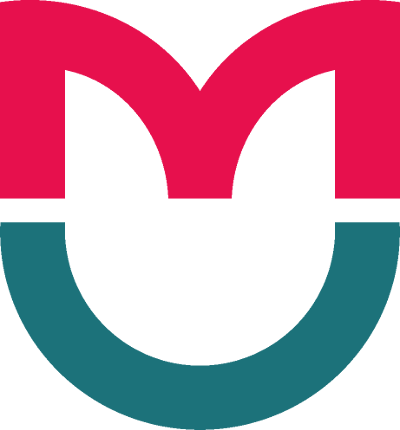
This article is an open access article distributed under the terms and conditions of the Creative Commons Attribution license (CC BY).
ORIGINAL RESEARCH
Systemic inflammation markers of diet-induced metabolic syndrome in rat model
Siberian State Medical University, Tomsk, Russia
Correspondence should be addressed: Julia G. Birulina
Moskovsky Trakt, 2, str. 7, Tomsk, 634050, Russia; ur.xednay@02anilurib
Funding: the study was supported by the Russian Science Foundation, Grant № 22-25-20039, https://rscf.ru/project/22-25-20039/ and funds of the Tomsk Region Administration.
Author contribution: Birulina JG, Voronkova OV — concept and design, manuscript writing; Ivanov VV, Buyko EE — metabolic syndrome modeling, biochemical blood tests; Shcherbakova MM — blood cytokine assay; Chernyshov NA — literature analysis, hemogram tests; Motlokhova EA — statistical analysis.
Compliance with ethical standards: the study was approved by Ethical Review Board at SibSMU (Protocol № 8201 of 27 March 2020) and carried out in compliance with humanity principles stated in the 86/609 EEC Directive and the Declaration of Helsinki.
Chronic systemic inflammation is essential in many multifactorial non-infectious diseases, including such alimentary-dependent conditions as type 2 diabetes, obesity and metabolic syndrome (MS). The low-grade chronic inflammation of adipose tissue is a key contributor to the insulin resistance development [1]. Metabolic impairments (hyperglycemia, hyperinsulinemia, dyslipidemia) confer pro-inflammatory properties to cellular elements in adipose tissue, with macrophage functionalities switching from anti-inflammatory M2 to pro-inflammatory M1. Together with hypertrophied adipose cells, the M1-polarized resident macrophages produce vast quantities of adipokines (leptin, resistin, etc.), pro-inflammatory cytokines (IL6, TNFα, IL1β, etc.) and chemokines (MCP-1, MIF, CCL-2, etc.), which stimulate infiltration of adipose tissue with recruited monocytic macrophages arriving from circulation [1, 2] thus supporting perpetuation of the inflammatory process and potentiating insulin resistance.
Although subclinical chronic inflammation in patients with MS is a well-known fact, no clinical-laboratory criteria for the basic phenomena of chronic systemic inflammation, (including subtypes, stages, compensatory reactions, maladaptation and damage) have been established. Experimental animal models provide an essential tool for the analysis of early and delayed manifestations of systemic inflammatory reactions as well as their specific mechanisms in diseases that affect multiple parameters of homeostasis [3].
One of the common principles used for in vivo modeling of the obesity-associated MS involves combined high-fat, high-carbohydrate (HFHC) diets fed ad libitum [4, 5]. Such diets provide sustainable models of metabolic impairments (insulin resistance, hyperglycemia, dyslipidemia, etc.) etiopathogenetically similar to alimentary-constitutional obesity in humans. At the same time, it has been noted that in only 60–80% of animal models the diet-induced obesity eventually conveys a spectrum of metabolic impairments fully corresponding to MS [6, 7]. Accordingly, MS modeling should involve identification and analysis of additional parameters potentially useful as valid biomarkers of pathogenesis in MS and associated conditions including chronic systemic inflammation.
This study aimed at characterization of systemic inflammatory reaction as a component of diet-induced MS in rat model.
