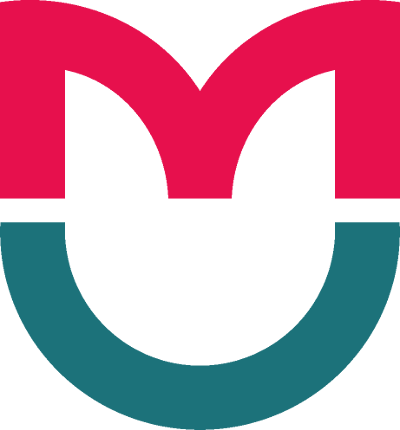
ORIGINAL RESEARCH
Peculiarities of amino acid profile in monocytes in breast cancer
1 Kulakov National Medical Research Center for Obstetrics, Gynecology and Perinatology, Moscow, Russia
2 Cancer Research Institute, Tomsk National Research Medical Center, Tomsk, Russia
3 Tomsk National State University, Tomsk, Russia
4 Siberian State Medical University, Tomsk, Russia
5 Institute of Transfusion Medicine and Immunology, Faculty of Medicine Mannheim, University of Heidelberg, Heidelberg, Germany
6 German Red Cross Blood Service Baden-Württemberg-Hesse, Mannheim, Germany
Correspondence should be addressed: Anastasia V. Novoselova
Academik Oparin street, 4, Moscow, 117198, Russia; moc.liamg@hciveknarfv
Funding: The study was financially supported by the Russian Federation represented by the Ministry of Science and Higher Education of the Russian Federation (agreement dated 29 September 2021 № 075-15-2021-1073 on the topic "Genetic and epigenetic editing of tumor cells and the microenvironment in order to block metastasis ").
Author contribution: Novoselova АV — material processing, monocyte amino acid profile analysis, statistical data processing, text editing; Yushina MN — material processing, text writing and editing; Patysheva MR — study concept and design, monocyte isolation; Prostakishina EA — monocyte isolation; Bragina OD, Garbukov EY — patient selection, collection of biological material; Kzhyshkowska JG — study concept and design.
Compliance with ethical standards: the study is approved by the ethics committee of the Research Institute of Oncology under Tomsk National Research Medical Center (record No.10 dated 05 December 2019), was conducted in accordance with the standards of the ethics committee of V.I. Kulakov National Medical Research Center for Obstetrics, Gynecology and Perinatology, federal laws of the Russian Federation (Nos. 152, 323, etc.) and the 1964 Declaration of Helsinki with all subsequent additions and amendments regulating scientific research on biomaterials obtained from humans. All participants signed an informed consent to participate in the study.
Monocytes in the adult body are a dynamic population of the main cells of innate immunity, which are permanently formed from precursors in the bone marrow and circulate in the bloodstream for 3 to 6 days. Monocytes in the adult organism serve as major precursors of tissue macrophages, major sensors of innate immunity for exogenous and endogenous pathological factors from the blood, and key precursors of tumor-associated macrophages [1–5]. Representing a pool of effector cells of innate immunity with pattern-recognizing receptors, they can act as regulators of tumor growth. Different types of monocytes have mixed effects on the growth and metastatic spread of cancer cells, they both activate and inhibit these processes. Tumor progression is associated with the triggering of a whole cascade of inflammatory and immune reactions. These pathological processes are associated with changes in the amino acid content of monocytes, which can lead to impaired monocyte function [6–8].
Monocytes are precursors of tumor-associated macrophages (TAMs) and dendritic cells, which form the tumor microenvironment [9]. At the site of inflammation, they perform the function of phagocytosis and the production of pro-inflammatory cytokines. In a pro-inflammatory microenvironment, they differentiate into inflammatory macrophages and inflammatory dendritic cells, which subsequently migrate to lymph nodes and activate CD4+ and CD8+ T-lymphocytes. Activated immune cells are subsequently involved in metabolic pathways associated with cancer cell progression [10–13]. This commonality of metabolic processes creates a fundamental competition for the nutrient substrates required by both cancer and immune cells in the tumor microenvironment.
