteins, as well as neuronal cell death and reduced hippocampal volume [3, 19]. The effects of STZ on the hippocampal astrocytes have been identified: the reduced number and altered structure of the astrocyte processes have been demonstrated [20]. Reduced hippocampal expression of insulin receptors upon administration of STZ has been found [21]. Since astrocytes are believed to be the target cells for insulin, and the knockout of astrocytic insulin receptors leads to cognitive impairment in animals [22], it can be assumed that dysfunction in glial components plays a vital part in STZ-induced localized metabolic disorders.
No data on tanycyte alterations in humans with AD have been presented in the literature. Only the age-related changes in the tanycyte processes' organization have been described [23]. However, degenerative changes, β-amyloid accumulation and mitochondrial dysfunction in hypothalamic nuclei are found during pathological studies [24].
The mechanisms, underlying hypothalamic dysfunction in humans with AD, are poorly understood. Apart from neurodegeneration, many researchers assign an important role to alterations in synthesis, secretion and transport of hypothalamic hormones and factors [18, 24], involved in homeostatic regulation of hypothalamic-pituitary axis, which could be mediated by dysfunction of tanycytes. For example, tanycytes are involved in regulation of the thyroliberin release and serve as a main source of triiodothyronine in the brain. Furthermore, neuroprotective effects of both thyroliberin and triiodothyronine have been demonstrated in AD models [25, 26].
Tanycytes express the GLUT2 transporter [13, 27] and are therefore one of the target cells for STZ; the damage to tanycytes, revealed during our study, is the intended effect of the toxin administration. The cause of the more prominent damage to α tanycytes, observed during our study, may be associated with uneven pattern of glucose transporter expression and the frequency of various tanycyte populations renewal, as well as with tanycyte metabolic features, which should be further clarified. The differences seen in dynamic changes in nestin and vimentin levels can be explained by the fact that the content of vimentin in α and β tanycytes is different, although all types of tanycytes express these proteins [18]. Moreover, there have been reports of multidirectional changes in regulation of cytoskeletal proteins in tanycytes [28].
It is important that the STZ-induced changes in tanycytes preceded other hypothalamic changes, associated with neurodegeneration and neuroinflammation. In general, considering the fact that tanycytes modulate neurons of ventromedial, dorsomedial and arcuate hypothalamic nuclei, and are involved in regulation of both local and systemic energy metabolism, as well as in regulation of eating behavior [15, 18, 29], the damage to tanycytes is likely to result in the carbohydrate metabolism disorders, observed in this AD model [30, 31]. However, hypothalamic dysfunction upon STZ administration and the previously reported hypothalamic neurodegeneration during the final stages [31] may be triggered by neuroinflammatory response, involving tanycytes and other cell populations.
The decreased intensity of tanycyte staining for MTCO1 (cytochrome C oxidase subunit I) we have identified is consistent with the decreased cytochrome C oxidase activity in streptozotocin-induced diabetes model [32]. Studying brain cortex and hippocampus in animals, which received STZ, revealed reduced activity of pyruvate dehydrogenase, α-ketoglutarate dehydrogenase, and cytochrome C oxidase in mitochondria of both neocortex and hippocampus [33]. Moreover, the reduced activity of mitochondrial complexes I‒ III upon intracerebroventricular injection of STZ was revealed [34]. These data emphasize mitochondrial sensitivity to STZ, previously studied in vitro [35]. The increased expression of PGC1a (regulator of mitochondrial biogenesis), observed early after STZ administration, could be considered a compensatory response to the developing disorders. The return to baseline PGC1a levels could be indicative of partial function recovery in the intact tanycytes. In turn, PGC1a dysregulation is associated with neuroinflammatory processes and could be considered one of the factors, contributing to neuronal damage in AD [36, 37]. The STZ-induced dynamic changes in mitochondrial dysfunction, observed in various structures, have yet to be assessed.
It would be interesting to juxtapose the observed STZinduced decrease in tanycyte proliferation with the previously reported effects of this toxin on the hippocampal neural stem cells [38], as well as with its high toxicity in immature neurons [39]. Since tanycytes are likely to have neurogenic potential [40], the STZ-induced impairment of proliferation and apoptosis in hypothalamic neural stem cells can exert the long-term effects, associated with impaired plasticity of hypothalamic neural networks, involved in homeostatic control.
Fig. 5 (fig. 5) (according to [14, 15, 17, 18]) summarizes some functions of α and β tanycytes, which are suspected of being involved in pathological changes in the streptozotocininduced AD models. The potential tanycyte involvement in the pathogenesis of AD suggests the possibility of developing the pharmacological methods for the tanycyte function correction. Some authors believe that the tanycyte proliferation regulation via exposure to growth factors could be provided [18].
CONCLUSIONS
The findings demonstrate increased sensitivity of hypothalamic tanycytes to STZ, reduced tanycyte proliferative activity and the associated mitochondrial alterations. Astrocyte activation and neuronal damage are preceded by alterations in tanycytes. The changes, observed in the mediobasal hypothalamus, suggest that the damage to tanycytes plays a vital part in local and systemic metabolic disorders occurring in the Alzheimer disease models. The possible functional consequences of damage to tanycytes are as follows: impaired barrier function and downregulated cellular metabolism in hypothalamic structures, unbalanced orexigenic and anorexigenic effects, dysregulation of the hypothalamic-pituitary-thyroid axis.