METHODS
Experimental MS was modeled in male Wistar rats (33 animals, body weight 280.5 × 36.1 g, age 6 weeks at the start of the experiment). The rats obtained from Goldberg Research Institute of Pharmacology and Regenerative Medicine (Tomsk, Russia) and randomized into control and experimental groups (15 and 18 animals, respectively) were housed in polypropylene cages (1612 cm2 floor area), three individuals per cage at 20–26 °С, 40–70% relative humidity and 12:12 light cycle. The animals were included in the study based on the following criteria: lack of explicit health problems; body weight deviation from the average value within 10%. The control group animals received a standard 24 : 6 : 44% protein-fat-carbohydrate diet (Delta Feeds chow; BioPro, Russia) with ad libitum access to food and water. The experimental (MS) group animals received a HFHC diet of the standard chow (66%) mixed with rendered lard (17%), fructose (17%) and cholesterol (0.25%; Sigma, USA) to a 16 : 21 : 54% protein-fat-carbohydrate ratio and replacement of drinking water with a 20% fructose solution. The experiment lasted 12 weeks.
Consistency of the model with established criteria for MS was tested as described previously [5, 8]. The recorded parameters were as follows: systemic arterial pressure ("Systola" system for non-invasive measurement of blood pressure in rodents; Neurobotics, Russia), body weight (Pioneer PX224 analytical balance; OHAUS, China), weight fractions of visceral adipose tissue and the liver (g per 100 g body weight) and biochemical indicators including serum glucose fasting, oral glucose tolerance test by spectrophotometry using enzymatic kits (Chronolab; Spain), triacylglycerol (TAG), total cholesterol (TC) and low-density (LDL-C), very low-density (VLDL-C) and high-density (HDL-C) lipoproteins levels (Architect с4000 clinical chemistry analyzer; Abbot, USA).
The animals were withdrawn from the experiment by СО2 asphyxia. The blood was collected from the heart into two test tubes: with a clotting activator for serum production and subsequent biochemical and enzyme immunoassay and with an anticoagulant for WBC quantitation.
The inflammatory process intensity was assessed by total and differential white blood cell (WBC) quantitation using BC2800 Vet automatic hematology analyzer (Mindray; China), Bradford total protein assay and high-sensitivity C-reactive protein test (Rat CRP ELISA Kit; Elabscience Biotechnology, China).
Immunosorbent assay was used to assess serum levels of IL6, IL10 and TNFα (corresponding kits by Bender MedSystems GmbH, Austria), insulin (Insulin Rat ELISA Kit; Thermo Fisher Scientifiс, USA) and leptin (Leptin Rat ELISA Kit; Thermo Fisher Scientific, USA). The homeostatic model for assessing insulin resistance (HOMA-IR) was calculated by the formula:
[serum insulin] × [serum glucose] / 22.5
The intensity of local inflammatory process in fat tissue was accessed through reactive oxygen species (ROS) production rate measured with the 2,3-dihydrodichlorofluorescein diacetate (DHCF-DA) assay, by incubation of 50 mg fragments of visceral fat tissue in 10 µM DHCF-DA for 60 min. The fluorescence was measured at an excitation wavelength of 530 nm and an emission wavelength of 485 nm using a microplate reader (Infinite 200 Pro M-plex; Tecan, Switzerland) [9].
Statistical analysis was carried out in SPSS Statistics 23 (IBM; USA). Data complying with the normal distribution law are presented as means and standard deviations, M ± SD, non-complying — as medians and interquartile ranges, Me (Q25; Q75). Between-the-group comparisons were made by Student's t-test or Mann–Whitney U test. The differences were considered significant at p < 0.05. Statistical relationships between quantitative indicators were assessed by calculating Spearman rank correlation coefficient or Pearson correlation coefficient for pairs.
RESULTS
The 12-week HFHC diet promoted physiological and laboratory manifestations of metabolic impairments in animals of experimental group (MS). The analysis of morphometric/physiological/ biochemical indicators revealed arterial hypertension, increased body weight and increased relative weights of the liver and visceral adipose tissue in animals with modeled MS compared to control group. Biochemical tests revealed impaired glucose tolerance (increased area under curve for glucose concentration on time, AUC0–120), fasting hyperglycemia and altered lipid spectrum indicators including increased serum levels of TAG, TC, LDL-C and VLDL-C specifically in animals with modeled MS (tab. 1). These findings characterize the model as consistent and suitable for the analysis of accessory criteria in MS.