The development of analytical techniques, primarily liquid chromatography with mass spectrometric detection (LC-MS), has led to the formation of metabolomics [14–16], which is actively used to identify the metabolic features of cancer processes, to clarify the mechanisms of pathogenesis and search for new drug targets [14, 17]. To date, the study of the amino acid profile deserves special attention. Changes in amino acid levels may be important for the formation of a proper immune response [13, 18–20] and may lead to disruption of immune cell migration, division and maturation. Amino acids are involved in the control of a number of pathways that regulate immune cell responses, including mTOR signaling and NO production [19, 21]. Competition for metabolites and signaling interaction between host immune cells and pathogens can affect the development of disease, including tumor genesis [18].
Breast cancer (BC) remains the leading malignant neoplasm in women by prevalence [22]. This is the first type of cancer, for which the role of immune cells in tumor growth maintenance was demonstrated in experiments on animals [23]. Further studies allowed to accumulate information on mutual influence of cancer cells and immune system cells [7, 9, 17, 24–29].
The study of monocyte heterogeneity and its role at different stages of cancer process progression is crucial to investigate the ability of cancer cells to obstruct immunological surveillance and hide from the aggression of immunocompetent cells. Work in this direction will make it possible in the future to identify new biomarkers of the cancer process and develop personalized cancer immunotherapy pathways [30].
The aim of this work was to determine the amino acid profile of monocytes isolated from the blood of BC patients.
METHODS
The study group included 13 patients aged 36–69 years with stage IIA-IIIA T1-2N0-1M0 breast cancer. All patients had Her2+ molecular tumor subtype (Ki-67 score of at least 30%). The control group included 10 healthy volunteers aged 50–67 years. Inclusion criteria for the control group: no acute chronic diseases, no history of cancer, no history of allergies or autoimmune diseases, signed informed consent to participate in the study.
The object of the study was peripheral venous blood taken on an empty stomach in test tubes with K3EDTA. Researchers obtained mononuclear cell fraction from blood by enrichment on a Ficoll density gradient (1.077 g/cm3). CD14+ monocytes were isolated from the obtained mononuclear cell fraction according to the Myltenyi Biotec positive magnetic separation protocol using a MidiMACS magnetic separator (Myltenyi Biotec; Germany). The purity of the obtained fraction was determined by flow cytometry on a Cytoflex (Beckman Coulter; USA) and was more than 92% CD14+ cells. Cell counts were based on flow cytometry data. Researchers separated an aliquot containing 4–5 million monocytes and washed it 3 times by centrifugation at 311 g for 5 min with cold 0.3% ammonium acetate. The resulting cell precipitate was dried in a nitrogen atmosphere and stored at –80 °C until extraction for not more than 6 months.
Sample preparation for LC-MS analysis
The study used acetonitrile, methanol, butanol, and chloroform (purification grades for liquid chromatography) purchased from Merck (Darmstadt; Germany), and a 37% aqueous hydrochloric acid solution (Acros Organics; USA). Deionized water was prepared using the Milli-Q Reference Water Purification System (Molesem; France).
We prepared samples according to the following procedure: add 480 μL of chloroform/methanol (2:1 vol./vol.) solution to the monocyte sample; sonicate the sample for 10 min at room temperature, add 150 μL of water, stir sample for 5 min; centrifuge the resulting solution at 13,000 g for 5 min at room temperature; sample 150 μL of the upper aqueous-methanol layer; dry it in a nitrogen flow for 30 min at 60 °C; add 200 μL of hydrochloric acid solution in butanol (0.1 mol/L); stir it for 3 minutes ; centrifuge it at 13,000 g for 15 s at room temperature; then incubate at 60 °C for 15 min for the derivatization reaction; centrifuge at 13,000 g for 15 s at room temperature; dry it in a nitrogen flow for 30 min at 60 °C; stir for 5 min; centrifuge at 13,000 g for 15 s at room temperature; transfer 200 μL of the resulting sample to a vial with an insert for further analysis.