Serum insulin levels were used as insulin resistance indicator in experimental MS. In animals with modeled MS, serum insulin levels were increased more than two-fold compared with control group, and HOMA-IR was 1.3 × 0.4. Animals with modeled MS also presented with elevated serum levels of the adipose tissue hormone leptin, which exceeded control values 1.5-fold on average (tab. 1).
The analysis of free radical oxidation rates in visceral fat tissue revealed ROS production increased significantly to 2.5 conventional units — more than two-fold compared with control group (1.2 conventional units, р = 0.008).
Biochemical tests revealed serum levels of total protein and C-reactive protein elevated, respectively, 1.3-fold and 2.6-fold in experimental MS compared with control values (tab. 1).
The analysis also revealed significant increase in total WBC counts, as well as total and differential blood granulocyte counts, in animals with experimental MS (tab. 2).
Serum levels of IL10 and TNFα in rats with HFHC dietinduced metabolic impairments exceeded the corresponding control values, respectively, 3.7- and 4.2-fold on average (tab. 3). A similar trend observed for IL6 proved statistically non-sigificant.
DISCUSSION
Two basic methodologies for MS modeling in small rodents have been established in experimental medical biology: (1) animal strains genetically predisposed to diabetes/obesity/ cardiovascular diseases and (2) imbalanced diets with modified nutrient ratio (rich in fats and sugars) [4, 10]. Both approaches result in the excessive accumulation of visceral fat as a key pathogenic factor mediating the profound metabolic impairments.
Despite the widespread use of HFHC diets as a basis for MS modeling, certain methodological complications have been recognized as hampering verification of the efficacy. For example, the developing visceral obesity cannot be monitored in medical terms, as routine biometric screenings (body mass indexing, waist measurements, etc.) are not extrapolatable to rodents. Several studies demonstrate that such hallmarks of MS as hypertension, dyslipoproteinemia and hyperglycemia are only partly reproducible in animals [11, 12]. In some cases, these limitations are due to insufficient duration of the diet, as well as age- and sex-related differences, genetic distinctions of particular strains of experimental animals, systematic errors in nutrition formula/regimen, etc.
The rates of metabolic activity of fat tissue can provide useful indicators for MS verification in laboratory animals. The leptin/ghrelin ratio has been shown to correlate with changes in body, splenic and fat weights in Wistar rats with experimental dyslipidemia and aletred levels of inflammation-regulating cytokines (MCP-1, M-CSF, IL18 and RANTES) which identifies these criteria as prospective biomarkers of direction and severity of metabolic impairments in obesity and MS [13].
Chronic inflammation of fat tissue, a strong pathogenetic factor in MS, develops on the background of insulin resistance coupled to hyperglycemia and dyslipoproteinemia [1, 2]. The highly active signaling substances (adipokines, proinflammatory cytokines) produced by adipose and stromal cells facilitate macrophage infiltration thus supporting the maintenance of local inflammatory response [14].
Our analysis of metabolic activity of cellular elements in the visceral fat of rats after a 12-week HFHC diet reveals augmentation of free-radical reactions with a 2-fold increase in the spontaneous ROS production levels compared with control group.
The high secretory activity of adipose cells in animals with modeled MS is also indicated by elevated blood levels of leptin showing an average 1.5-fold increase compared with control group (tab. 1). At that, serum levels of leptin and insulin revealed a moderate positive correlation (r = 0.701; р = 0.001).
Leptin is predominantly produced by adipose cells of white fat tissue, and its blood levels correlate with the visceral fat volume. Under physiological conditions, leptin inhibits insulin production by the pancreas [15]. The MS-associated hyperleptinemia observed by us in this study is most probably of compensatory nature, reflecting insulin resistance and the elevated blood levels of insulin. Incidentally, such metabolic impairments as hyperglycemia and dyslipoproteinemia have been associated with decreased expression of leptin receptors on cells, promoting resistance [16, 17].
Based on its structure, leptin can be classified as proinflammatory adipokine. Leptin participates in chemotaxis regulation and neutrophil activation, T cell differentiation and NK cell pool maintenance. At the humoral level, leptin stimulates production of TNFα and IL6. Increased secretion of leptin by adipose cells attracts macrophages and facilitates their transfer to adipose tissue by stimulating angiogenesis [18].
The metabolic conditions relevant for activation of cellular elements in fat tissue (notably the levels of glucose, insulin and fatty acids) have been shown to determine its cytokine repertoire. For example, high concentrations of free fatty acids can activate TLR4 receptors on macrophages, indirectly triggering expression of genes responsible for the inflammatory mediator synthesis [2, 14]. This leads to formation of the 'vicious circle' in the adipose tissue inflammation pathogenesis, as systemic effects of certain pro-inflammatory cytokines include fatty acid synthesis activation and increase in their blood levels through suppression of adiponectin secretion and regulation of production for other cytokines.
In this study, we recorded a statistically significant increase in serum levels of TNFα and IL10 in rats with experimentally induced MS compared to control animals (tab. 3).
At early stages of inflammation, the balance between defensive-adaptive and pathological inflammatory reactions is ensured by anti-inflammatory cytokines (IL10, IL13, TGFβ, etc.), which limit tissue damage by inhibiting expression of major histocompatibility complex class II and other costimulatory molecules on immunocompetent cells [19]. This probably explains the elevated serum levels of anti-inflammatory IL10 in experimental group animals with MS against the background of metabolic impairments. Such inference is indirectly supported by a moderate positive correlation between IL10 and leptin levels (r = 0.523; р = 0.012).
Of note, progression of MS and obesity may be accompanied by a decrease in production of anti-inflammatory cytokines and hormones (ghrelin, adiponectin) by fat tissue, which enhances the mononuclear cell infiltration of fat tissue and aggravates the inflammation [20, 21]. As a rule, this is a low-grade inflammation, given that the anti-inflammatory resistance factors are capable of prolonged deterrence of the generalized process and prevent the deployment of secondary damage against the background of chronic systemic alterations. Accordingly, compared with acute inflammatory conditions, chronic inflammation is accompanied by modest shifts in systemic indicators.
In this study we assessed the dynamics of systemic manifestations of the inflammatory process confined to visceral fat tissue. Apart from the altered cytokine levels, animals with MS showed significant increases in total WBC and differential granulocyte counts, as well as serum levels of total and C-reactive protein compared to control animals.
Many inflammatory conditions are accompanied by elevated production of acute phase proteins, chiefly by hepatocytes. Under pathological conditions, white adipose cells are also capable of producing high amounts of C-reactive protein, which exacerbates the glucose metabolism impairment and aggravates insulin resistance [22, 23].
Reactive leukocytosis can be attributed to particular components in MS pathogenesis. High-fat diets have been shown to induce myeloid hyperplasia in animal models, especially with regard to neutrophil cellular patterns [24]. Several studies enrolling patients with glucose intolerance and obesity revealed a positive correlation between antropometric/ physiological/biochemical indicators (body mass index, arterial hypertension, hyperinsulinemia, dyslipoproteinemia) and increased WBC counts [25–27]. A possible role of leptin in MS-associated leukocytosis has been highlighted: leptin and its receptors have been implicated in hemopoiesis-stimulating signaling in the red bone marrow [25].
Glycylation end-products, ROS and pro-inflammatory cytokines exert similar effects on hemopoietic cells and mature leukocytes. Pro-inflammatory cytokines are important inducers of leukocytosis, particularly neutrophilic, by multiple mechanisms including demargination of intravascular neutrophils and facilitation of granulocytopoiesis and neutrophil exit in the bone marrow [28, 29].
CONCLUSIONS
The experiment revealed signs of systemic inflammation, including reactive leukocytosis, hyperproteinemia and elevated serum levels of C-reactive protein and cytokines TNFα and IL10, alongside the visceral obesity and impaired lipid/carbohydrate metabolism (hyperinsulinemia, hyperglycemia, dyslipoproteinemia) in rats with modeled MS. The observed immunological changes were accompanied by increased metabolic activity of visceral fat tissue, indicated by hyperleptinemia and increased free radical oxidation rates. Serum levels of leptin positively correlated with serum levels of both insulin and IL10. Thus, apart from metabolic impairmentrs, the 12-week experimental exposure to a HFHC diet reproducibly modeled the early signs of systemic inflammation characteristic of MS and obesity. Such diet-induced MS models can be considered a convenient tool for studying the early and delayed MS complications mediated by pathogenetic factors of chronic systemic inflammation.