LC-MS analysis and data processing
We analyzed the samples by high-performance liquid chromatography using a 1260 Infinity II chromatograph (Agilent; USA) with detection by a 6460 Triple Quad mass spectrometer (Agilent; USA). Samples were separated by liquid chromatography using an Agilent Zorbax Eclipse XDB-C18 2.1 × 100 mm column with a sorbent diameter of 1.8 μm (Agilent; USA).
For the analysis of amino acids, we injected 3 μL of the sample and as the mobile phase, we used two eluents, fed at a flow rate of 150 μL/min and maintained a column temperature at 20 °C. Eluent A was 10 mM ammonium acetate solution in water, eluent B was acetonitrile. We changed the composition of the mobile phase during the analysis according to tab. 1.
Transitions between precursor ions and product ions for the analyzed amino acids are shown in tab. 2. Parameters of the ion source were as follows: drying gas temperature — 150 °C, drying gas flow rate — 10 L/min, spray gas pressure — 2.76 bar, curtain gas temperature — 400 °C, curtain gas flow rate — 10 L/min, capillary voltage — 2000 V.
We determined metabolite levels using QuantAnalysis software (Agilent; USA).
Statistical analysis of the data
All data were analyzed in the R software environment (http:// www.r-project.org/, versions R 4.1.1, 4.1.2). For initial processing of data tables we used the packages tidyverse (version 1.3.1) and readxl (1.3.1). The results were visualized using ggplot2 (version 3.3.5) and ggforestplot (version 0.1.0).
Amino acid levels were compared using the nonparametric Wilcoxon-Mann-Whitney test. Medians (Me) and quartiles Q1 and Q3 were used to describe quantitative data. The value of the threshold level of significance p was taken to be 0.05.
The team of researchers developed logistic regression models to assess the possibility of classifying patients into groups on the basis of the parameters under study. Levels of individual amino acids were considered as independent variables in the models. The dependent variable was whether the sample belonged to the 'normal' or BC group. Of all the models developed, we selected the four with the highest area under the ROC curve (AUC). The quality of the models developed was assessed by plotting the ROC curve, determining the area under the ROC curve, and calculating sensitivity and specificity.
If amino acid levels differed in monocytes from those in BC patients, we analyzed the involvement in metabolic pathways using the MetaboAnalyst 5.0 resource (https://www.metaboanalyst.ca/home.xhtml)
RESEARCH
Semi-quantitative data on the content of 26 amino acids in monocytes of healthy donors and in monocytes of BC patients were obtained in the analysis. The list of amino acids included methyl-histidine, aminobutyric acid, 5-hydroxylysine, alanine, arginine, asparagine, aspartic acid, citrulline, cystine, glutamic acid, glutamine, glycine, histidine, leucine, lysine, methionine, ornithine, phenylalanine, proline, sarcosine, serine, threonine, trans-4-hydroxy-proline, tryptophan, tyrosine, valine. We obtained amino acid profile by dividing the chromatographic peak area of each amino acid by the total peak area of all amino acids in the corresponding sample. To search for differences in amino acid profiles in monocytes obtained from "normal" and BC patients, we compared the data obtained by LC-MS using the Wilcoxon-Mann-Whitney method. Significant differences in metabolite levels were found for glycine (p-value = 0.0127), asparagine (p-value = 0.0197), proline (p-value = 0.0159), methionine (p-value = 0.0357), tryptophan (p-value = 0.0028), tyrosine (p-value = 0.0127) (fig. 1).
Where the levels of amino acids differed significantly between monocytes of the "normal" and BC groups, we carried out a bioinformatic analysis of metabolic pathways involving these acids. The analysis was performed using the MetaboAnalyst resource. As a result, biological networks have been identified that could potentially be involved in altering the phenotype of monocytes under the influence of BC. As we analyzed the data presented in the KEGG database and SMPDB (tab. 3, tab. 4), we were able to identify pathways ordered by levels of significance (pathway enrichment analysis — y-axis in fig. 2) and the values of the influence of the metabolites in question on the pathway (pathway topology analysis — x-axis in fig. 2). The color of the node corresponds to the level of significance, and the radius of the node correlates with the amount of influence on the path. The effect on the pathway was calculated as the sum of the significance values of the corresponding metabolites divided by the sum of the significance values of all metabolites in each pathway. Metabolic pathway enrichment was assessed by Over representation analysis (ORA) using a hypergeometric test.
To test the feasibility of diagnosing RBCs by amino acid levels in monocytes, we developed logistic regression models for each of the significant amino acids (fig. 3A). We also considered models using a combination of amino acids and selected the four with the highest area under the operating curve (AUC) by ROC analysis (fig. 3B). tab. 5 shows the results of the quality assessment of the single amino acid models. Models built on a combination of amino acids had AUC = 1 and 100% sensitivity and specificity (fig. 3B).
DISCUSSION
Of the 26 amino acids analyzed, only six amino acids had significant differences in monocyte levels between healthy donors and BC patients. Of these six amino acids, glycine, tryptophan and asparagine are of particular interest because disturbances in the metabolic pathways associated with them are associated with tumor-induced changes [11, 13, 14, 18, 27, 31].
The availability over time of the essential amino acid tryptophan is an important determinant of the strength and quality of the immune response. Proliferation and activation of human T cells were strongly inhibited in tryptophan-free media compared to conventional nutrient media [32, 33]. Cancer cells, TAM and cancer-associated fibroblasts can reduce tryptophan levels through the enzymatic activity of indoleamine-2,3-dioxygenase (IDO) [13]. TAM, and sometimes tumor cells themselves, increase IDO levels and create an immunosuppressive microenvironment via at least two mechanisms: tryptophan depletion and accumulation of tryptophan catabolites such as kynurenine, 3-hydroxyanthranilate and quinolinate [34, 35]. Tryptophan depletion inhibits the proliferation of activated T cells, and tryptophan-derived catabolites act as ligands for aromatic hydrocarbon receptors [36]. Kinurenine is a suppressor of T-cell immunity. By stimulating aromatic hydrocarbon receptors, it directs naïve T cell differentiation towards regulatory T cells and inhibits Th17 cell differentiation [22, 37].
Biosynthesis of serine, glycine and one-carbon metabolism are crucial for maintaining cancer cell survival and proliferation and are of high clinical relevance. Excessive activation of serine and glycine biosynthesis leads to oncogenesis, providing a substrate for one-carbon metabolism. One-carbon metabolism, which is a cyclic metabolic network based on the chemical reaction of folic acid compounds, provides essential proteins, nucleic acids, lipids and other biological macromolecules to support tumor growth (31).
It was found that asparagine can affect cancer cell growth [38], leading to the use of the bacterial enzyme L-asparaginase to limit the availability of asparagine [14, 39]. Significant efforts are being made to develop agents to deplete other amino acids and to act on central metabolic pathways that are dysregulated in cancer cells, including glycolysis, the tricarboxylic acid cycle and lipogenesis. Many of these drugs are still in preclinical stages, but some are currently being evaluated in clinical trials [14].
CONCLUSIONS
This study identified six amino acids whose levels differed significantly in the monocytes of breast cancer patients from those of healthy donors. Significant differences in metabolite levels were found for glycine (p-value = 0.0127), asparagine (p-value = 0.0197), proline (p-value = 0.0159), methionine (p-value = 0.0357), tryptophan (p-value = 0.0028), tyrosine (p-value = 0.0127). Bioinformatic analysis of metabolic pathways involving the discovered amino acids revealed biological networks that could potentially be involved in altering the phenotype of monocytes exposed to BC. The observed changes in the amino acid content of monocytes may indicate the influence of tumor processes not only on the microenvironment, but also indirectly on distant immune system participants. Based on the differences found, a model with 100% specificity and sensitivity has been proposed to diagnose BC according to the amino acid profile of monocytes.